Advancements in 3D Brain Imaging Technologies
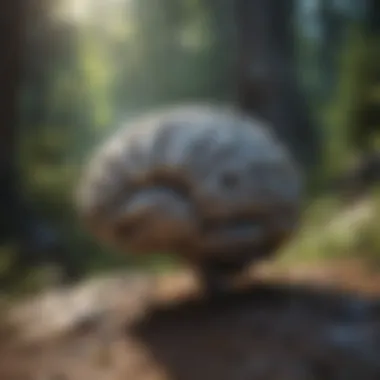
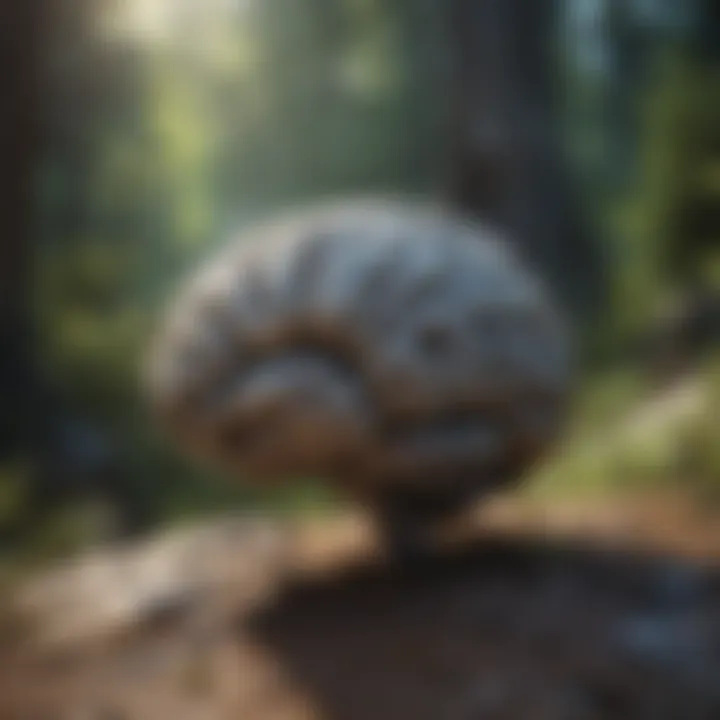
Intro
The field of 3D brain imaging has gained remarkable traction in recent years. Emerging technologies are enhancing our ability to visualize the brain in ways previously thought impossible. Innovations in imaging modalities have transformed both research and clinical practices. This article seeks to provide a comprehensive overview of these advancements.
Understanding the brain's intricate structure and functions is essential for both neuroscience research and medical interventions. Consequently, this article will reflect on the key technologies that are shaping 3D brain imaging, their significant applications in various branches of neuroscience, and the ethical considerations tied to their use.
By addressing these components, readers will have a solid foundation for appreciating the evolving landscape of brain imaging.
Preamble to 3D Brain Imaging
The landscape of medical imaging has evolved significantly, with three-dimensional brain imaging standing at the forefront of this progress. Understanding the brain requires not only the visual representation of its structures but also the dynamic interactions within. 3D brain imaging provides a crucial avenue to achieve this understanding, enabling researchers and clinicians to diagnose, study, and treat various neurological conditions effectively.
Understanding Brain Imaging
Brain imaging encompasses all techniques designed to visualize the anatomy and function of the brain. Techniques like Magnetic Resonance Imaging (MRI), Computed Tomography (CT), and others are pivotal in shaping our knowledge. Each method has its unique advantages and limitations, making it essential to choose the appropriate imaging technique based on the clinical or research question.
- Precision: 3D imaging offers precise localization of brain structures, facilitating a more accurate interpretation of data.
- Functionality: Beyond merely displaying anatomy, functional imaging techniques assess brain activity, critical for understanding conditions like epilepsy or brain tumors.
- Integration: The integration of structural and functional imaging enhances the understanding of disease mechanisms, providing insights that could lead to better therapeutic strategies.
Historical Context
The history of brain imaging is rich and complex, tracing back over a century. Initial techniques were rudimentary, but they have progressively advanced into sophisticated modalities that allow intricate exploration of brain functionality.
In the early days, methods such as X-rays were the mainstay for brain imaging. However, as technology progressed, so did our understanding:
- CT Imaging Emergence: The introduction of Computed Tomography in the 1970s changed everything. It allowed for detailed cross-sectional images of the brain, significantly improving diagnostic capabilities.
- Advent of MRI: In the 1980s, Magnetic Resonance Imaging emerged, offering superior image quality without the exposure to ionizing radiation. This technique marked a pivotal change in neuroimaging, enabling better visualization of soft tissues.
- Functional Imaging Breakthrough: The development of functional MRI in the 1990s added another dimension, providing insights into brain activity in real-time.
These historical advancements set the stage for the current landscape of 3D brain imaging, demonstrating its evolution from basic images to complex, detailed, and functional representations that are widely utilized in modern neuroscience.
"The evolution of brain imaging techniques illustrates a journey toward greater accuracy and understanding of the brain's complexities."
As we examine these advancements, it is clear that the implications for research and clinical application are profound, underscoring the necessity of 3D brain imaging in contemporary neuroscience.
Principles of 3D Imaging Techniques
Understanding the principles of 3D imaging techniques is essential in grasping how these technologies transform the field of neuroscience and medicine. This section will explore various imaging modalities, discussing their core principles, benefits, and specific applications. The value of these techniques cannot be overstated; they allow researchers and clinicians to visualize and analyze complex structures within the brain with remarkable precision. By detailing the key imaging techniques, it becomes clear how they contribute to advancements in diagnosing and treating neurological conditions.
Magnetic Resonance Imaging (MRI)
Magnetic Resonance Imaging (MRI) stands out due to its ability to provide high-resolution images of the brainโs anatomy. The technique is based on the principles of nuclear magnetic resonance, which exploits the magnetic properties of atomic nuclei. In MRI, hydrogen protons found in water molecules within the body respond to a magnetic field and radiofrequency energy, producing detailed images.
Key features and benefits of MRI include:
- No ionizing radiation: Unlike CT scans, MRI does not use harmful radiation, making it safer for repeated studies.
- Excellent soft tissue contrast: MRI excels at distinguishing between different types of soft tissues, crucial for identifying tumors or lesions.
- Functional imaging capabilities: Techniques like functional MRI (fMRI) go beyond structural imaging, allowing researchers to observe brain activity in real-time based on blood flow changes.
Computed Tomography (CT)
Computed Tomography (CT) is a widely used imaging modality that employs X-ray technology to produce detailed cross-sectional images of the brain. By rotating around the patient, a CT scanner captures multiple images from different angles, which are then reconstructed into a 3D model using computer algorithms.

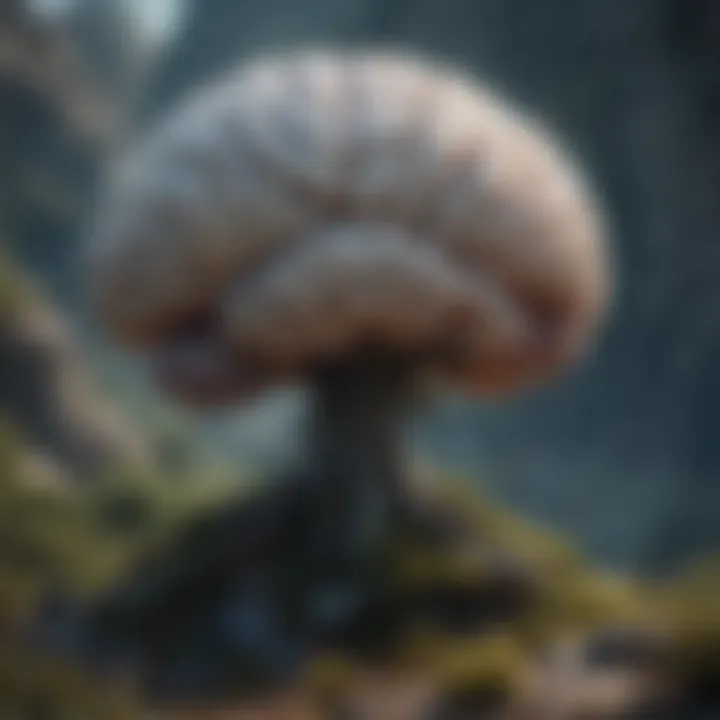
Some notable aspects of CT include:
- Speed: CT scans are typically faster than MRI, essential in emergency scenarios to quickly assess traumatic brain injuries.
- Bone detail visualization: CT provides excellent imagery of bony structures, making it suitable for detecting fractures or abnormalities in the skull.
- Cost-effectiveness: Generally, CT scans are more affordable than MRI, contributing to their widespread use in medical practice.
Positron Emission Tomography (PET)
Positron Emission Tomography (PET) represents a unique approach by utilizing radioactive tracers to visualize metabolic processes in the brain. This imaging technique provides insights into brain function by detecting gamma rays emitted when a positron emitted by a radioactive substance interacts with electrons in the body.
PET is particularly useful for:
- Determining brain metabolism: By monitoring glucose consumption, PET can help identify areas of the brain that may be functioning abnormally, such as in Alzheimer's disease.
- Research applications: PET is invaluable in understanding neurodegenerative diseases and the effects of various treatments on brain metabolism.
- Combining modalities: When combined with CT or MRI, PET offers a comprehensive view that includes both structural and functional data.
Functional MRI (fMRI)
Functional MRI (fMRI) builds upon traditional MRI technology to assess brain activity by measuring changes in blood flow. The premise is based on the fact that activated brain areas require more oxygen, leading to localized increases in blood flow โ a concept known as the hemodynamic response.
The advantages of fMRI include:
- Non-invasive: fMRI enables the measurement of brain activity without surgical intervention.
- Real-time monitoring: Researchers can visualize brain activity during specific tasks, making it an important tool for cognitive neuroscience.
- Versatile applications: fMRI is extensively used in understanding language, memory, and emotion processes in the brain.
"Functional MRI has revolutionized our understanding of the brain's intricacies, offering insights into both its structure and function."
Comparative Analysis of 3D Imaging Modalities
The comparative analysis of 3D imaging modalities is essential to grasp the diverse landscape of techniques in brain imaging. Understanding the differences and similarities among these methods allows researchers, clinicians, and educators to make informed decisions based on specific needs and applications. Different technologies offer unique benefits and face various limitations. This analysis will focus on three critical factors: resolution and image quality, speed of imaging techniques, and cost implications.
Resolution and Image Quality
Resolution directly influences the clarity and detail of images produced by different imaging modalities. Magnetic Resonance Imaging (MRI) is known for its high-resolution capabilities, capturing intricate details of brain structure. This is vital for identifying abnormalities such as tumors or lesions. On the other hand, Computed Tomography (CT) offers lower resolution in comparison, but it excels in quickly identifying hemorrhages and bone fractures.
Positron Emission Tomography (PET) combines functional data with metabolic information, presenting a different dimension of analysis. Its resolution may not match MRI but reveals critical insights into brain metabolism. As for Functional MRI (fMRI), it provides information on brain activity by detecting changes in blood flow. While its spatial resolution is good, it may lack temporal resolution, complicating the interpretation of rapid brain activity.
"The choice of imaging modality heavily depends on the specific clinical or research question at hand."
Speed of Imaging Techniques
The time required to obtain images varies significantly across modalities. CT is the fastest imaging technique, often taking just a few minutes. This speed makes it favorable in emergency situations where time is critical. MRI, however, is generally slower as it can take 30 minutes to over an hour. This duration is due to the complexity of acquiring images, as well as the need for patient stillness during the scan.
In contrast, PET scans require even longer, typically ranging from 30 minutes to several hours, accounting for the time needed to inject the radiotracer and allow it to circulate. The speed of fMRI sits in-between these methods, taking about 10 to 15 minutes, making it suitable for both research and clinical use but still reliant on the cooperation of the patient.
Cost Implications
The financial aspect of choosing an imaging technique cannot be overlooked. MRI is usually more expensive than CT due to its advanced technology and maintenance costs. The cost of a single MRI scan can range significantly based on various factors including location and the complexity of the imaging required. In contrast, a CT scan tends to be more budget-friendly, making it accessible in many healthcare settings.
PET scans are among the most costly due to the intricate technology and the expense of radiotracers. fMRI pricing generally aligns with MRI but is influenced by its specialized application. Thus, financial considerations play a crucial role in determining which modality to use, particularly in resource-constrained environments.
In summary, the comparative analysis of 3D imaging modalities highlights key factors that influence the choice of brain imaging techniques. Resolution and image quality, speed of imaging techniques, and cost implications are pivotal in deciding which modality best suits a particular requirement, whether for research or clinical applications.
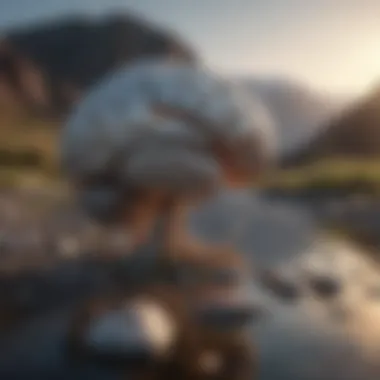
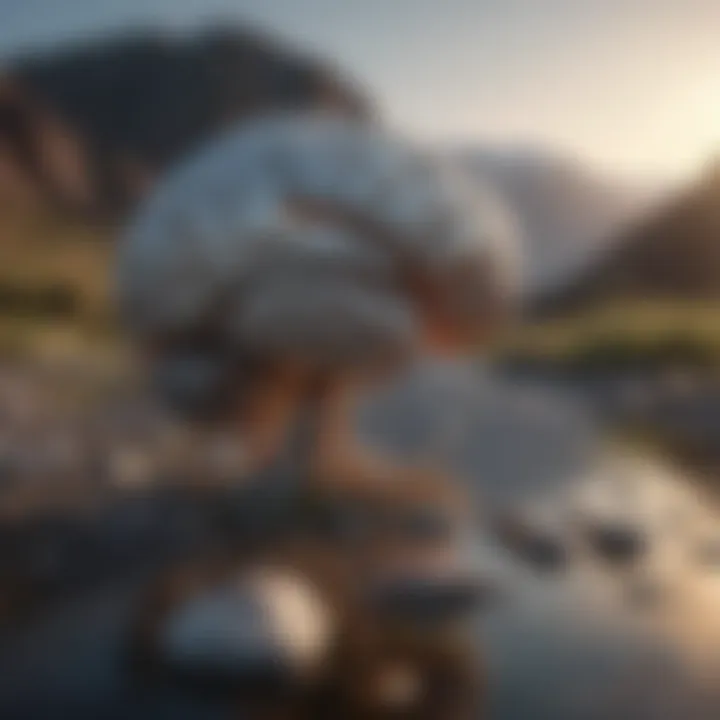
Applications of 3D Brain Imaging
The field of three-dimensional brain imaging offers profound implications across various domains. Understanding and applying these imaging technologies is essential for improving neurological research and clinical practices. As we examine the applications of 3D brain imaging, we will uncover specific areas where these technologies are significantly changing the landscape.
Research in Neuroscience
In neuroscience, 3D brain imaging plays a pivotal role in advancing our comprehension of brain structure and function. Techniques such as MRI and PET enable researchers to visualize the brain's anatomy and its activity in real time. This capability is critical for exploring neurodevelopmental processes, neurodegenerative diseases, and brain connectivity. The following are important benefits of utilizing 3D imaging in neuroscience research:
- Structural Insights: 3D images provide detailed structures of brain anatomy, allowing researchers to identify variations and abnormalities.
- Functional Mapping: Technologies like fMRI measure brain activity by detecting changes in blood flow, offering a dynamic view of functional areas during various tasks.
- Longitudinal Studies: Researchers can track changes over time in individuals, aiding studies on aging, cognitive decline, and disease progression.
Furthermore, these imaging methodologies enhance the collaboration between disciplines, encouraging multidisciplinary approaches that lead to innovative findings.
Clinical Applications
3D brain imaging has invaluable applications in the clinical setting. It aids in diagnosing various neurological disorders and guiding multifaceted treatment plans. The significance of these applications includes:
- Diagnosis: High-resolution imaging helps identify conditions such as tumors, stroke, and epilepsy by providing clear images of brain structures.
- Treatment Monitoring: Imaging allows clinicians to assess how well a treatment is working by visualizing the brain's response over time.
- Tailored Therapies: 3D imaging data can facilitate personalized treatment strategies, helping medical professionals choose the most effective interventions for individual patients.
Clinical usage also entails the integration of imaging data with other medical information, promoting comprehensive patient assessments that can lead to improved outcomes.
Pre-surgical Planning
One of the notable applications of 3D brain imaging is in pre-surgical planning for neurological surgeries. This involves intricate procedures such as tumor removals or epilepsy surgeries. Here are several key aspects:
- Detailed Visualization: Pre-operative imaging provides surgical teams with essential information about the tumor's location and its relationship to critical brain structures. This minimizes risks and improves surgical precision.
- Functional Mapping: Surgeons can utilize functional imaging to identify areas responsible for essential functions like movement and speech, ensuring these regions are preserved during surgery.
- Simulation of Procedures: Advanced imaging can be used to create simulations of surgical approaches, allowing the surgical team to strategize the best technique for reaching the target while minimizing damage to surrounding tissues.
Overall, the applications of 3D brain imaging are expansive. They lead to enhancements in understanding brain functions and disorders, bolster diagnostic accuracy, and support precise surgical interventions. As technology continues to evolve, so too will the potential of 3D brain imaging to transform practices in neuroscience and clinical care, leading us into a future where brain-related ailments are managed with increasing sophistication.
"The intersection of technology and neuroscience is producing new tools that improve our understanding of the brain and its complex functions. " - Neuroscience Today
These multifaceted applications affirm the critical need for ongoing research and innovation in the field of 3D brain imaging.
Ethical Considerations in 3D Brain Imaging
The rapid advancements in 3D brain imaging technologies raise significant ethical considerations. Understanding these issues is crucial for researchers, clinicians, and policymakers. Brain imaging, by its very nature, delves into the most complex and intimate of human experiences. With the ability to view the structure and activity of the brain comes the responsibility to address issues related to privacy, consent, and potential misuse.
Privacy and Data Protection
Privacy in brain imaging is a critical concern. The data obtained from imaging techniques like MRI, CT, and fMRI can reveal sensitive information about individuals. This includes not only anatomical details but also insights into thoughts, emotions, and potential health risks. Such data can easily lead to misuse, including discrimination by employers or insurance companies.
"Protecting patient information is paramount in developing trust and integrity in scientific research."
To mitigate risks, it is essential to implement robust data protection measures. This includes anonymizing data wherever possible and ensuring strict access controls on sensitive information. The challenge lies in balancing the need for research with the individual's right to privacy. Without stringent policies and adherence to ethical standards, the potential for data breaches remains a pressing issue.
Informed Consent
Informed consent forms the backbone of ethical research practices. It means that participants should fully understand the nature of the study, including how their data will be used and what it might reveal. In the realm of 3D brain imaging, this can get complicated. The technology may uncover aspects of a person's cognitive state, which can be unexpected or distressing.

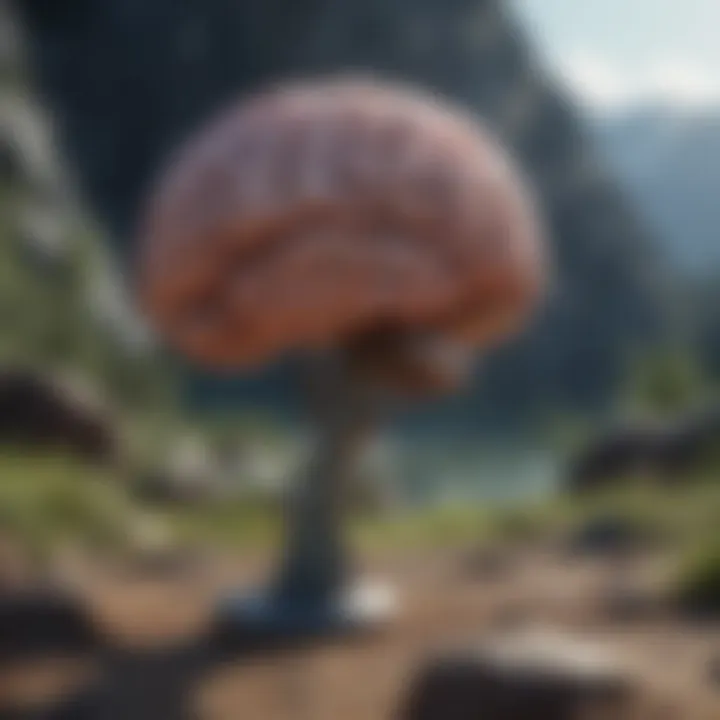
Participants should be provided with clear information about the implications of their involvement. This includes potential discoveries that might affect their psychological well-being or future treatment options. Researchers must ensure that consent is obtained in a manner that respects the autonomy of individuals and their rights to make informed choices about their own health information.
Potential for Misuse
The possibilities afforded by 3D brain imaging come with inherent risks of misuse. One prime concern is the potential for exploitation in legal settings. For instance, imaging results could be used to either incriminate or exonerate individuals based on their brain activity. This raises troubling questions about determinism and free will.
Moreover, there could be misuse in the form of enhancement or modification of brain functions, leading to ethical quandaries around what it means to be human. As technology continues to advance, ensuring ethical oversight and governance in this field becomes imperative.
Ultimately, the ethical considerations surrounding 3D brain imaging necessitate ongoing dialogue and reflection. Engaging various stakeholders, including ethicists, clinicians, and patients, is essential as the technology continues to evolve. The ethical framework must adapt to these changes to safeguard individuals while promoting innovative research in neuroscience.
Innovations on the Horizon
Innovations in 3D brain imaging hold considerable promise for the future of neuroscience and clinical practices. These advancements provide insight into how we can enhance our understanding of the human brain and its complexities. The interplay between technology and neuroscience is pivotal. As we explore innovations such as machine learning, improved image processing techniques, and the integration of various imaging modalities, we open avenues for better diagnosis, treatment, and patient outcomes.
Machine Learning in Imaging
Machine learning is transforming how we process and interpret brain imaging data. By applying algorithms that can learn from patterns in large datasets, researchers can enhance the accuracy of image analysis. This approach can lead to earlier detection of conditions like Alzheimer's disease or multiple sclerosis. For instance, machine learning algorithms can identify subtle changes in brain structure or function that might escape human observation. These tools can provide radiologists and neurologists with critical insights, ultimately improving diagnostic precision.
With the continuous influx of imaging data, machine learning's role becomes ever more crucial. It can automate time-consuming tasks and allow professionals to focus on complex cases. The adaptation of machine learning in imaging showcases a significant shift in the methodology of neuroscience research and clinical practice.
Advancements in Image Processing
Recent advancements in image processing have made it possible to obtain clearer and more detailed images of the brain. Techniques such as advanced filtering, 3D reconstruction, and enhanced resolution algorithms are becoming standard in imaging protocols. These innovations result in high-fidelity images, aiding in the understanding of brain anatomy and pathology.
Moreover, improved image processing can facilitate real-time analysis during procedures. For example, during neurosurgery, immediate access to high-quality imaging can guide the surgeon's decisions. This reduces risks associated with surgical interventions, allowing for safer and more effective patient care.
Integration of Imaging and Neuroscience
The integration of imaging technologies with neuroscience research is setting a new standard in understanding brain functionality. Without a doubt, combining modalities like MRI and PET can provide a more holistic view of brain activity and structure. This synergy allows researchers to explore complex interactions within the brain more effectively.
Not only does integration enhance research clarity, but it also fosters interdisciplinary collaboration. Neuroscientists, radiologists, and data scientists can work together to develop new hypotheses and test them using innovative imaging techniques. As research in this area progresses, the potential for new discoveries increases significantly.
"The intersection of advanced imaging technologies and neuroscience could lead to breakthroughs that we cannot yet imagine."
The End
The conclusion serves as a vital component of this article, encapsulating the key insights attained throughout the exploration of 3D brain imaging technologies. It synthesizes the extensive information presented in previous sections, offering the reader a clear view of how these advancements influence both research and clinical practices.
Summation of Findings
The findings from this article highlight several significant achievements in the realm of 3D brain imaging. Firstly, technologies like MRI, CT, PET, and fMRI have transformed our capability to visualize and understand brain anatomy and function. Each modality offers distinct advantages, such as resolution, speed, and cost-effectiveness, catering to various needs in both clinical and research settings.
In clinical applications, 3D brain imaging plays a crucial role in diagnosing neurological disorders. For instance, functional MRI provides real-time insights into brain activity, aiding in the assessment of conditions like epilepsy or stroke. Additionally, these imaging techniques are pivotal in pre-surgical planning, ensuring that interventions are performed with maximum precision and minimal risk.
Moreover, ethical considerations cannot be overlooked. Issues related to privacy, informed consent, and potential misuse of imaging data are integral to the ongoing dialogue about the responsible use of these technologies.
Future Directions in 3D Brain Imaging
Looking forward, the landscape of 3D brain imaging is poised for rapid advancements. Machine learning techniques are gradually becoming integral to the field, enhancing image processing and analysis. This integration promises improved accuracy and efficiency in diagnosing brain disorders. New tools will allow researchers to explore brain functions and structures with previously unattainable detail.
Innovative imaging techniques are also on the horizon. For instance, hybrid imaging methods that combine CT and MRI could offer comprehensive views of brain structures alongside metabolic information. Additionally, the ongoing collaboration between neuroscience and engineering fields is likely to yield new methods for studying brain dynamics.
In summary, the future of 3D brain imaging appears bright, driven by technological innovations and interdisciplinary cooperation. As advancements continue, they will undoubtedly reshape both research methodologies and clinical practices, expanding our understanding of the brain.