Adenosine Triphosphate: The Energy Currency of Life
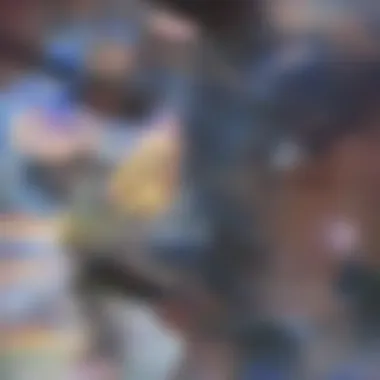
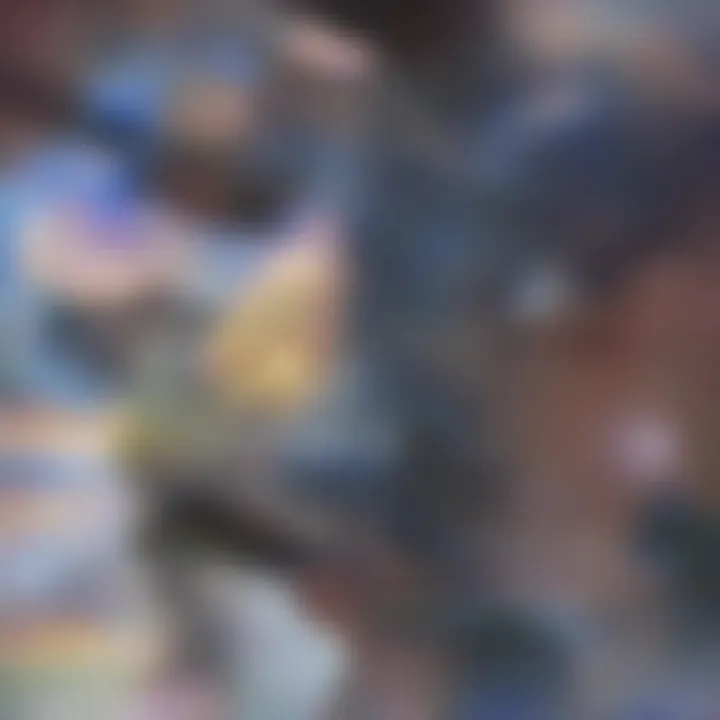
Intro
Adenosine triphosphate, commonly known as ATP, is often referred to as the energy currency of life due to its central role in cellular processes. ATP is crucial for powering biochemical reactions that sustain life. This molecule is responsible for providing the energy required for various cellular functions including muscle contraction, nerve impulse propagation, and biochemical synthesis.
In this exploration, we will delve into the intricate structure and multifaceted functions of ATP. Additionally, we will examine its synthesis and degradation processes, alongside its essential role in metabolic pathways. Understanding ATP is vital for grasping the complexities of cellular dynamics and energy transfer within biological systems.
Understanding Adenosine Triphosphate
Adenosine triphosphate, commonly known as ATP, is often referred to as the energy currency of life. The understanding of ATP transcends mere biochemistry; it encapsulates the very essence of energy transfer in living organisms. ATP is pivotal in various biological processes, including metabolism, muscle contraction, and cellular signaling. It is essential for life, powering everything from basic cellular functions to complex physiological responses. Understanding ATP enhances comprehension of how organisms utilize energy and maintain homeostasis.
ATP functions effectively through its structure and chemical properties, which are crucial for its role in energy transfer. This comprehension aids in exploring various biochemical pathways and understanding metabolic disorders. Furthermore, recognizing the function of ATP allows students and researchers to appreciate its significance in health and disease management. In this article, we delve deep into the specifics of ATP, considering its fundamental aspects and its broader biological implications.
Basic Structure of ATP
Adenosine triphosphate consists of three primary components: an adenine base, a ribose sugar, and three phosphate groups connected by high-energy bonds. The structure is significant because the bonds between these phosphate groups are where the energy is stored and released during chemical reactions. When a phosphate group is removed—through a process known as hydrolysis—energy is released, which is then available for cellular work. This mechanism is fundamental for ATP's role as an energy currency.
Additionally, the specific arrangement of these components is essential for the molecule's function. The configuration of ATP allows for easy interaction with various enzymes and proteins, facilitating numerous biochemical reactions needed for cellular metabolism. Thus, a deeper understanding of its structure also provides insight into how ATP functions within different cellular environments.
Chemical Properties of ATP
The chemical properties of ATP are central to its function as a biochemical energy transfer molecule. ATP is highly soluble in water, which enables it to efficiently transport energy within cells. The presence of multiple negative charges in the phosphate groups contributes to its high-energy state. When these groups break off during hydrolysis, they release energy that cells can utilize for various tasks, ranging from muscle contraction to active transport across membranes.
Moreover, ATP’s stability under physiological conditions is relevant for its role in metabolism. The molecule is inherently unstable and preferentially breaks down to adenosine diphosphate (ADP) and inorganic phosphate (Pi) to release energy, which shows its readiness to participate in metabolic activities. Understanding these properties also sheds light on how ATP interacts within metabolic pathways and how its levels are regulated in various physiological conditions.
"ATP not only fuels molecular machines that are vital for life but also participates in a complex network of regulation that sustains cellular functions."
Synthesis of Adenosine Triphosphate
The synthesis of adenosine triphosphate (ATP) is a centerpiece in our understanding of cellular energy dynamics. Its creation is essential for various cellular functions, ranging from metabolic processes to muscle contraction. ATP is not just an energy currency; it is fundamental to sustaining life. By exploring its synthesis, we gain insights into how cells convert nutrients into usable energy, outlining the pathways and mechanisms involved.
ATP Formation in Cellular Respiration
Cellular respiration is a primary method by which ATP is produced. The process occurs in the mitochondria, commonly referred to as the powerhouses of the cell. There are three main stages of cellular respiration: glycolysis, the citric acid cycle, and oxidative phosphorylation. Each of these stages contributes to the overall production of ATP.
- Glycolysis: This is the first step that occurs in the cytoplasm. Here, one glucose molecule is broken down into two molecules of pyruvate, generating a net gain of two ATP molecules and two NADH molecules, which will play a role in later stages.
- Citric Acid Cycle: After glycolysis, the pyruvate enters the mitochondria. Through a series of reactions, it is converted to acetyl-CoA and enters the citric acid cycle, also called the Krebs cycle. Each turn of this cycle produces ATP directly and generates electron carriers, NADH and FAD.
- Oxidative Phosphorylation: The electron transport chain receives electrons from NADH and FAD, releasing energy used to pump protons across the mitochondrial membrane. This setup creates a proton gradient, which drives ATP synthesis as protons flow back through ATP synthase, producing ATP.
Role of Glycolysis in ATP Production
Glycolysis holds significant importance as the initial pathway for ATP production. It does not require oxygen, making it an anaerobic process. Glycolysis serves as a rapid means to generate ATP, especially under conditions where oxygen is limited. The net gain of two ATPs from glycolysis means that this process can be crucial in muscle cells during intense exercise, where energy demand is high and oxygen may not be adequately supplied.
Electron Transport Chain and ATP Generation
The electron transport chain is known for its efficiency in ATP generation, yielding the majority of ATP during cellular respiration. Located in the inner mitochondrial membrane, this series of protein complexes facilitates the flow of electrons derived from NADH and FAD. Each electron transfer releases energy, which is harnessed to pump protons into the intermembrane space, creating an electrochemical gradient. The return of protons through ATP synthase generates ATP in a process known as chemiosmosis. This process emphasizes the efficiency of ATP generation in aerobic conditions, making it indispensable for energy-demanding cells.
Alternative ATP Synthesis Pathways
Although cellular respiration is the dominant method for ATP synthesis, there are alternative pathways that can also contribute to ATP production. These pathways include:
- Fermentation: Anaerobic conditions lead to fermentation, which allows glycolysis to continue by regenerating NAD+. In muscle cells, this process produces lactic acid, while yeast performs alcoholic fermentation.
- Substrate-Level Phosphorylation: This occurs when a phosphate group is directly transferred from a substrate to ADP to form ATP, not dependent on the electron transport chain. It is less efficient than oxidative phosphorylation but is vital during high-energy demand periods.
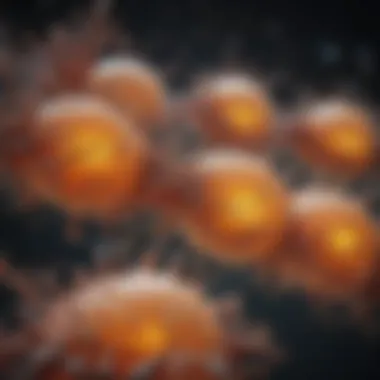
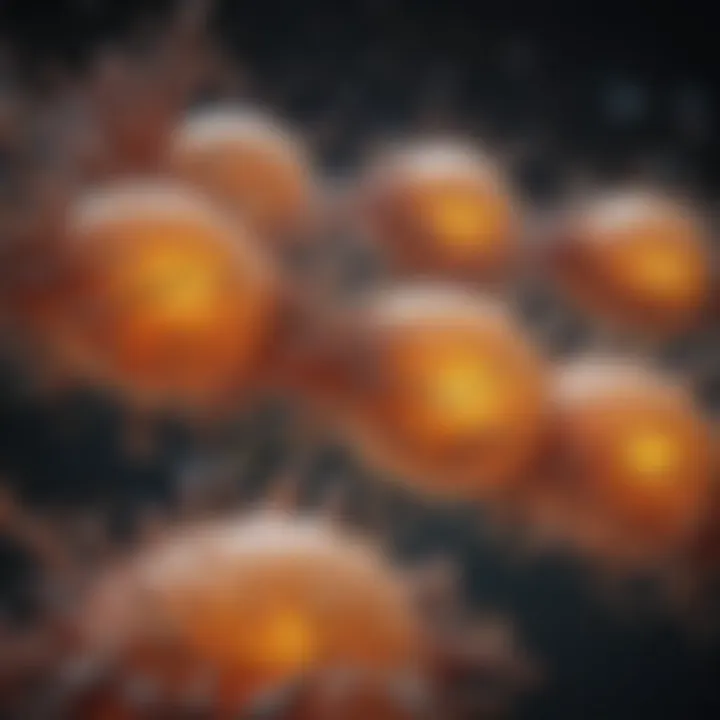
Degradation of Adenosine Triphosphate
The degradation of adenosine triphosphate (ATP) is a vital process in cellular biochemistry. Understanding how ATP is broken down helps elucidate its role in energy metabolism. ATP does not merely serve as a source of energy; its breakdown is an essential part of maintaining cellular functions and regulating metabolic pathways. When cells require energy, they initiate the degradation of ATP, making the process crucial for sustaining life.
ATP Hydrolysis
ATP hydrolysis is the first step in ATP degradation. This chemical reaction occurs when water molecules interact with ATP, resulting in the release of energy. The breaking of the high-energy phosphate bonds releases energy that the cell can harness for various biological activities.
The reaction can be summarized as follows:
[ extATP + extH_2 extO \rightarrow extADP + extP_i + extEnergy ]
Here, adenosine diphosphate (ADP) and inorganic phosphate (P(_i)) are formed alongside energy.
The rate of ATP hydrolysis is influenced by numerous factors, including enzyme activity, concentration of ATP, and availability of substrates. Enzymes such as ATPases play a significant role in accelerating this process, making energy readily available when needed.
Products of ATP Breakdown
The primary products of ATP breakdown are ADP and inorganic phosphate. However, cellular metabolism often leads to the production of other molecules as well. The degradation process results in the following:
- Adenosine Diphosphate (ADP): Formed when ATP loses a phosphate group, ADP can be further converted back to ATP through cellular respiration.
- Inorganic Phosphate (P(_i)): This molecule can be used in various biochemical pathways, including the synthesis of nucleotides and energy storage compounds.
- Adenosine: When ADP undergoes further hydrolysis, adenosine may be produced.
These breakdown products play their roles in various metabolic pathways and cell signaling processes. The conversion of ADP back to ATP through phosphorylation is vital for cellular energy cycles.
Regulatory Mechanisms of ATP Utilization
Cellular regulation of ATP utilization is complex and tightly controlled. Enzymes that catalyze ATP hydrolysis are regulated by substrate availability and allosteric effects. Additional factors affecting ATP utilization include:
- Energy Charge: The energy status of a cell, often termed energy charge, determines how much ATP is utilized. As energy demand increases, ATP hydrolysis accelerates.
- Allosteric Modulation: Enzymes involved in energy metabolism are modulated by the concentration of ATP and its degradation products. High levels of ADP or AMP enhance ATP regeneration pathways.
- Hormonal Signals: Hormones signal metabolic pathways to either increase or decrease ATP production based on current energy demands.
Understanding these regulatory mechanisms is significant for countless biological processes. By comprehending how ATP degradation and utilization are regulated, we can better appreciate its fundamental role in cellular function and energy homeostasis.
The balance between ATP production and degradation is crucial for maintaining cellular energy levels.
Functional Role of Adenosine Triphosphate
Adenosine Triphosphate (ATP) is not merely a chemical entity; it serves as a central player in numerous biological processes. Understanding its functional roles provides insight into why it is often referred to as the energy currency of life. ATP is vital in facilitating energy transfer in cellular activities that support life. Its involvement extends from metabolism to movement, and understanding these connections is fundamental for students and professionals alike.
ATP in Metabolic Pathways
ATP is crucial in metabolic pathways, acting as the primary energy intermediary in the body. During metabolism, ATP is generated from the breakdown of carbohydrates, fats, and proteins. This process occurs through various metabolic pathways, such as glycolysis and the citric acid cycle. Each time ATP is produced, it enables cells to perform essential functions.
For example, ATP helps synthesize macromolecules like proteins and nucleic acids. It also fuels biosynthetic pathways. In these pathways, ATP drives chemical reactions forward, contributing to homeostasis and cellular growth.
- Glycolysis: ATP is both produced and consumed during glycolysis, the first step of cellular respiration.
- Krebs Cycle: In the citric acid cycle, ATP production is maximized as energy carriers are generated for further use in the electron transport chain.
Thus, ATP is intricately involved in transforming nutrients into usable energy, illustrating its indispensable role in metabolism.
ATP's Role in Muscle Contraction
Another important function of ATP lies in muscle contraction. Muscle cells rely on ATP to fuel the actin and myosin filaments, which are essential for muscle movement. When a nerve impulse stimulates a muscle fiber, ATP binds to myosin, allowing it to interact with actin fibers. This interaction leads to the sliding mechanism that causes muscle contraction.
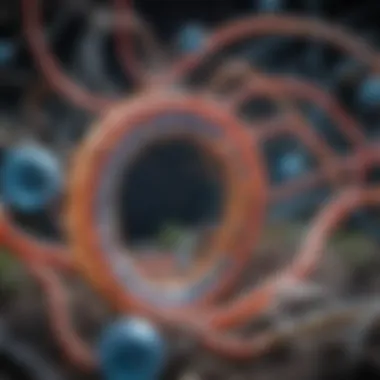
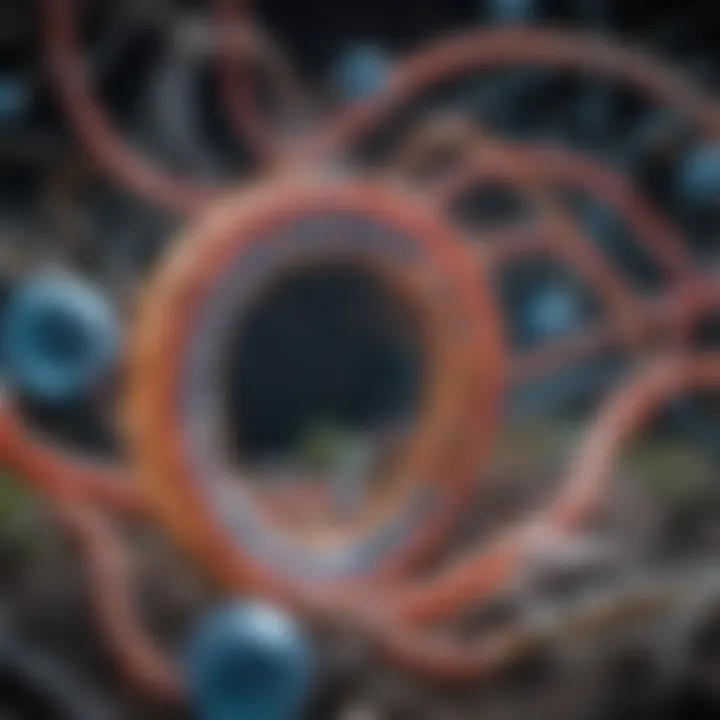
After ATP is hydrolyzed to ADP (adenosine diphosphate) and inorganic phosphate, the energy released is what allows for continued muscle contractions. The cycle repeats as ATP is regenerated during the recovery phase.
This process is especially critical during intense physical activity, where muscle fatigue can occur if ATP levels deplete. The maintenance of ATP levels is, therefore, a crucial aspect for athletes and individuals engaged in regular physical training, highlighting how ATP is a key booster in muscular endurance.
ATP and Signal Transduction
ATP also plays a significant role in signal transduction, which is vital for cellular communication. Cells use ATP as a signaling molecule to regulate various processes within the cell and to communicate with neighboring cells. This includes the activation of protein kinases, which are enzymes that modify other proteins by chemically adding phosphate groups (phosphorylation).
The phosphorylation process affects many cellular functions, including cellular growth, differentiation, and apoptosis (programmed cell death).
“ATP serves not only as the energy source but also as a ligand in several signaling pathways, showcasing its versatility.”
In addition to direct signaling mechanisms, ATP is involved in purinergic signaling, where it binds to purinergic receptors on the cellular surface. This type of signaling affects a variety of physiological processes, including neurotransmission and immune responses.
In summary, ATP functions in metabolic pathways, muscle contractions, and cellular signaling. These roles highlight its significance as the energy currency of life, illuminating how ATP underpins various biological activities essential for maintaining life.
Adenosine Triphosphate Beyond Energy Metabolism
The significance of adenosine triphosphate (ATP) extends well beyond its primary role in energy metabolism. ATP is not merely an energy carrier; it is also integral to various physiological processes within the cell. This section delves into the multifaceted roles of ATP, focusing on its involvement in cellular signaling and neurotransmission.
ATP in Cellular Signaling
ATP plays a crucial role in cellular signaling, acting as a signaling molecule beyond its function as an energy source. It is involved in transmitting signals through extracellular pathways, influencing cellular communication in significant ways. When cells release ATP, it interacts with purinergic receptors on the surface of other cells. This interaction can trigger a cascade of intracellular events that lead to various cellular responses.
Some key points regarding ATP's role in cellular signaling include:
- ATP can act as a primary messenger, initiating cellular responses to different stimuli.
- It helps modulate the activity of ion channels, influencing cellular excitability and various cellular functions.
- ATP signaling is critical in diverse physiological processes, such as immune responses and tissue repair.
Understanding ATP's signaling role illuminates how cells coordinate complex responses to environmental changes. Through this signaling mechanism, ATP bridges the gap between energy metabolism and cellular function, emphasizing its pivotal role in maintaining homeostasis.
Role of ATP in Neurotransmission
ATP is fundamentally essential in neurotransmission, impacting how neurons communicate. In the synaptic cleft, ATP serves as a co-transmitter alongside classical neurotransmitters like dopamine and serotonin. This co-transmission allows for more complex signaling processes within the nervous system.
Key functions of ATP in neurotransmission include:
- Modulation of Neurotransmitter Release: ATP can enhance or inhibit the release of neurotransmitters, affecting synaptic strength and plasticity.
- Regulation of Synaptic Activity: By binding to purinergic receptors on postsynaptic neurons, ATP alters excitability and neurotransmission efficiency.
- Involvement in Pain Pathways: ATP is released during tissue damage or inflammation, signaling to pain pathways and contributing to nociception.
The dual role of ATP in both energy transfer and neurotransmission showcases its versatility and importance. Its ability to affect communication between neurons illustrates its critical role in the nervous system's overall functionality.
ATP is not only the energy currency of life but also a vital signaling molecule that mediates various physiological functions.
Adenosine Triphosphate in Health and Disease
Adenosine triphosphate (ATP) plays a pivotal role in health and disease, acting as the energy currency that sustains cellular processes. Understanding ATP can provide insights into various conditions, especially those related to energy metabolism. This section will detail how ATP is involved in metabolic disorders and its relevance in cancer metabolism. Recognizing these links helps in developing therapeutic strategies and improving medical responses to these challenges.
Energy Metabolism Disorders
Energy metabolism disorders often stem from impaired ATP production or usage. These disorders can affect many organs and systems, leading to a variety of symptoms depending on the severity and the specific tissues involved. Common examples include mitochondrial diseases, where the energy-producing components of cells are dysfunctional.
The key elements to consider in this context involve the pathways of ATP synthesis. Mitochondrial dysfunction can lead to diminished ATP availability. This lack can result in muscle weakness, fatigue, and other systemic issues. Targeting ATP replenishment has therapeutic promise.
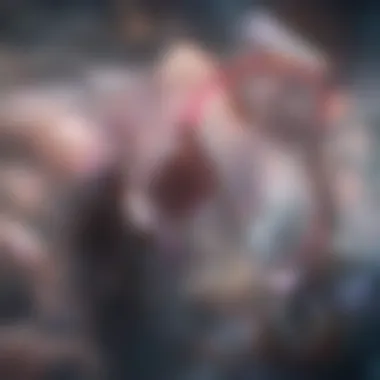

Here are some important aspects of energy metabolism disorders:
- Genetic Factors: Many energy metabolism disorders have a hereditary component, with mutations affecting ATP synthesis.
- Mitochondrial Function: The performance of mitochondria is directly linked to ATP production. Problems in these organelles can result in serious health implications.
- Symptoms and Diagnosis: Fatigue, muscle cramping, and neurological symptoms can often signal these disorders. Proper diagnosis involves testing ATP levels and the efficiency of metabolic pathways.
Understanding these matters is critical for researchers to devise new treatment plans and therapies aimed at restoring energy balance in affected individuals.
ATP and Cancer Metabolism
Cancer cells exhibit altered metabolism, predominantly relying on glycolysis for ATP production, even in the presence of adequate oxygen. This phenomenon, known as the Warburg effect, showcases the distinctive behavior of cancer cells when it comes to ATP generation.
Cancer cells prioritize rapid growth and proliferation, requiring constant energy supply. Here are several points of consideration regarding ATP's role in cancer:
- Increased Demand: Tumors often have higher ATP needs compared to normal tissues due to uncontrolled cell division.
- Target for Therapy: Inhibiting ATP production in cancer cells is being explored as a therapeutic strategy. Drugs like 2-deoxyglucose (2-DG) aim to disrupt glycolysis in tumors, thereby limiting their energy supply.
- Metabolic Flexibility: Cancer cells can switch between metabolic pathways to adapt to different environments. Understanding this flexibility can yield insights into overcoming drug resistance.
"Targeting cancer metabolism by affecting ATP production may yield significant therapeutic advances in oncology."
Addressing the relationship between ATP and cancer provides a foundation for novel treatment modalities. By prioritizing ATP's roles, researchers can enhance the understanding and management of various diseases linked to energy metabolism. Further studies are essential to explore how maximizing ATP efficiency could improve health outcomes.
Research Advances Related to Adenosine Triphosphate
Research into adenosine triphosphate (ATP) has continually expanded over the past few decades. This exploration is not only crucial for understanding fundamental biochemical processes but also has implications in medical research and therapeutic innovations. There are several specific elements that underscore the importance of advancements in ATP research:
- Enhanced Understanding of Cellular Dynamics: Ongoing studies uncover how ATP influences various cellular functions, such as signaling pathways and metabolic processes. This knowledge is vital for developing treatments for metabolic disorders.
- Potential Therapeutic Targets: ATP plays a role in multiple diseases, including cancer and neurodegenerative disorders. Research efforts focus on how to manipulate ATP levels or its pathways. By targeting ATP-related processes, therapies can be more precisely developed.
- Contribution to Biotechnology: Advances in the study of ATP may lead to innovations in biotechnology, including bioengineering and synthetic biology. The ability to understand and modify ATP functions could improve production methods in various industries.
"Research on ATP not only deepens our knowledge of cellular processes but also opens new avenues for medical therapies."
Recent Studies on ATP Functions
Recent investigations into ATP functions have shed light on its complexity. Studies focus on how ATP acts beyond simple energy currency. For instance, researchers examine specific roles of ATP in signaling processes. Biochemical signaling pathways are often regulated directly by ATP concentrations. Understanding these relationships has led to breakthroughs in pharmacology. For example, studies on the ATP-binding cassette transporters have provided insights into drug transport and resistance mechanisms in cancer cells.
Additionally, researchers have explored ATP's role in neurobiology. Studies reveal that ATP acts as a neurotransmitter in some contexts, influencing synaptic transmission. This has implications for understanding disorders like Alzheimer’s disease.
Technological Innovations in ATP Research
Technological advancements have significantly accelerated ATP research. Various innovative methods have been developed to study ATP synthesis and degradation in real-time. For example, luminescence-based assays allow scientists to measure ATP levels accurately in biological samples. This has increased the ability to conduct high-throughput screens for potential drugs.
Moreover, the application of CRISPR technology in ATP research offers precise gene-editing capabilities. This allows for the investigation of genes involved in ATP metabolism and regulation. Notably, techniques such as mass spectrometry are being employed to study ATP’s interactions in metabolic pathways with unprecedented detail.
End
The conclusion of this article emphasizes the critical importance of adenosine triphosphate (ATP) in various biological systems. ATP serves not only as an energy carrier but also as a signaling molecule, affecting numerous biochemical pathways. Understanding ATP's multifaceted role offers significant insights into cellular functions, which is beneficial for students, researchers, and professionals engaged in biological and medical fields. The integration of recent research findings underlines ATP's relevance in health and disease, making it a focal point in biochemistry, molecular biology, and pharmacology.
Furthermore, recognizing the implications of ATP in conditions such as cancer metabolism or energy metabolism disorders enforces the necessity for continued research in this area. As our understanding grows, so does the potential to develop targeted therapies that manipulate ATP pathways. ATP's accessibility and its central role in life processes open up pathways to advancements in medical treatments.
Summary of ATP's Role in Biological Systems
Adenosine triphosphate plays a pivotal role as the energy currency within cells. It is involved in a plethora of metabolic processes, driving reactions that enable growth, repair, and overall homeostasis in living organisms. ATP's structure, containing three phosphate groups, allows it to release and transfer energy efficiently when hydrolyzed. This energy release is essential for cellular activities such as muscle contraction, biosynthesis, and active transport.
In addition, ATP participates in cellular signaling mechanisms. It acts as a substrate for kinases, enzymes that modify proteins through phosphorylation, impacting functionality and activity. The interaction of ATP with various receptors also exemplifies its role in signal transduction. The cumulative effects emphasize the comprehensive involvement of ATP in sustaining life.
Future Directions in ATP Research
Research focused on ATP continues to expand, unveiling novel aspects of its function and applications. Future directions may explore the intricate molecular mechanisms by which ATP influences cellular signaling pathways and its role in cellular communication. Understanding how ATP dynamics are altered in different pathological states can lead to breakthroughs in therapeutic interventions.
Moreover, the exploration of ATP analogs and inhibitors provides opportunities for the development of new drugs that target energy metabolism processes. There is a promising potential in the field of bioenergetics, validating ATP's role in metabolic reprogramming in diseases like cancer.
In summary, the exploration of ATP in research is not just a scientific endeavor; it holds the potential for significant impacts on health and treatment methodologies in the future. As knowledge around ATP continues to advance, so too will our ability to manipulate its functions for various interdisciplinary applications.