Bacterial Two-Hybrid System: A Comprehensive Overview
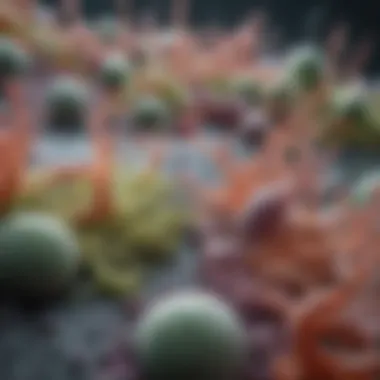
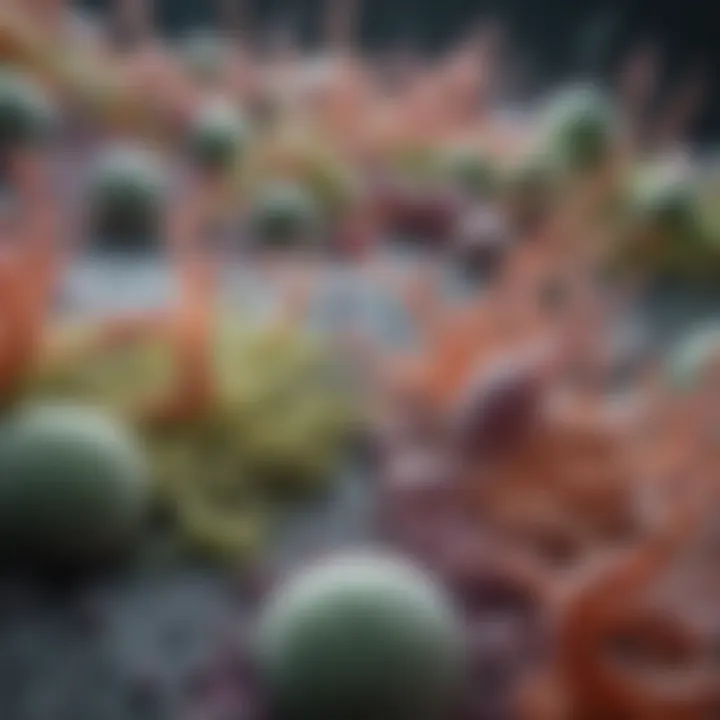
Intro
The field of molecular biology constantly evolves, demanding innovative techniques for exploring complex biological processes. One such technique is the bacterial two-hybrid system, which has emerged as an invaluable tool for investigating protein-protein interactions. By utilizing this system, researchers can discern how proteins interact within a cellular context, providing crucial insights into various biological functions.
In the following sections, we will examine the intricacies of this system. Starting with the overarching research overview, we will summarize the key findings and provide a solid background for understanding its significance. This will be followed by a detailed description of the methodology, breaking down the experimental design and data collection techniques employed in two-hybrid assays.
Prelims to Bacterial Two-Hybrid System
The bacterial two-hybrid system plays an essential role in contemporary molecular biology. It offers a versatile platform for investigating protein-protein interactions, which are fundamental to a myriad of biological processes. Understanding these interactions can elucidate pathways and cellular functions that are crucial for both basic and applied research. Thus, this system serves as a bridge connecting fundamental biology to practical applications in biotechnology and drug discovery.
Definition and Purpose
The bacterial two-hybrid system is a research method used to detect and analyze interactions between two proteins within a bacterial environment, specifically in Escherichia coli. The underlying principle is rooted in the idea of reconstituting a functional transcription factor when two proteins of interest bind to each other. In simple terms, it helps researchers identify whether two proteins interact, how strong that interaction is, and potentially what that interaction implies for biological functions.
The primary purpose of the bacterial two-hybrid system includes:
- Identification of Protein Interactions: It provides data on which proteins bind together, an important factor in understanding cellular mechanisms.
- Studying Protein Functions: By analyzing the interactions, scientists can infer the roles of specific proteins in diverse biological contexts.
- Drug Development: Information obtained can guide therapeutic strategies, especially in targeting specific pathways in disease.
This methodology is crucial because direct observation of protein interactions in a physiological context is often challenging. The bacterial two-hybrid system thus offers a controlled environment to study these dynamic interactions.
Historical Development
The inception of the bacterial two-hybrid system can be traced back to the late 1980s and early 1990s, when scientists began developing methods to identify protein interactions in more manageable systems outside of eukaryotic environments. The early models were based on yeast, known for their ability to support eukaryotic proteins' interactions. However, researchers recognized limitations in scale and efficiency when working with more complex organisms.
In 1996, the bacterial two-hybrid system was introduced as an alternative. This system utilized E. coli to simplify the experimental framework while maintaining the essential parameters necessary for studying protein interactions. The direct use of bacteria allowed for higher throughput and easier manipulation, leading to a broader application in genetic research and molecular biology.
Since then, advancements have been made in improving the sensitivity and specificity of the system. Variants have emerged, enhancing the original model's capabilities, while still focusing on the efficient identification of interacting proteins.
The evolution of this system illustrates the scientific community's adaptability and innovation in exploring the intricacies of protein behaviors. As a result, the bacterial two-hybrid system remains a foundational approach for researchers seeking to unlock the complexities of cellular interactions.
Mechanism of the Bacterial Two-Hybrid System
The mechanism of the bacterial two-hybrid system is crucial for understanding how protein-protein interactions are discerned. This sophisticated technique combines genetics and biochemistry to facilitate the identification of proteins that interact within bacterial cells. By utilizing this method, researchers can elucidate the dynamics of cellular processes and provide insights into various biological functions. The robustness and adaptability of this system make it a fundamental tool, especially in fields such as molecular biology and biotechnology.
Basic Principles
The basic principle of the two-hybrid system relies on the reconstitution of a functional protein from two separate fragments. When two proteins of interest interact, they bring the fragments together, enabling expression of a reporter gene. This gene serves as an indicator of the interaction, allowing scientists to infer that two proteins are likely to be interacting within the cellular environment. The setup simplifies the complexity of studying interactions by using a bacterial host, often Escherichia coli, where the assay can be easily controlled and monitored.
Components Involved
DNA Binding Domain
The DNA binding domain (DBD) plays an essential role in the functioning of the bacterial two-hybrid system. It is responsible for targeting specific DNA sequences, thereby allowing the expression of the reporter gene only when protein interactions are established. The DBD's key characteristic is its ability to bind to specific regulatory elements within the promoter region of reporter genes. This specificity is what makes the DBD a beneficial choice for this system. Additionally, a unique feature of the DBD is its modular design, which often allows flexibility in targeting various genes, enhancing the overall versatility of the system. However, one consideration is that the effectiveness of the DBD can sometimes vary based on context, potentially influencing the results.
Activation Domain
The activation domain (AD) is vital for facilitating gene expression once the proteins of interest are found to interact. The AD's primary function is to recruit RNA polymerase and other transcriptional machinery. This domain is known for its activation capabilities; thus, it is a crucial tool in the bacterial two-hybrid system as it amplifies the interaction signal. A notable aspect of the AD is that it can vary widely between different organisms, allowing for cross-species applications. However, the advantage of this diversity also introduces a challenge, as different activation domains may lead to varying efficiencies in different contexts or host strains.
Reporter Genes
Reporter genes are the final component that allows visualization of protein interactions within the bacterial two-hybrid system. When proteins interact, the reporter gene is expressed, and its activity can be easily measured using various assays. One noteworthy characteristic of reporter genes is their simplicity in detecting interactions, which makes them a popular choice in molecular biology research. They can range from enzymes that produce a color change to luminescent proteins that emit light. A unique feature of reporter genes is their ability to provide quantitative data, which enhances the reliability of experimental results. However, the dependence on accurate expression levels means that careful optimization is essential to avoid artifacts that may lead to misinterpretation of results.
"The implementation of the bacterial two-hybrid system significantly streamlines the process of identifying protein interactions, making it a cornerstone in modern molecular biology."
Experimental Design
The experimental design phase is crucial in the application of the bacterial two-hybrid system. This stage determines the success of protein interaction assays. A well-structured design not only outlines the objectives but also ensures clarity and reproducibility in the results. In this context, several key elements must be considered, ranging from construct preparation to selection and screening processes.
Construct Preparation
Construct preparation involves the design and assembly of plasmids encoding the proteins of interest. This step is essential, as the way these constructs are generated directly affects the outcome of the experiment. Researchers must optimize the methods of linking DNA binding and activation domains to the respective proteins. Careful attention to the sequence and orientation of the inserts can significantly influence the interactions observed.
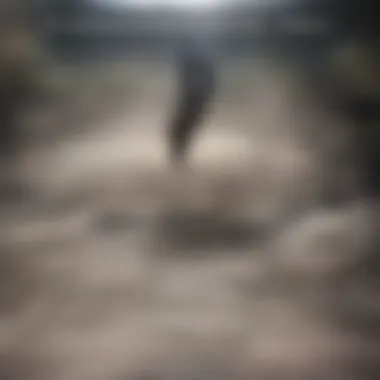
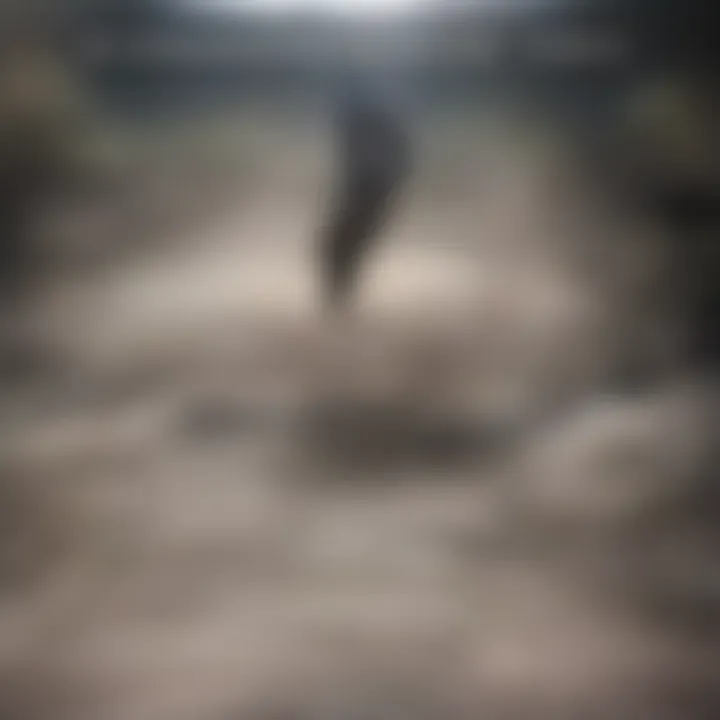
Using polymerase chain reaction (PCR) techniques or restriction enzyme digestion assists in creating the desired constructs. Additionally, confirming the constructs through sequencing is vital to ensure accuracy. This initial stage plays a vital role in preventing downstream errors that could lead to misinterpretation of results.
Transformation of Host Cells
Once construct preparation is complete, the next phase involves the transformation of host cells. Escherichia coli is commonly employed for this purpose, owing to its well-characterized genetics and ease of manipulation. The methods for transformation vary but often include electroporation and heat shock techniques.
Successful transformation results in the incorporation of plasmids into the bacterial cells, allowing for the expression of the protein constructs. Factors such as the choice of media, incubation time, and temperature can impact transformation efficiency. Therefore, optimizing these parameters is essential for achieving high rates of transformation.
Selection and Screening
After transformation, it is important to select and screen the host cells that successfully incorporated the constructs. This often involves the use of selective media containing antibiotics or specific nutrients. Only the cells that have taken up the plasmids will grow under these selective conditions. Screening typically involves assays that detect protein interactions, commonly using reporter genes such as lacZ, which encodes for β-galactosidase.
In this stage, researchers assess colonies for the expression of the reporter gene, which indicates successful interaction between the proteins of interest.
The reliability of experimental outcomes is highly dependent on well-planned design and thorough execution of selection and screening protocols.
Types of Bacterial Two-Hybrid Systems
Understanding the various types of bacterial two-hybrid systems is essential for researchers aiming to explore protein-protein interactions. Each method offers unique features and benefits, catering to different experimental needs and goals. Knowing which system to utilize can greatly influence the accuracy and efficiency of identifying interactions.
Classic Bacterial Two-Hybrid System
The classic bacterial two-hybrid system relies on the reconstitution of a transcription factor to detect interactions between proteins. This method uses a plasmid containing separate genes that encode for a DNA binding domain and an activation domain. When two proteins of interest interact, these domains come together, activating the expression of a reporter gene. The simplicity of this approach is one of its major strengths, allowing for straightforward analysis of binary interactions.
This system is often used for initial screenings of interactions. However, it could also yield false positives. Proper controls and replications are needed to validate results, which adds to the complexity of interpretation.
Variations and Enhanced Systems
Over time, researchers have developed several enhancements to the classic system, improving its sensitivity and applicability.
Split-Luciferase System
The Split-Luciferase System significantly improves detection capabilities through the use of luciferase fragments. When two proteins interact, the fragments come together to form an active luciferase. This allows for a highly sensitive luminescence readout. The key characteristic of this system is its high sensitivity, which makes it a preferred choice when studying weak or transient interactions.
One unique feature of the split-luciferase system is that it can allow for real-time monitoring of interactions. This is advantageous in dynamic studies. However, it also requires careful calibration and control to ensure accurate luminescence readings.
Split-GFP System
Another variation is the Split-GFP System, which utilizes fragments of the green fluorescent protein (GFP). In this system, the proteins of interest are tagged with different fragments of GFP. Interaction between these proteins leads to the reconstitution of the fluorescent signal. This bright output serves as a reliable indicator of interactions.
A major benefit of the Split-GFP System is its non-invasive nature, allowing for interactions to be studied within living cells. However, the main drawback is the reliance on proper expression levels of the GFP fragments, which can complicate interpretation.
Dual-Luciferase System
The Dual-Luciferase System offers a more robust approach by allowing the measurement of two different luciferase activities simultaneously. This creates a normalized value which can be extra useful for assessing interactions against control experiments. The key aspect of this method is the use of two distinct luciferase reporters. This enhances the reliability of results, especially in comparative studies.
The unique feature of dual-luciferase is its ability to provide more context around interactions by distinguishing between two different outcomes in the same experiment. However, optimization of both luciferase outputs is critical. Misalignment can lead to misleading data interpretations.
In summary, while the classic bacterial two-hybrid system has its own merits, advancing through these variations provides researchers with enhanced tools for detecting protein interactions. Each method has its own pros and cons, requiring careful consideration based on the specific context of the research question.
Applications of the Bacterial Two-Hybrid System
The bacterial two-hybrid system is a significant tool in molecular biology, essential for exploring the intricate world of protein interactions. Proteins often work together in complexes, making it crucial to understand how they influence each other's functions within cells. The applications of this system are diverse and impactful, driving progress in various fields, from fundamental biology to applied sciences.
Studying Protein Interactions
Studying protein interactions is one of the primary applications of the bacterial two-hybrid system. This method allows researchers to reveal how proteins interplay within cellular processes. By fusing proteins of interest to specific domains, it becomes possible to detect interactions through the activation of reporter genes. This can lead to the identification of novel partners in various pathways that govern cell function.
Key advantages of using this system include:
- High Throughput: The capacity to screen many interactions simultaneously allows substantial data collection, which is essential for comprehensive functional studies.
- Cost-Effective: Compared to other methods, such as co-immunoprecipitation, the bacterial two-hybrid system is generally less expensive and quicker to implement.
- Simplicity: Working within a prokaryotic system can simplify the complexities associated with eukaryotic organisms.
In essence, understanding protein interactions sheds light on various biological phenomena, such as signal transduction, gene regulation, and cellular responses to environmental cues. This knowledge is vital for advancing fundamental biology and therapeutic approaches.
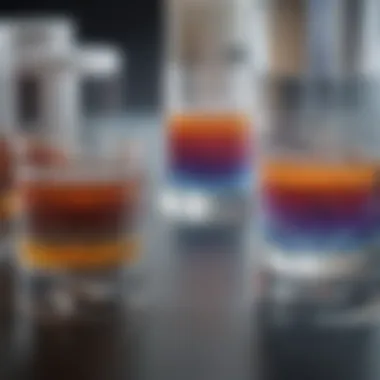
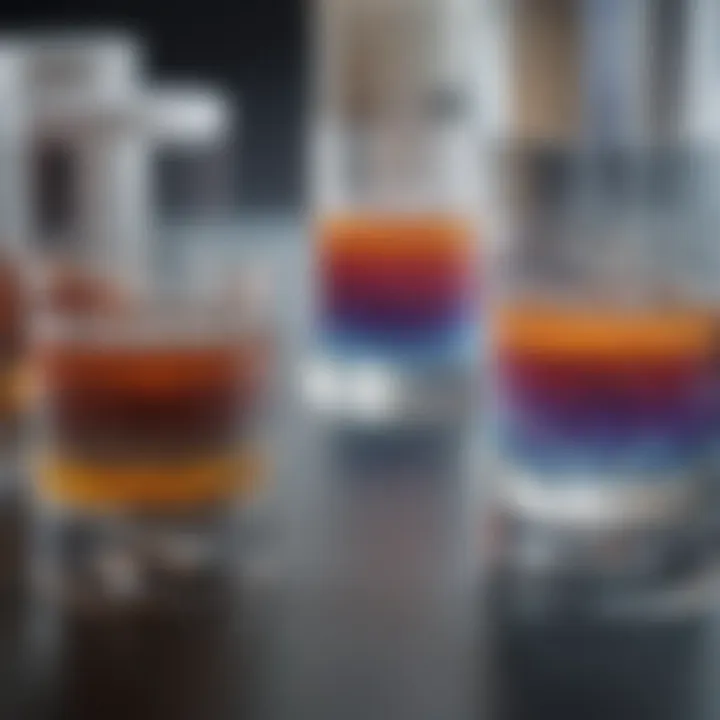
Drug Discovery and Development
The bacterial two-hybrid system holds great potential in the realm of drug discovery and development. Understanding how target proteins interact with potential drug compounds is critical for the design of effective therapeutic agents. By utilizing this system, researchers can screen for small molecules that disrupt or enhance specific protein interactions.
Moreover, the system can help identify new drug targets. This is particularly pertinent in conditions where current therapies are insufficient. For example:
- Target Identification: Through protein interaction studies, novel therapeutic targets can be elucidated for diseases like cancer or neurodegenerative disorders.
- Mechanism Understanding: Knowing how drugs interact with specific proteins helps clarify their mechanisms of action, improving drug design.
- High-throughput Screening: The system can be adapted for screening large libraries of potential drugs, significantly accelerating the process of drug discovery.
These advantages make the bacterial two-hybrid system a promising candidate for modern pharmacological research.
Functional Genomics
Functional genomics aims to understand the functions of genes and their products. The bacterial two-hybrid system plays a crucial role in this area by facilitating the identification of gene functions through protein interactions. It helps scientists build networks of interactions that define the cellular landscape.
In functional genomics, critical advantages of this system include:
- Gene Function Exploration: With the ability to study protein interactions in a systematic way, researchers can infer the roles of genes based on their interaction profiles.
- Pathway Analysis: By mapping out interactions, the system enables the reconstruction of metabolic and signaling pathways, providing a broader context for understanding cellular functions.
- Understanding Disease Mechanisms: Insights into how specific proteins interact can reveal the underlying mechanisms of various diseases, paving the way for targeted research and therapeutic strategy development.
As researchers continue to harness the potential of the bacterial two-hybrid system, it will likely remain a cornerstone tool in advancing our understanding of biology at a molecular level.
Limitations of the Bacterial Two-Hybrid System
Understanding the limitations of the bacterial two-hybrid system is crucial for interpreting its results accurately. While this method is a powerful approach in molecular biology for studying protein interactions, it is not without drawbacks. These limitations can influence the reliability and validity of the data generated through this experimental design.
False Positives and Negatives
One significant issue in the bacterial two-hybrid system is the occurrence of false positives and false negatives.
False Positives: These occur when the system incorrectly indicates a protein-protein interaction that does not actually exist. This can result from several factors, including:
- Non-specific interactions: Proteins may interact with each other in an unintended manner due to inherent properties of the host bacteria.
- Overexpression effects: When proteins are overexpressed in bacteria, they might engage in interactions not observed under physiological conditions.
False Negatives: Conversely, false negatives occur when actual interactions are not detected. This can happen due to:
- Suboptimal conditions: Factors such as temperature, pH, and media components can impact the effectiveness of interactions being detected.
- Weak interactions: Some interactions, especially those not constitutively strong or transient in nature, may escape detection by the system, leading to missed opportunities in discovering relevant interactions.
False positives and negatives can significantly skew the interpretative value of the findings, leading to misguided research conclusions.
Contextual Limitations
The contextual limitations of the bacterial two-hybrid system also warrant examination. These limitations arise from the reliance on specific environmental and biological contexts for protein interactions to be accurately studied. Key factors include:
- Host Cell Environment: Bacterial cells offer a different intracellular milieu compared to eukaryotic cells. This discrepancy can impact the folding and modification of proteins, leading to altered behavior and functionality.
- Protein Modifications: Many eukaryotic proteins undergo post-translational modifications essential for their activity. The bacterial two-hybrid system does not mimic these processes, which may lead to interactions being misrepresented or overlooked.
- Complexity of Interactions: In vivo, proteins often interact within complex networks. The two-hybrid system oversimplifies these interactions by isolating pairs of proteins, which might not reflect the dynamics of their natural cellular environment.
In summary, while the bacterial two-hybrid system remains a valuable tool for exploring protein interactions, researchers must remain aware of its limitations. Understanding false positives, false negatives, and contextual limitations can guide the refinement of experimental design and interpretation of results, allowing for more accurate conclusions.
Comparative Methods
The exploration of protein-protein interactions is crucial in molecular biology, and understanding the various methodologies available enhances the reliability of findings. Comparative methods in this context highlight the strengths and weaknesses of different experimental approaches. This section will examine the bacterial two-hybrid system in relation to yeast two-hybrid systems and other detection techniques, illustrating their key characteristics and applications.
Comparison with Yeast Two-Hybrid Systems
Yeast two-hybrid systems have been widely utilized to study protein interactions. They allow for a more complex cellular environment for interaction studies due to the eukaryotic nature of yeast. In contrast, the bacterial two-hybrid system simplifies the environment. While both systems aim to detect protein interactions, their applications differ.
Bacterial systems tend to involve less post-translational modification, which can be an advantage or a disadvantage depending on the proteins under study. This lack of complexity may lead to simpler, clearer results for specific interactions but can miss interactions dependent on eukaryotic modifications. Yeast two-hybrid systems may provide a broader context of interactions that occur in vivo.
The choice between these methods often relies on the specific research question. For example, if researchers want to understand interactions in a simplified model, they might opt for a bacterial approach. On the other hand, if they are studying eukaryotic proteins that often require specific scaffolding, yeast-based techniques may be preferable.
Other Detection Techniques
Bimolecular Fluorescence Complementation
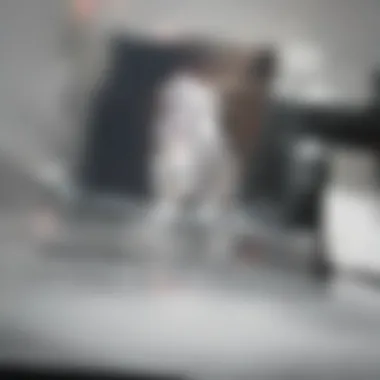
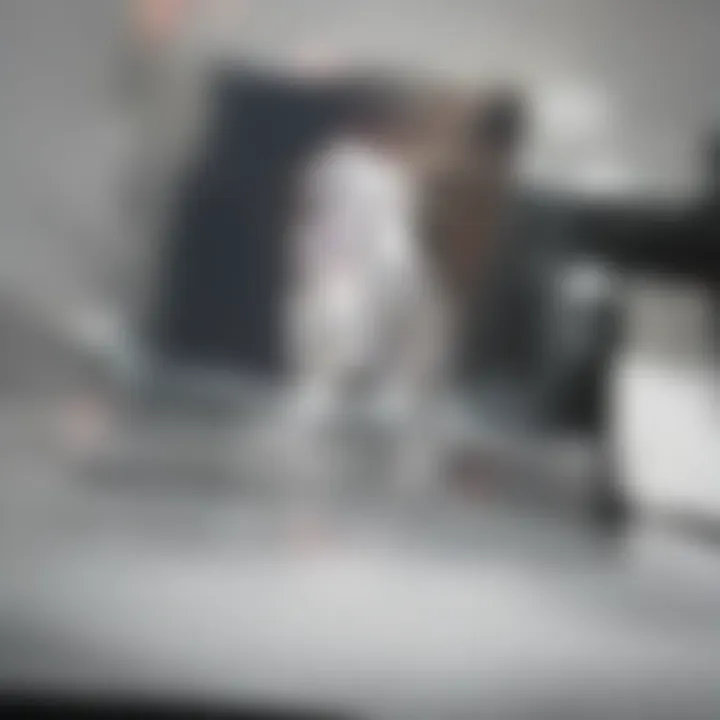
Bimolecular Fluorescence Complementation (BiFC) is an innovative technique used to examine protein interactions within live cells. This method involves splitting a fluorescent protein into two non-fluorescent fragments that can be tagged to the proteins of interest. When the two tagged proteins interact, the fragments come together, restoring fluorescence. Thus, BiFC serves as a powerful tool for visualizing interactions in real time within the cellular context.
A key characteristic of BiFC is its ability to provide spatial and temporal resolution of interactions, making it popular in many research scenarios. Unlike conventional methods that may require cell lysis, BiFC allows researchers to observe interactions as they naturally occur.
However, BiFC also has its limitations. The requirement for specific folding and maturation of the fluorescent protein fragments can sometimes lead to misleading results. Additionally, the potential for non-specific interactions weighs against its use in cases where precise interaction data is crucial.
Co-immunoprecipitation
Co-immunoprecipitation (Co-IP) is another prominent method for studying protein interactions. This technique uses specific antibodies to isolate a protein complex from a mixture. By pulling down one protein, researchers can determine what other proteins are associated. This provides a snapshot of direct interacting partners within a broader network.
The key advantage of Co-IP is its ability to study interactions in native conditions, retaining post-translational modifications that other methods might not capture. This often results in relevant biological insights about how proteins function together in cellular pathways.
Nonetheless, Co-IP also faces challenges. The technique is dependent on the quality of the antibodies used, and false-positive or negative results can arise. Additionally, it might not distinguish interactions as elegantly as the bacterial or yeast systems, making interpretation of complex data more challenging.
In summary, comparative methods shed light on the advantages and disadvantages of each approach. Each technique contributes uniquely to the understanding of protein interactions. Making an informed choice among these methods allows researchers to gather the most reliable data relevant to their specific studies.
Interpretation of Results
The interpretation of results is a critical phase in the bacterial two-hybrid system. Accurate analysis of interaction data guides researchers towards meaningful conclusions about protein interactions. This step is not merely an extension of the experimental process; it is a vital component that ties together hypothesis formulation, data collection, and scientific understanding. Gaining insights from interaction data can foster advances in various fields, especially in understanding cellular mechanisms and developing novel therapeutic strategies.
Analyzing Interaction Data
Analyzing interaction data involves extracting information from the outcomes of the assays. This encompasses the qualitative and quantitative assessment of how proteins behave when they interact. Key considerations include:
- Data normalization: Standardizing data is essential to compare different interactions effectively. It minimizes variability, ensuring that differences in observed interactions are a function of the protein interactions themselves rather than experimental errors.
- Statistical analysis: Using appropriate statistical methods helps determine the significance of interactions. Methods like t-tests or ANOVA can evaluate differences across multiple conditions or comparisons effectively.
- Control comparisons: It is prudent to include negative and positive controls to validate results. Controls help determine whether observed interactions are true signals or artefacts of the system.
Once this initial analysis is done, more complex assessments can follow, such as mapping the interaction networks to understand better the biological pathways involved.
Validating Outcomes
Validation is necessary to corroborate the findings from the bacterial two-hybrid experiments. Outlined steps include:
- Reiteration of experiments: Repeating the assays under different conditions helps ensure that the observed interactions are reproducible. Consistency in results across multiple trials builds confidence in the findings.
- Alternative methods: Employing complementary techniques, such as co-immunoprecipitation or mass spectrometry, can reinforce the conclusions drawn from the two-hybrid assays. This triangulation of data sources strengthens overall results.
- Functional assays: Examining the biological significance of the interactions through functional assays reveals whether the pairs have relevant roles in cellular processes. This adds another layer of validation beyond mere existence of interaction.
The ultimate goal of validating outcomes is not only to confirm the presence of interactions but also to elucidate their biological implications. A thorough analysis presents clear pathways for future research and potential applications in biotechnology and drug discovery.
Future Perspectives
The future perspectives of the bacterial two-hybrid system (BTHS) point to a significant evolution in our understanding and utilization of this crucial tool in molecular biology. As research progresses, the need for innovations and applications that extend the utility of BTHS into new domains becomes imperative. This section highlights key elements and potential advancements in the field, illustrating how ongoing developments can affect the broader landscape of biotechnology and pharmacology.
Innovations in Bacterial Two-Hybrid Systems
Increasing precision in detecting protein-protein interactions remains a focus in the evolution of BTHS. Recent innovations aim to enhance the sensitivity and specificity of assays. An example includes the introduction of split-protein systems, where proteins of interest are divided into two parts that only form a functional unit upon interaction. This not only reduces background noise but also allows for the detection of transient interactions that traditional systems might miss.
Moreover, advancements in the use of fluorescent reporters have made BTH assays more versatile. Systems using split-GFP technology, for instance, enable researchers to visualize interactions in vivo, facilitating real-time monitoring of cellular processes. This real-time aspect holds promise for dynamic studies that reflect physiological conditions more accurately compared to static assays.
High-throughput screening methods are also being integrated with BTHS, allowing researchers to analyze multiple interactions simultaneously. This scalability can lead to a more comprehensive insight into cellular networks and interactions. Automation in these systems can further streamline processes, enabling quicker research cycles and more extensive datasets for analysis.
Expanding Applications in Biotechnology
The application of bacterial two-hybrid systems extends far beyond fundamental research; it is pushing boundaries in biotechnology. In drug discovery, BTHS provides a scaffold for identifying potential drug targets by exploring specific protein interactions that can be manipulated pharmacologically. Utilizing this method can speed up the identification of novel therapeutic compounds.
In the realm of synthetic biology, BTHS aids in designing new pathways or functions by allowing researchers to map interactions that are otherwise complicated or not well understood. Understanding these relationships can guide the development of engineered organisms that produce valuable bioproducts.
Protein engineering also benefits from the bacterial two-hybrid approach. By elucidating protein interaction networks, scientists can design proteins with tailored interactions, enhancing their stability or activity, which is critical for industrial applications.
As the biological sciences advance, the BTHS will likely evolve to meet changing needs. This adaptability ensures its relevance in future biological research and application, marking it as a vital component of modern molecular biology.
Ending
The conclusion section is crucial because it encapsulates the key findings and significance of the bacterial two-hybrid system, highlighting its contribution to molecular biology and related fields. This article has presented an extensive overview of the principles, methodologies, and innovations associated with this experimental technique. The bacterial two-hybrid system stands as a transformative tool in understanding protein interactions. By elucidating the dynamics of these interactions, researchers can gain insights into cellular mechanisms and develop potential solutions in drug discovery and other applications.
Summarizing Key Insights
In recapping the essential points covered in this article, several critical insights emerge:
- Versatility: The bacterial two-hybrid techniques enable the study of protein-protein interactions in a straightforward yet effective manner.
- Historical Evolution: An understanding of the historical context deepens appreciation for the current methods and innovations.
- Methodological Rigor: Emphasis on careful experimental design, from construct preparation to screening, assures reliability in results.
- Broad Applications: The implications for biotechnology, pharmacology, and functional genomics showcase the relevance of this technique across various research domains.
The significance of the bacterial two-hybrid system continues to grow as advancements in technology and methodologies emerge. It is a cornerstone in the advancement of understanding cellular functions, making way for breakthroughs in science and medicine.