3D Printing Innovations in Bone Tissue Engineering
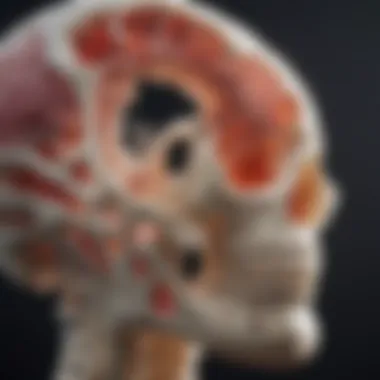
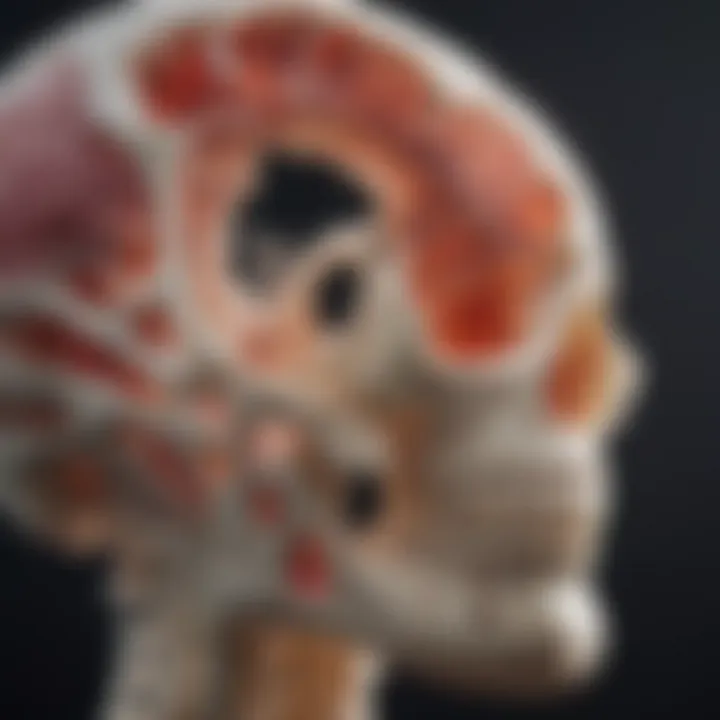
Intro
The world of medicine is ever-evolving, with innovative technologies constantly reshaping how we approach complex problems. One remarkable advancement is the integration of 3D printing in the field of bone tissue engineering. This tech is not just a gimmick or a passing fad; it’s a crucial tool that could redefine surgical practices and enhance regenerative medicine. With a focus on biocompatibility and material innovation, this journey into the realm of 3D-printed bone grafts unlocks new possibilities for patients suffering from skeletal ailments.
As we delve deeper into this exciting intersection of technology and biology, it's essential to grasp the fundamental principles that underpin these advancements. We will explore the intricate processes that allow for the creation of customized bone structures and highlight how these techniques stand to impact patient care and surgical outcomes.
Research Overview
Summary of Key Findings
Numerous studies highlight the potential of 3D printing to produce patient-specific bone grafts that facilitate quicker recovery and better integration with existing biological tissues. One particularly intriguing finding from recent research suggests that materials such as bioactive glass and composite polymers offer enhanced osseointegration, meaning they bond more effectively with bone tissue. This integration is paramount as it minimizes the body’s rejection of foreign materials, a challenge previously faced in traditional grafting techniques.
Background and Context
The rise of 3D printing technology can be traced back to various industries including automotive and aerospace, but its application in medicine, insbesondere in bone tissue engineering, is what draws considerable interest. The traditional methods of grafting often left much to be desired regarding precision and adaptability. Bone defects caused by trauma or disease present unique challenges—things like matching the graft shape, size, and material properties to the individual patient. 3D printing steps in as a game changer, providing tailor-made solutions that not only fit like a glove but also promote better biological responses.
Methodology
Experimental Design
Most research employs a variety of sophisticated methodologies to test the effectiveness of 3D printing in bone healing. These can range from in vitro studies on human osteoblasts to in vivo models with animal subjects. Each stage emphasizes the interaction between the 3D-printed materials and biological systems—crucially studying factors like cell adhesion, proliferation, and differentiation.
Data Collection Techniques
Data collection is a vital part of any research endeavor. Researchers often utilize imaging techniques like micro-computed tomography (CT) to assess the structural integrity of printed grafts over time. Additionally, histological analysis aids in understanding how well the graft integrates with existing bone tissue at a cellular level.
"The potential for 3D-printed bone grafts highlights not only innovation in engineering but also a profound shift in how we think about treating skeletal health issues."
Prelude to 3D Printing in Medicine
The realm of healthcare has been significantly transformed by the advent of 3D printing technology. This innovative approach is not merely a trend; it represents a fundamental shift in medical practices, particularly in areas such as bone tissue engineering. The ability to create customized, precise replicas of biological structures holds immense promise for improving surgical outcomes and patient care.
Historical Context
To understand the current landscape of 3D printing in medicine, it helps to take a step back and reflect on its origins. The concept of additive manufacturing traces its beginnings to the 1980s when Chuck Hull pioneered stereolithography, a technique that allowed for the layer-by-layer construction of objects. Initially, this technology found its footing in industries like aerospace and automotive. However, by the early 2000s, researchers began applying these techniques to the medical field, with the first significant applications seen in prosthetics and dental implants.
Since then, the journey has been marked by rapid advancements. One might say that the technology has matured beyond its teenage years, now boasting increasingly intricate designs and a variety of materials suitable for biological applications. Companies like Organovo and Stratasys have spearheaded research to explore not just the creation of bone scaffolds but also the printing of tissues and organs—although we are still some ways from fully functioning bio-printed organs.
Evolution of Medical Applications
As the technology has matured, so too have its applications in health care. In the realm of bone tissue engineering, for instance, the ability to tailor constructs that mimic natural bone structure is a game changer. Rather than relying on traditional grafts or synthetic materials, surgeons can turn to 3D printed solutions that integrate seamlessly with the patient’s anatomy.
- Customized Implants: Patients no longer receive one-size-fits-all implants. With 3D printing, healthcare providers can create implants tailored to the specific dimensions and curvature of an individual’s bones. This leads to better fit and faster recovery times.
- Complex Geometries: 3D printing allows for intricate designs that are simply unattainable through conventional manufacturing. The porous structures not only support bone growth but also enhance the integration of the implant with surrounding tissues.
- Material Diversity: The field has seen an explosion of bio-compatible materials—from ceramics and polymers to metals—that can be printed and are suited for different medical needs. This versatility enables tailored approaches based on the specific pathology being addressed.
- Patient-Specific Solutions: The use of imaging techniques, like CT scans, to inform the design process ensures that printed constructs align closely with existing anatomy, fostering better surgical results.
As we navigate through the landscape of 3D printing in bone tissue engineering, it's essential to remain aware of the accompanying challenges and ethical considerations. However, the potential benefits are vast, and the advancements made in this field can and will shape the future of medical practices, leading to optimized outcomes and improved patient lives.
Fundamentals of 3D Printing Technology
The fundamentals of 3D printing technology form the bedrock of its application in bone tissue engineering. This technology’s ability to construct complex structures layer by layer is paramount in tailoring solutions to meet the needs of injuries and reconstructive surgeries. Understanding these principles not only helps researchers and practitioners alike appreciate the potential but also addresses important considerations in practical applications.
Specific Elements of 3D Printing Technology
At its core, 3D printing revolves around additive manufacturing processes, which distinguish it from traditional subtractive methods. The additive nature permits greater design freedom. These custom designs can be tailored preoperatively, aligning them closely with patient anatomy. In bone tissue engineering, the capability to replicate intricate bone structures with porous architectures is an absolute game-changer.
Benefits
The emerging technologies in 3D printing come with a host of benefits: they reduce material waste, lowering costs in production and development phases. Furthermore, the shortened lead time from design to physical part means faster prototyping. Finally, the ability to personalize implants establishes a patient-centric approach in medicine, enhancing outcomes and satisfaction levels.
"Personalized medical solutions in the form of 3D-printed bones aren't just a trend; they're a revolution in precision medicine."
Considerations
Despite the advancements and potentials, there are considerations like the regulatory landscape that cannot be overlooked. As the technology matures, ensuring consistent quality and safety of printed materials will be crucial. There's also the issue of education; practitioners must be trained on this tech to harness its full capabilities effectively.
Additive Manufacturing Processes
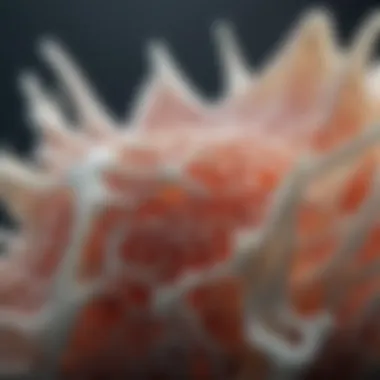
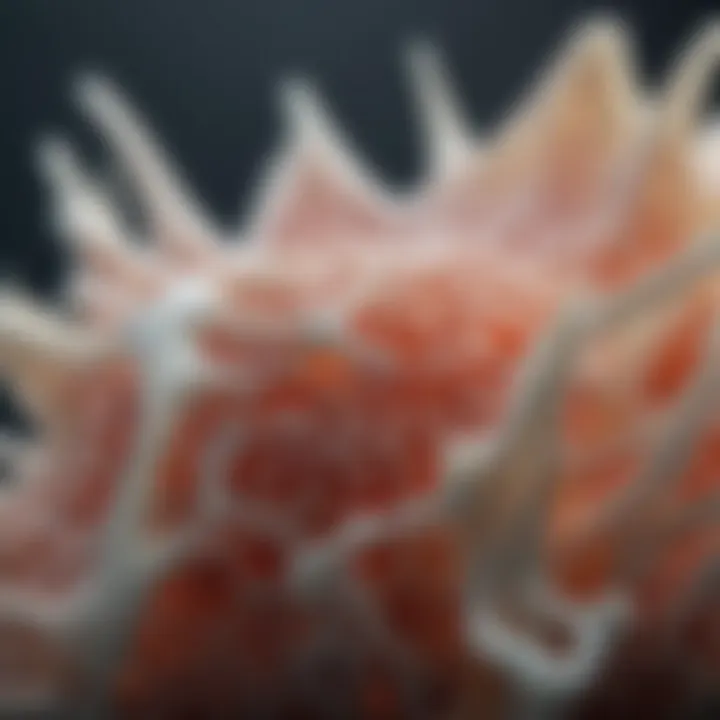
Additive manufacturing processes are the heart of 3D printing. Traditional manufacturing often involved cutting away material to create an object. Contrary to that approach, additive manufacturing builds items by layering materials, which allows for much greater design flexibility and complexity. This is particularly vital for creating bone structures that mimic natural anatomy. The common processes include methods such as Fused Deposition Modeling, Stereolithography, and Selective Laser Sintering, and each brings its specific advantages to bone tissue engineering.
Types of 3D Printing Methods
Each 3D printing technique tackles challenges differently, but they all share the goal of creating functional, biocompatible structures for medical usage. Below are some of the most prevalent methods used in the context of bone tissue engineering:
Fused Deposition Modeling
Fused Deposition Modeling (FDM) stands out by utilizing a thermoplastic filament, which is heated until it melts, and then extruded to form layers. This method is particularly beneficial due to its low cost and simplicity, making it a popular entry point into 3D printing. Its key characteristic is the ability to use a variety of materials, including bio-based plastics that support cellular attachment. One unique feature of FDM is its ease of providing structural reinforcement, suitable for bone-like applications. However, it can suffer from limited resolution, affecting the fine detail necessary for some complex structures.
Stereolithography
Stereolithography (SLA) employs a laser to cure a resin into hardened plastic. This method is notable for its high precision and smooth surface finish. This accuracy makes SLA a favorable choice for creating detailed bone scaffolds. The unique feature of Stereolithography is its ability to produce complex geometries that can closely mimic natural bone structures. However, a downside is the relatively high cost of equipment and materials, which can raise barriers to entry for some institutions.
Selective Laser Sintering
Selective Laser Sintering (SLS) works by using a laser to fuse powdered materials together, resulting in a solid structure. One significant aspect of SLS is its ability to work with a range of materials including metals, polymers, and ceramics. This versatility opens up a pathway for creating biocompatible bone grafts. The key characteristic of SLS is that it does not require support structures, thus allowing for greater freedom in part design. Still, the process can be energy-intensive and costly, which are significant factors when considering large-scale applications in clinical settings.
In summary, the fundamentals of 3D printing technology not only frame our understanding but also reaffirm the potential these methods can unlock in bone tissue engineering. As we delve into the nuances of each method, it becomes clear that while challenges exist, the momentum towards innovation is promising.
Materials Used in 3D Printed Bones
The selection of materials in 3D printing for bone tissue engineering holds paramount importance, serving as the backbone of the entire process. These materials not only influence the mechanical properties and biocompatibility of the resulting structures but also play a critical role in promoting the integration of the printed materials with the surrounding biological tissues. As the demand for customized and effective bone grafts grows, understanding the various materials—biomaterials, customizable properties, and their implications—becomes essential.
Biomaterials for Bone Regeneration
Biomaterials specifically engineered for bone regeneration possess unique characteristics that facilitate their interaction with biological systems. The choice of materials can significantly affect cellular response, scaffolding strength, and overall tissue integration.
Polymeric Materials
Polymeric materials are gaining traction due to their versatility and favorable characteristics. These materials, often characterized by their elasticity, allow for significant freedom in design and customization. One key characteristic of polymeric materials is their ability to be engineered for specific mechanical properties, ensuring they match the natural bone’s flexibility and strength.
A notable feature of polymer materials is their degradation profile. Many can degrade over time, allowing for the gradual transfer of load from the implant to the healing bone tissue. However, this presents both advantages and disadvantages; while the gradual degradation promotes tissue growth, it may not always support the initial mechanical stability required in load-bearing applications.
Ceramic Materials
Ceramic materials also play a critical role in bone tissue engineering. With their inherent bioactive properties, ceramics, such as hydroxyapatite, are often used due to their ability to promote bone growth and integration. One key factor that makes ceramic materials popular is their excellent osteoconductivity, which supports bone cells in adhering to the implant and encourages new bone formation.
The unique feature of ceramics is their ability to mimic the inorganic component of bone, offering high compressive strength. Nonetheless, they exhibit brittleness, which can be a drawback in high-stress environments. Managing this brittleness while ensuring sufficient porosity remains a challenge in their development.
Composite Materials
Composite materials emerge as a robust alternative, combining the strengths of both polymers and ceramics to create a hybrid solution. A significant aspect of composite materials is their enhanced mechanical properties, depending on the formulation. They can be engineered to have a balance between strength and elasticity, making them a choice for applications requiring both durability and flexibility.
The notable feature of composite materials is that they can be customized to tailor specific degradation rates, a critical factor in alignment with biological healing processes. However, the complexity in manufacturing these composites can pose challenges. Each unique combination of materials necessitates meticulous design and optimization to ensure functionality and safety.
Customizable Material Properties
Customizable material properties are foundational to achieving tailored solutions for individual patient needs. In bone tissue engineering, the ability to adjust composition, mechanical strength, and degradation rates enhances the potential for successful outcomes. This customized approach not only facilitates better integration within the biological environment but also allows for adjustments based on the specific clinical context, ultimately improving patient recovery and quality of life.
Design and Production Processes for Bone Printing
The design and production processes in bone printing encompass a vital phase that integrates artistic creativity with advanced engineering and biological insight. These processes are not merely about creating bone-like structures; they are the foundation that influences the success of 3D printed implants. This part of the article aims to delve into critical elements such as the significance of Computer-Aided Design (CAD) and the thorough application of layer-by-layer fabrication methods. Understanding these components enables researchers to harness the full potential of 3D printing, paving the way for breakthroughs in bone tissue engineering.
CAD and 3D Modeling Techniques
Computer-Aided Design plays a significant role in shaping the trajectory of bone printing technology. CAD software facilitates the design of highly intricate models that can replicate the microarchitectural features of natural bone. Design precision is paramount here. The idea is not just to mimic bone shape but to reflect its internal composition and behavior under stress.
Benefits of employing CAD include:
- Customization: Surgeons can create personalized models tailored to individual patient anatomy, thus enhancing fit and function. This can be especially useful in reconstructive surgeries.
- Visualization: Detailed 3D models allow for a clear visualization of the surgical process, making it easier for surgical teams to strategize.
- Iterative Design: CAD systems enable rapid testing and modification, leading to optimized designs that can be produced in shorter time frames.
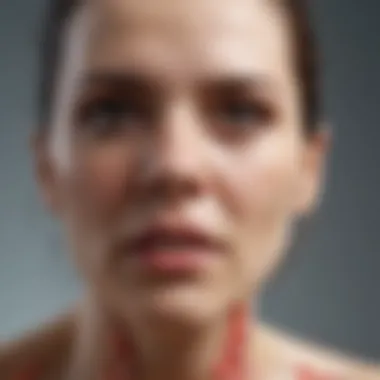
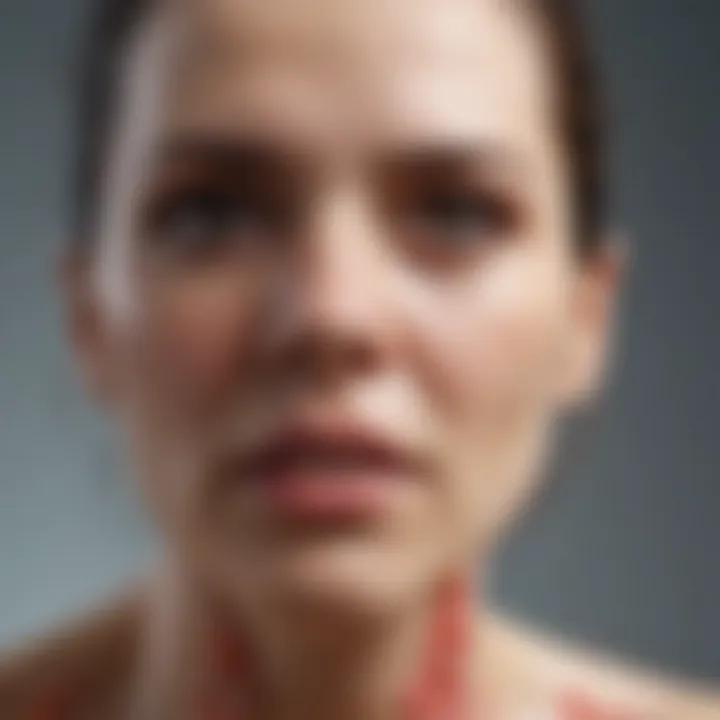
With the skills in CAD, designers can program various parameters like porosity, mechanical strength, and scaffolding layout, which directly influence how well the printed bone integrates with the existing tissue. However, this design phase must be backed by knowledge in biology and material science; unsound designs could result in poor biocompatibility or insufficient mechanical support in their application.
Layer-by-Layer Fabrication Approach
The layer-by-layer fabrication approach is a hallmark of additive manufacturing that underlines the beauty and complexity of 3D printing. Each layer is meticulously laid down, gradually building up a complete structure from the ground up. This method is particularly advantageous when it comes to fabricating bone implants due to its accuracy and ability to recreate complex geometries.
Key aspects of this approach include:
- Detail Precision: Each layer is created with high accuracy, allowing for the replication of intricate details crucial for functional bone grafts.
- Material Efficiency: This method minimizes waste, as material is only used where needed, in contrast to traditional subtractive manufacturing techniques.
- Biomaterial Integration: The layer-by-layer strategy allows for blending different materials within the same print process—say, integrating polymers with ceramics for enhanced biocompatibility and strength.
This technique does not come without challenges. The layering process requires careful control of various conditions such as temperature and material consistency. Additionally, there’s the risk of layer adhesion failure, which could compromise the structural integrity of the final implant.
Overall, the design and production processes for bone printing are crucial to its advancement in the medical field. As the techniques evolve, they draw ever closer to producing implants that not only serve their purpose but also forge a path toward more holistic, integrated healing strategies.
"The integration of sophisticated design tools with innovative manufacturing techniques is pivotal for the next generation of bone tissue engineering."
Stay tuned as we progress through the exploration of biocompatibility and safety considerations in the subsequent section.
Biocompatibility and Safety Considerations
The role of biocompatibility and safety in bone tissue engineering through 3D printing cannot be overstated. As more advanced materials and methods emerge, understanding how these elements interact with the human body becomes critical. Simply put, biocompatibility refers to the ability of a material to perform safely and effectively within the biological environment. When we talk about bone implants or grafts, ensuring that the material does not provoke an adverse reaction in the body is paramount. Safe materials enhance the healing process and align with the body’s natural structure, which is what makes them so valuable in regenerative medicine.
Regulatory Standards
Meeting regulatory standards is a must in this field, especially considering the advancements in 3D printing where custom designs and materials are outlined in the production process. Agencies like the U.S. Food and Drug Administration (FDA) have set forth guidelines that address the manufacturing, testing, and clinical use of medical devices — 3D-printed bones included. These guidelines ensure that every material used has been rigorously tested for
- Toxicity: Ensuring that no harmful substances leach into the surrounding tissues.
- Mechanical Integrity: The printed graft has to withstand forces and stresses present in the skeletal system.
- Sterility: The end product must be sterile to prevent infections during procedures.
Complying with these standards doesn’t just aid in patient safety; it also fosters public trust in advanced medical technologies. When health specialists can assure patients that the materials used are fully tested, it enhances the credibility of the procedures.
"In many cases, regulatory compliance is the difference between successful patient outcomes and serious complications."
Testing for Biocompatibility
Testing plays a significant part in establishing the confidence needed to introduce new materials into clinical settings. To evaluate biocompatibility, a range of in vitro (within a controlled environment outside of a living organism) and in vivo (within a living organism) tests are conducted. These tests help researchers ascertain how the new materials affect living tissues. Common testing parameters include:
- Cytotoxicity Tests: Evaluating whether the material releases any substances that could harm surrounding cells.
- Implantation Studies: Observing the long-term effects when implanted into animal models to see how the body reacts over time.
- Histopathological Analysis: Examining tissue samples to identify any inflammatory responses or foreign body reactions following implantation.
These evaluations are instrumental in ensuring that any new 3D-printed bone implant or graft is not only safe but also effective in promoting healing. Without rigorous testing, it would be like diving into the deep end of the pool without checking the water first—it’s a gamble that can have dire consequences.
Clinical Applications of 3D Printed Bones
The emergence of 3D printing technology in the field of bone tissue engineering is nothing short of a paradigm shift. The application of this innovative technology in clinical settings is rapidly evolving, opening doors to unprecedented solutions for complex orthopedic issues. By creating patient-specific implants, 3D printing not only enhances the accuracy of surgical procedures but also significantly improves patient outcomes.
Case Studies in Bone Repair
Delving into specific examples can shed light on the tangible benefits of 3D printing in medical practice. One noteworthy case involved a 34-year-old patient, previously facing amputation due to a bone tumor in their lower leg. Surgeons utilized advanced 3D scanning technology to map the exact anatomy of the patient’s leg. Using this data, they designed and printed a custom titanium implant that replicated the natural shape and mechanical properties of bone. Following the procedure, the patient demonstrated remarkable recovery, regaining full mobility and returning to daily activities within months. This case underscores how personalized implants facilitate a more natural healing process.
In another instance, a team at a leading research hospital treated a young child with a critical bone defect due to congenital conditions. The surgical team created a biodegradable scaffold through 3D printing, subsequently infused with stem cells harvested from the patient. Over time, this scaffold dissolved as the child's natural bone grew and integrated into the implant, showcasing the potential of 3D printing in regenerating bone rather than merely replacing it. This approach could minimize the need for future surgeries, presenting a sustainable solution in pediatrics.
Applications in Complex Surgeries
Beyond straightforward repairs, 3D printing has made significant inroads into more complicated surgical scenarios. One specific application can be found in craniofacial surgery. Surgeons utilize 3D printed models of a patient’s cranium to preoperatively plan a procedure aimed at correcting deformities or trauma-related injuries. By having a scaled model, the surgical team gains invaluable insights, resulting in precise incisions and tailored approaches during actual surgery.
Moreover, in orthopedic trauma cases, where multiple broken bones may complicate repair, 3D printed fixation devices allow for tailored support that aligns perfectly with a patient’s unique anatomy. These custom plates and screws not only provide superior mechanical support but also enhance the alignment of bone healing, which can decrease recovery time and potential complications.
Another area of progress is in joint replacements. For instance, patients who require knee resurfacing may benefit from custom 3D printed implants designed for an exact fit, reducing the risk of implant failure and the necessity of revision surgeries later on. The take-away here is that in complex surgical applications, 3D printing offers surgeons a powerful tool to navigate challenges with unprecedented precision.
"The true essence of 3D printing in medicine is about making solutions fit the patient, rather than making patients fit existing solutions."
In summary, the real-world applications of 3D printed bones extend far beyond mere novelty. They represent a commitment to innovation in patient care—evolving surgical practice, enhancing recovery, and ultimately shaping the future of orthopedic treatment.
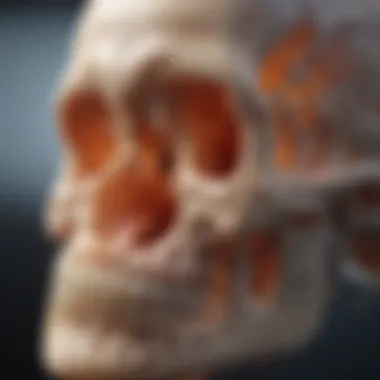
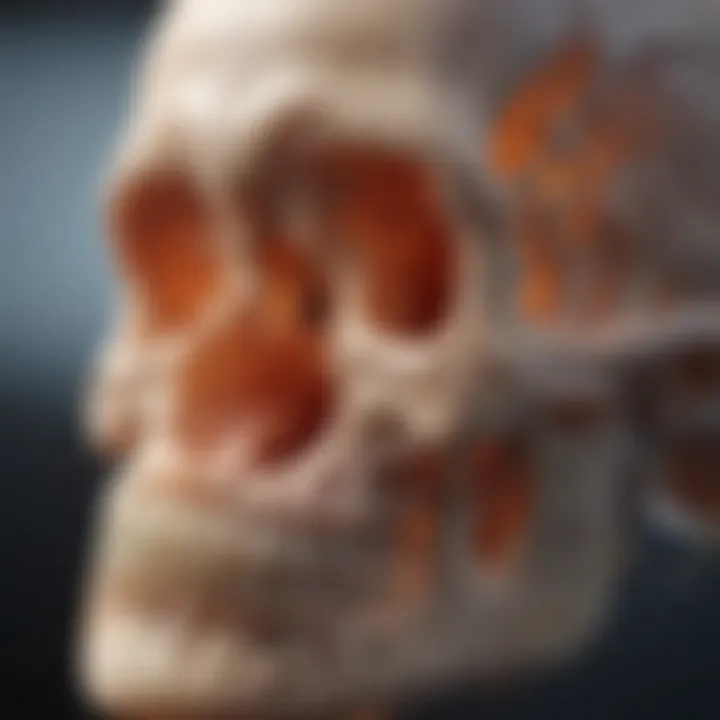
Challenges in 3D Printing Bone
The advancement of 3D printing technology in bone tissue engineering has brought along numerous opportunities for innovation, yet it’s not without its set of challenges. Understanding these challenges is crucial because overcoming them is essential for maximizing the benefits and efficiency of 3D printed bone grafts. Not only does this impact the quality of the printed materials, it also influences the acceptance of these technologies in clinical settings. Navigating these obstacles can inform researchers and practitioners, enabling improvements in both methodologies and applications.
Technical Limitations
One of the most pronounced hurdles in this field is the technical limitations of the printing technology itself. Despite progress, several issues remain for producing bone scaffolds that can mimic the complex architecture of natural bone. To elaborate, consider the following aspects:
- Resolution and Detail Accuracy: Achieving high resolution is critical for replicating the microstructure of bone tissue. Current 3D printers might struggle with this, leading to scaffolds with features that are either too large or imprecise.
- Material Properties: The mechanical strength of 3D printed bones often doesn’t meet the requirements of natural bone. For instance, some materials used can be too brittle or fail to support load-bearing applications.
- Cellular Compatibility: While creating scaffolds, ensuring that they are conducive to cell attachment and proliferation is essential. However, some materials can impair cell function, leading to unsuccessful integration with the host tissue.
These technical limitations pose significant barriers to the full utilization of 3D printing in bone repair and regeneration. Researchers are continuously seeking ways to refine these technologies, but noticeable gaps remain, indicating that further advances are necessary to advance the practical utility of 3D printed bone.
"Addressing technical limitations is foundational for the future of bone tissue engineering. Failure to do so can hinder the realization of these technologies in real clinical settings."
Ethical Considerations
The use of 3D printing in medicine brings about complex ethical considerations that can't be ignored. As the landscape shifts, the implications of creating biological structures raise several questions:
- Equity in Access: Who gets to benefit from these advanced technologies? Ensuring equitable access to 3D printed solutions in different socioeconomic environments is vital. If these innovations remain available only to certain populations, it can exacerbate existing healthcare disparities.
- Long-Term Implications: The long-term effects of artificial constructs in human bodies are still not fully understood. There’s a potential for unforeseen complications that could arise from their integration. Decisions made without thorough understanding could lead to ethical dilemmas, especially in vulnerable patient populations.
- Informed Consent: Patients must be well informed about what undergoing 3D printed bone graft procedures entail. This includes potential risks and benefits, ensuring they can make educated choices about their treatment.
In summary, tackling these ethical considerations is just as critical as overcoming the technical limitations. As the field evolves, fostering an ethos of responsible innovation will guide the practice and ensure the technology serves humanity in its entirety.
Future Directions in Bone 3D Printing
The horizon for bone 3D printing is bursting with potential. As this technology matures, the integration of novel innovations today will shape the surgical landscape for tomorrow. By tapping into advancements in materials science, computational techniques, and bioprinting, we open a world of possibilities that can enhance surgical efficiency and patient outcomes. It’s not just about creating structures that mimic bone; it’s about crafting intricate systems that actively promote healing and reduce recovery times. The following sections will delve into exciting developments and the synergy of 3D printing with regenerative medicine.
Innovations on the Horizon
Emerging trends in 3D printing technology promise not only to improve the accuracy of bone grafts but also redefine the capabilities of the materials used.
- Smart Biomaterials: The introduction of smart biomaterials that respond to biological stimuli opens new doors. These materials can adjust their properties based on the surrounding environment, promoting enhanced healing processes.
- 3D Bioprinting: Integrating living cells with scaffolding materials represents another leap forward. Using 3D bioprinting techniques, scientists can fabricate composite structures that contain viable cells, which may lead toward functional bone tissue that integrates seamlessly with the body.
- Personalized Implants: Customizing implants based on patient-specific anatomical data can significantly improve the success rates of surgeries. By utilizing advanced imaging techniques such as MRI or CT scans, surgeons can design bespoke implants tailored to the exact shape and density of the patient's bone.
- Composite Materials: There is a rising trend in using composite materials that combine different substances to capitalize on the strengths of each. For instance, mixing bioceramics with polymers not only provides mechanical strength but also offers biological compatibility, leading to a more effective integration into the body.
"The future of bone tissue engineering is not just about replication; it's about restoration and regeneration in a way that the body can trust again."
Integration with Regenerative Medicine
The synergy between 3D printing and regenerative medicine could very well define the next leap in surgical practice.
- Stem Cell Applications: Innovations involving using stem cells in conjunction with 3D printed scaffolds can facilitate tissue regeneration in ways we've only begun to understand. As stem cell research advances, its incorporation into printed structures can potentially enhance the body’s natural healing capabilities.
- Tissue Engineering Scaffolds: Creating scaffolds that not only provide structural support but also promote cell growth and tissue repair is a significant focus area. These scaffolds can be designed to degrade over time, allowing for the natural building of tissue while the scaffold is absorbed, ensuring that regeneration occurs smoothly.
- Biodegradable Materials: The advancement of biodegradable materials allows for scaffolds that can slowly dissolve and be replaced by new bone, minimizing complications and maximizing integration. Preparing these materials will be crucial as it influences the success of implant integration during healing processes.
- Regulatory Pathways: As these technologies advance, establishing clear regulatory pathways will be vital. Collaboration among manufacturers, regulatory bodies, and medical professionals can foster an environment where innovations can be introduced safely, ensuring that new solutions reach the patients who need them effectively.
In summary, the blending of 3D printing with regenerative medicine is set to reshape how we approach bone repair. With innovations continually surfacing, fostering an interdisciplinary conversation can facilitate breakthroughs that will enhance patient care and redefine recovery outcomes.
Closure
The integration of 3D printing into bone tissue engineering stands as a marker of innovation that carries the potential to reshape healthcare practices. Throughout this article, we’ve explored various dimensions of this technology, showcasing how its application can lead to more effective treatments for bone-related injuries and conditions. The significance lies not just in the technology itself but also in how it addresses numerous challenges faced in traditional methods.
An essential takeaway is the vast array of materials now available for use in this sector. With options ranging from biodegradable polymers to bioactive ceramics, the selection allows for personalization of grafts to meet specific needs of individual patients. Also, the customizable nature of 3D printing means that shapes and structures of grafts can be tailored precisely, aligning closely with the human anatomy. This fine-tuned approach can substantially boost recovery times and enhance overall success rates.
Yet, as we look towards the future, we must remain cognizant of obstacles like regulatory hurdles and technical limitations. The balance between innovation and safety cannot be overstated. Adherence to stringent regulatory standards and continuous testing for biocompatibility ensures that advancements do not come at the cost of patient safety.
Additionally, we cannot ignore the ethical considerations surrounding the technology. Questions concerning equitable access, particularly for underprivileged populations, remain relevant. The dialogue about these concerns must evolve alongside technological advancements to ensure that progress benefits all.
In summary, while the journey of 3D printing in bone tissue engineering is still unfolding, the implications of its advancement signal hope. By continuing to refine methodologies and promoting interdisciplinary collaborations, the horizon seems promising for not just patients, but for the future of medicine as a whole.
Summary of Key Insights
The discourse around 3D printing in bone tissue engineering has unveiled a number of pivotal insights:
- Personalization: Tailored bone grafts are leading to improved surgical outcomes and faster healing times.
- Material Diversity: The growing library of biocompatible materials provides options that adapt to specific surgical needs.
- Technical Challenges: Awareness of the technical limitations can drive continued improvement and innovation in this field.
- Regulatory Frameworks: Emphasizing the need for strict adherence to safety standards ensures patient protection while encouraging exploration.
- Ethical Conversations: Addressing equity in access is as crucial as the technology itself.
Reflections on the Future Impact
Looking ahead, the potential for 3D printing technology in bone tissue engineering appears vast. As research delves deeper into hybrid materials and stem cell integration, the possibilities for regenerative medicine expand exponentially. Advancements in printing resolution and speed will likely enhance the accessibility of this technology in clinical settings.
Moreover, the cross-pollination of disciplines—from engineering to biology—promises richer innovations. Educational institutions and research bodies can greatly benefit from collaboration, creating a pipeline of new talent aimed at tackling emerging challenges.
Ultimately, the future impact rests not just in technological prowess, but in harmonizing that prowess with ethical considerations and regulatory frameworks. The ongoing discourse surrounding 3D printing in medicine will, hopefully, guide these innovations towards accessible and life-saving treatments for all.