Carbon Capture Geoengineering: An In-Depth Analysis
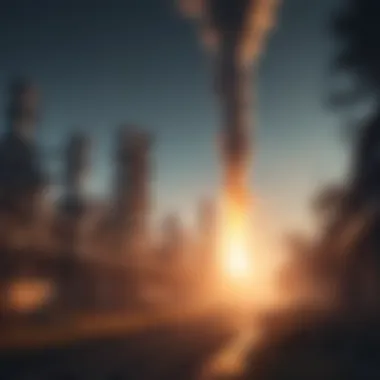
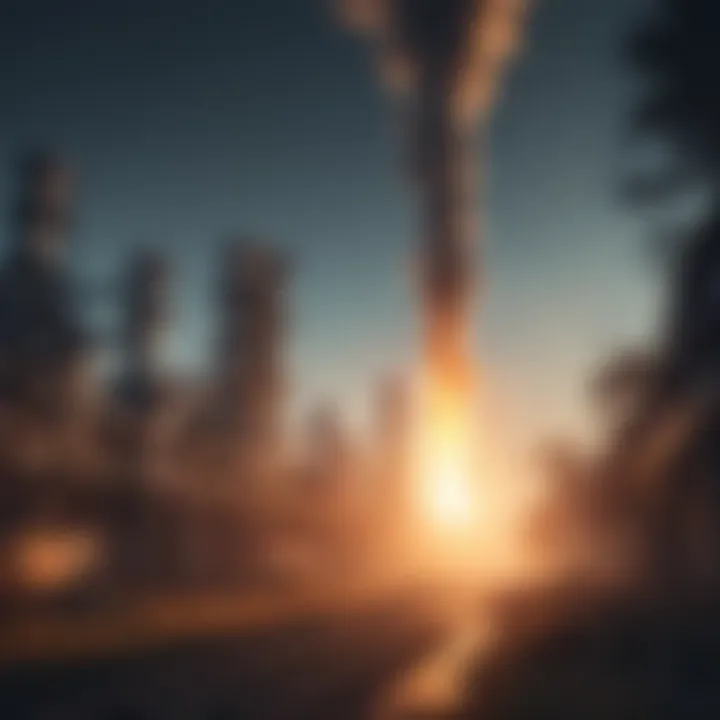
Intro
Carbon capture geoengineering is a critical area of focus in the ongoing battle against climate change. As the impacts of global warming become increasingly severe, exploring innovative techniques to mitigate these effects becomes essential. This introduction sets the stage for an in-depth examination of carbon capture geoengineering, presenting its scientific underpinnings, methodologies, and the broader implications it carries.
The development of carbon capture technologies is driven by the urgent need to reduce atmospheric carbon dioxide levels. With rising temperatures and more frequent climate-related disasters, the conversation around these technologies has gained momentum. The approaches to capturing carbon vary widely, encompassing a range of methods—from direct air capture systems to bioenergy with carbon capture and storage.
The importance of this topic cannot be overstated. In addition to understanding the technological aspects, we must consider the ecological implications, economic outcomes, and ethical dilemmas associated with implementing these solutions. This article aims to navigate these multifaceted dimensions, offering readers a comprehensive perspective on carbon capture geoengineering and its integral role in climate mitigation strategies.
Understanding Carbon Capture Geoengineering
Carbon capture geoengineering represents a critical area of research in addressing climate change. This section outlines its importance, specifically focusing on carbon capture technologies and the broader concept of geoengineering. Understanding these concepts is vital, as they lay the groundwork for potential solutions to mitigate the ongoing effects of climate change.
Defining Carbon Capture
Carbon capture refers to the process of capturing carbon dioxide (CO2) emissions from sources such as power plants and industrial facilities before it enters the atmosphere. The captured CO2 can then be stored underground or utilized in various applications, such as enhancing oil recovery or producing chemicals. This practice is essential for reducing greenhouse gas concentrations, thus playing a crucial role in slowing down global warming.
Current estimates suggest that carbon capture could remove billions of tons of CO2 from the atmosphere each year if implemented effectively. The technology is particularly relevant for industries with hard-to-abate emissions, where alternatives to fossil fuel combustion are scarce. Notably, certain captur technology innovations include amine-based absorption systems and solid sorbents, both designed for efficient and economical CO2 removal.
Geoengineering as a Concept
Geoengineering encompasses a range of methods aimed at deliberately manipulating the Earth's climate system to counteract the effects of climate change. Carbon capture is a subset of geoengineering, which focuses specifically on carbon dioxide removal. The idea is to employ various strategies that can have notable impacts on atmospheric composition and temperature regulation.
Unconventional approaches within geoengineering have generated much discourse among scientists, policymakers, and the public. These methods include solar radiation management and carbon dioxide removal techniques. Each approach has its advantages and drawbacks, influencing how stakeholders view their deployment.
Moreover, ethical, environmental, and social implications are prominent areas of consideration within the geoengineering dialogue. As potential solutions are explored, it is vital to weigh the benefits against the possible risks.
"The advancement of carbon capture technologies is paramount to achieving climate targets and ensuring sustainable development."
In summary, comprehending carbon capture and geoengineering paves the way for informed discussions on climate change solutions. These concepts highlight the need for innovative strategies to mitigate greenhouse gas emissions effectively. As society seeks to transition towards a more sustainable future, it is essential to focus on these pivotal aspects.
The Science of Carbon Capture
Understanding the science behind carbon capture is essential in the discourse surrounding climate change mitigation strategies. The mechanisms involved in carbon capture not only highlight the complexity of the issue at hand but also underscore the significant potential these technologies hold in reducing atmospheric carbon dioxide levels. By delving into the chemical processes and technological innovations that drive carbon capture, this section illuminates the intricacies of carbon capture geoengineering.
Chemical Processes Involved
The chemical processes of carbon capture are diverse, involving various methods to separate carbon dioxide from other gases in the atmosphere. One primary method is chemisorption, where carbon dioxide reacts with certain chemicals to form a solid or liquid compound. This process is implemented in technologies like amine-based carbon capture systems. In these systems, amines bond with carbon dioxide to create a easily separable solution.
Another chemical process is physisorption, which relies on physical forces rather than chemical reactions. Using activated carbon or zeolites, this technique is often employed in direct air capture systems. The efficiency of physisorption can be influenced by temperature and pressure, allowing for adaptability in various environments.
Additionally, mineral carbonation processes carbon dioxide with natural minerals, resulting in stable carbonates that can be safely stored. This not only aids in carbon capture but also provides an effective means of long-term sequestration, making it a notable method in carbon capture geoengineering.
Key Points on Chemical Processes:
- Chemisorption generally offers higher capture rates compared to physisorption.
- Processes can vary significantly based on chemical properties of the capture material.
- Mineral carbonation presents advantages in stability and safety.
- Each method has its own set of benefits and drawbacks in terms of efficiency and cost.
Technological Innovations
Technological advancements in carbon capture have emerged as critical pillars in the ongoing battle against climate change. Breakthroughs in both hardware and software are reshaping how we approach carbon capture solutions. Innovations, such as improved sorbents and membranes, have increased the efficiency of capturing carbon dioxide from various sources.
For instance, direct air capture technologies have become prominent. Companies like Climeworks utilize large facilities that draw air in and use chemical processes to extract carbon dioxide, offering a tangible way to address atmospheric carbon levels. The scalability of such technologies is vital; ongoing research aims to reduce costs and enhance efficiency.
Another significant innovation lies in integrated bioenergy systems. This method couples biomass energy production with carbon capture and storage (BECCS). While generating energy, carbon capture can occur simultaneously, turning a potential environmental liability into a resource. However, the sustainability of biomass sourcing remains a crucial discussion point.
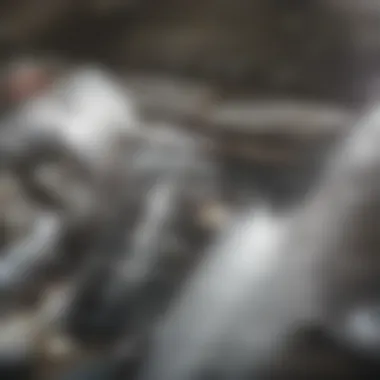
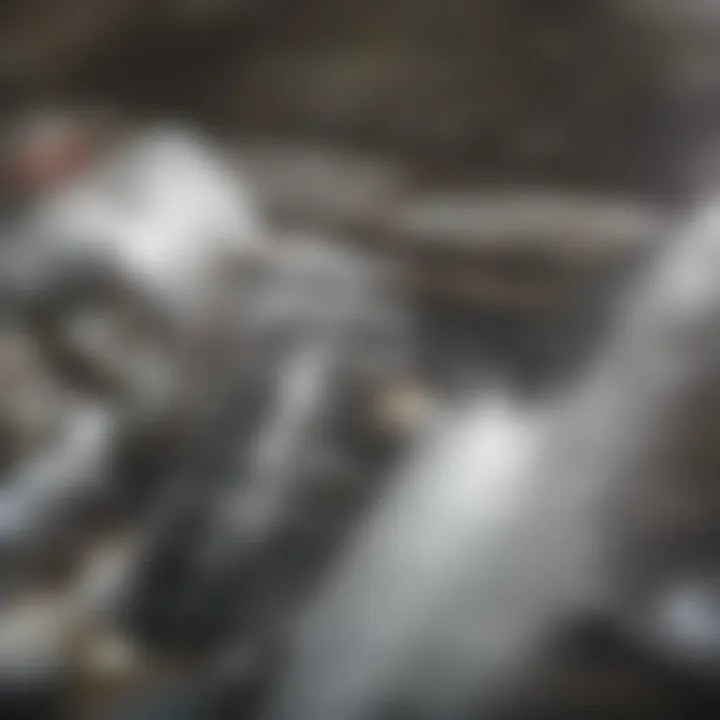
Moreover, monitoring and control technologies are vital. Advanced sensors and data analytics ensure that capture systems operate at optimal levels. This reveals a growing trend in the integration of artificial intelligence and machine learning within the carbon capture context, paving the way for smarter and more responsive systems.
Carbon capture technologies evolve at a rapid pace, driven by both scientific inquiry and market needs. The developments in this field influence practical deployment considerations, including cost and energy consumption.
Methods of Carbon Capture
The topic of Methods of Carbon Capture is critical to understanding how we can effectively combat climate change. Various techniques exist, each with unique attributes and potential benefits. The key lies in recognizing the distinct contributions that these methods can make to reduce atmospheric carbon dioxide levels. These methods can provide pathways to achieve significant long-term reductions in greenhouse gas emissions. Each method deserves attention based on its efficiency, scalability, and ecological consequences.
Direct Air Capture Techniques
Direct Air Capture (DAC) refers to processes designed to remove carbon dioxide directly from the ambient air. Unlike traditional carbon capture, which targets point sources like power plants, DAC can theoretically operate anywhere. The storage of captured CO2 can occur underground or through various utilization methods. The facilities for DAC can be located in regions that have geological formations suitable for CO2 storage.
The importance of DAC lies in its potential for scalability. Facilities can be designed with modular systems, making it easier to scale operations over time. Key players in this field include Climeworks and Carbon Engineering, who employ sorbents or solvents to bind CO2 molecules. This technique emphasizes flexibility and adaptability in addressing the global carbon challenge.
Benefits of DAC:
- Removes CO2 from the air, reducing overall atmospheric concentration.
- Can be combined with sustainable energy sources, minimizing its carbon footprint.
- Offers a chance for negative emissions, which may be necessary to meet global climate goals.
Bioenergy with Carbon Capture and Storage (BECCS)
Bioenergy with Carbon Capture and Storage combines biomass energy production with carbon capture technology. It involves growing plants that absorb CO2, converting these plants into energy, and capturing the released carbon during the energy generation process. By doing this, BECCS can result in negative emissions. This method hinges on sustainable biomass sourcing, ensuring that the environmental benefits are genuine.
The systemic approach of BECCS allows for the utilization of existing energy infrastructure, providing an easier transition to lower-carbon energy systems. However, it is crucial to consider the land-use aspect associated with biomass cultivation, as well as the hydraulic and environmental impacts involved.
Key Considerations for BECCS:
- Sustainability of biomass sourcing and its impact on local ecosystems.
- Integration with existing infrastructure and potential technological advancements.
- Assessment of land-use changes and competition with food production.
Ocean-based Carbon Capture
Ocean-based Carbon Capture exploits the natural capacity of oceans to sequester carbon dioxide. This approach can take various forms. One method is enhancing algal blooms, which absorb CO2 during photosynthesis. Another method involves ocean fertilization where nutrients are added to stimulate growth in phytoplankton, increasing the carbon absorbed.
Additionally, oceanic approaches often consider mineralization, where carbon is reacting with minerals in seawater and forming stable carbonates. The ocean covers over 70% of the Earth's surface. Thus, its role in global carbon management is vast and impactful.
Advantages of Ocean-based Capture:
- Utilizes natural processes, often with minimal energy input.
- Extensive potential for carbon storage due to vast oceanic surface area.
- Enhances marine ecosystems if approached correctly.
Ecological Impacts of Carbon Capture
Understanding the ecological impacts of carbon capture is essential to comprehending the broader implications of geoengineering technologies. As the planet faces the dire consequences of climate change, the role of carbon capture becomes more significant. This section will explore the potential benefits of carbon capture methods and examine the risks and side effects associated with these technologies. This analysis provides a balanced view of how carbon capture can contribute to ecological health, alongside the necessary caution regarding unintended consequences.
Potential Benefits
Carbon capture technologies offer a range of ecological benefits that may support both environmental health and climate mitigation. These benefits include:
- Reduction of Atmospheric CO2: One of the primary advantages of carbon capture is the potential to significantly lower levels of carbon dioxide in the atmosphere, contributing to a decrease in the greenhouse effect.
- Improved Air Quality: By capturing carbon emissions from industrial sites, these technologies can lead to improved air quality in surrounding areas, positively affecting public health and local ecosystems.
- Ecosystem Restoration: Some methods, such as bioenergy with carbon capture and storage (BECCS), not only capture carbon but also allow for reforestation and habitat restoration. This improves biodiversity and supports natural ecosystems.
- Sustainable Resource Management: Carbon capture can lead to more efficient use of fossil fuels. For industries like cement production, carbon capture can mean using resources more sustainably, aligning economic interests with ecological goals.
"The reduction of greenhouse gases through carbon capture presents a dual benefit: combating climate change while improving local environmental conditions."
Risks and Side Effects
Despite the potential benefits, carbon capture technologies are not without risks. Understanding these potential drawbacks is crucial for any comprehensive analysis. Key concerns include:
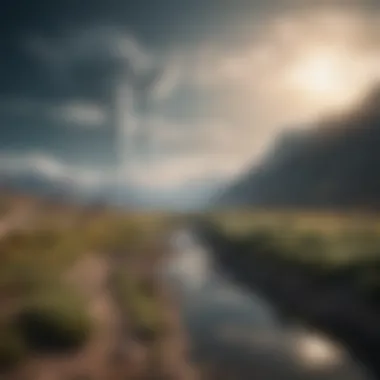
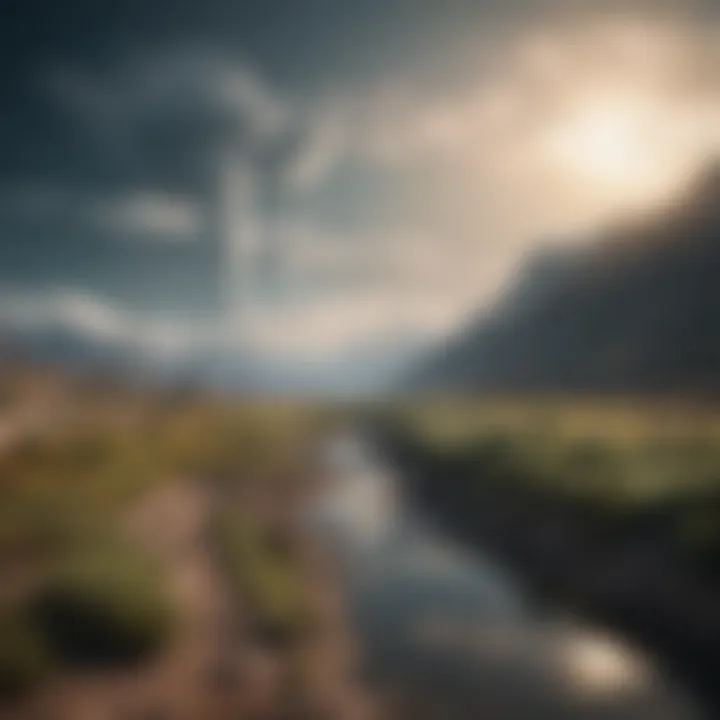
- Environmental Disruption: The implementation of carbon capture technologies can affect local habitats and ecosystems, especially in cases where land is permanently altered for infrastructure.
- Carbon Leakage: If captured carbon is not stored properly, there is a risk of leakage back into the atmosphere. This could undermine the very purpose of the technology and contribute to greater climate change challenges.
- Resource Intensity: The processes involving carbon capture can often be energy-intensive, leading to increased consumption of water and energy resources, which might outweigh some environmental benefits.
- Unsustainable Land Use: The use of bioenergy with carbon capture and storage can lead to unsustainable land practices. If large areas of land are dedicated to bioenergy crops, this can compete with food production and biodiversity.
In considering the ecological impacts of carbon capture, it is important to weigh both potential benefits and risks carefully. This balanced approach helps in forming more informed policy decisions and in promoting responsible implementation of carbon capture technologies.
Socio-economic Considerations
Socio-economic considerations are crucial in the context of carbon capture geoengineering. This section discusses how these technologies will influence economies, industries, and employment. Understanding these aspects helps assess the wider implications of implementing carbon capture strategies. The effectiveness and feasibility of these technologies not only depend on their scientific and ecological attributes but also on their socio-economic viability.
Cost Analysis
Cost analysis in carbon capture geoengineering involves evaluating the financial requirements associated with various technologies. Initial investments in technologies like Direct Air Capture or BECCS can be significant. However, understanding the long-term costs and benefits is essential.
- Investment requirements: The demand for advanced technologies will require substantial initial capital for research, development, and deployment.
- Operational costs: Each method carries unique operational costs over time. Long-term analyses are necessary to gauge the economic sustainability of these technologies.
- Government support: Public funding and incentives can play a critical role in reducing the financial burden on private entities. Policies can create a favorable environment for investment in carbon capture.
"Cost is not merely a factor; it shapes the viability of carbon capture in the global market."
Communities can also benefit indirectly through job creation and industry support that arise from a thriving carbon capture sector. Nevertheless, a comprehensive cost-benefit analysis is essential to gauge the economic impact of such technologies on a global scale.
Impact on Employment and Industries
The implementation of carbon capture geoengineering will undoubtedly affect employment and various sectors. The creation of jobs related to carbon capture technology will offset potential job losses in traditional energy sectors, although the transition may be challenging.
- Job creation: New industries centered on carbon capture and storage technologies are likely to emerge, providing numerous job opportunities. Education and training programs will be necessary to prepare the workforce for these new roles.
- Impact on traditional industries: Some industries may face job reductions as carbon capture technology evolves and the demand for fossil fuels decreases.
Overall, while the transition to carbon capture geoengineering could lead to job displacement in existing energy sectors, it also presents opportunities for economic growth and innovation. The successful integration of these technologies must consider these socio-economic factors to promote a balanced approach to climate change mitigation.
Ethical Debates in Geoengineering
The discussion around carbon capture geoengineering is not solely grounded in its scientific and technological dimensions. It also encompasses crucial ethical debates that question the morality and appropriateness of such interventions in the climate system. Understanding these ethical concerns is essential for anyone engaged in the dialogue about climate change solutions.
At the heart of these debates are several specific elements, including the moral responsibility to future generations, the potential for exploitation, and the implications for justice and equity in global climate efforts. The consequences of carbon capture technologies are not fully known, leading to uncertainties that raise fundamental questions about our right to intervene in natural processes. Ultimately, these debates challenge us to consider not just what we can achieve through geoengineering, but what we should achieve.
Moral Implications
The moral implications of carbon capture geoengineering are profound. One significant concern revolves around the idea of 'playing God.' Many argue that intervening in the climate system alters natural processes in ways that humanity may not understand. Such actions risk unintended environmental consequences, potentially harming ecosystems or communities.
Furthermore, there are ethical considerations related to intergenerational equity. Current decisions about geoengineering could impact future generations. If today’s choices lead to detrimental outcomes, it raises ethical questions about the burden placed on those who did not have a say in those decisions. Is it just to leverage technology that may have unknown effects, thus abdicating our responsibility to those who will inherit this planet?
In addition to these moral questions, there are concerns regarding consent. Many carbon capture technologies may disproportionately affect marginalized communities, who may be less equipped to adapt to changes or who may not have been consulted in the decision-making process. This raises issues of justice, as underprivileged groups might bear the costs of geoengineering efforts while wealthier nations reap the benefits.
Policy and Governance Challenges
Ethical debates also extend into the realm of policy and governance. Developing a regulatory framework for carbon capture geoengineering is inherently complex. Policymakers must balance the potential benefits of technological interventions against the associated risks.
One major challenge in governance is the lack of international consensus on how to manage geoengineering technologies. Different countries have varying capacities, priorities, and ethical standpoints regarding climate interventions. Countries may pursue their geoengineering strategies without adequate cooperation or oversight, leading to conflicts and unintended consequences on a global scale.
Moreover, accountability mechanisms are not clearly defined. If an experiment goes awry due to poor governance, who is responsible? This question highlights the need for robust international agreements that set ethical standards and guidelines for geoengineering practices. Without such agreements, there is a risk of governance gaps where unethical practices may flourish, potentially leading to environmental harm or geopolitical tensions.
"The debate over geoengineering is less about technology and more about the ethical frameworks that underpin our choices."
International Perspectives
The role of international perspectives in carbon capture geoengineering is crucial. Climate change is a global problem, and no single nation can tackle it effectively in isolation. Understanding how different countries engage with carbon capture technologies provides insights into collaborative efforts, policy frameworks, and diverse strategies for implementation. It is important to recognize that solutions may vary greatly based on regional circumstances, technological capability, and socio-economic factors.
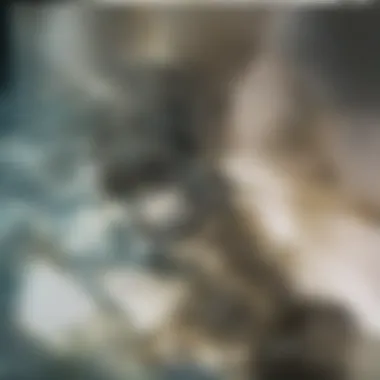
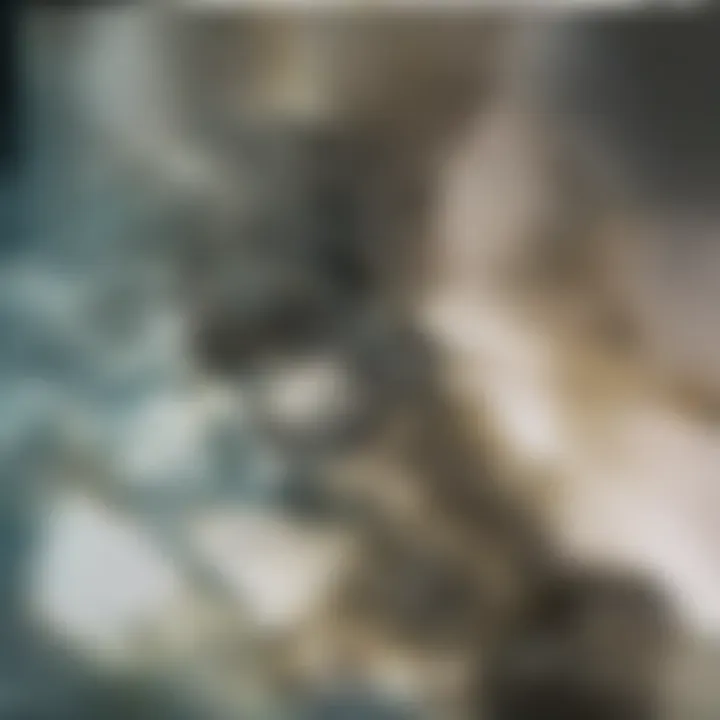
International cooperation can lead to shared technologies and methods. Countries often benefit from collective research efforts, pooling financial resources, and knowledge sharing. Additionally, nations involved in ecologically sensitive areas can align their approaches to minimize environmental impact, thus creating a more effective response to climate change.
Furthermore, international agreements help set standards and promote accountability. They provide frameworks within which countries can operate, ensuring that carbon capture efforts do not become a free-for-all. Clear regulations can help manage risks associated with carbon capture, encouraging responsible practices. This is crucial to gain public support and funding for projects.
Overall, engaging with international perspectives not only enhances the understanding of carbon capture geoengineering but also reinforces the significance of global unity in the fight against climate change.
Global Initiatives and Agreements
Global initiatives and agreements in carbon capture geoengineering exhibit the commitment of multiple nations to address climate challenges. Many international treaties, like the Paris Agreement, now include provisions for carbon capture as a means of achieving emission reduction targets. These agreements create frameworks to incentivize and support research and technology deployment.
Some notable initiatives include:
- The Carbon Capture Utilization and Storage (CCUS) Action Plan: Developed by multiple countries to accelerate the development of technologies that capture and store carbon emissions.
- International Energy Agency (IEA) initiatives: This organization promotes collaborative efforts and shares best practices among member nations to ensure effective deployment of carbon capture technologies.
By engaging in these initiatives, countries can coordinate their efforts, making carbon capture a viable climate solution across borders.
Regional Approaches to Carbon Capture
Regional approaches to carbon capture highlight the diversity of methods based on local conditions. For example, in regions rich in bioenergy resources, Bioenergy with Carbon Capture and Storage (BECCS) might be favored. This method aligns well with agricultural systems, enabling carbon capture while supporting local economies.
Countries with coastal access may develop ocean-based carbon capture strategies. These methods often involve enhancing natural processes that absorb carbon in marine environments. The Great Barrier Reef Restoration efforts, for example, could incorporate carbon capture techniques to improve marine health while addressing climate change.
In urban settings, cities like San Francisco and Stockholm are leveraging advanced Direct Air Capture technologies, tailoring this approach to their specific needs and existing infrastructure. Their experiences can provide valuable lessons for other metropolitan areas seeking effective carbon management solutions.
The Future of Carbon Capture Geoengineering
The future of carbon capture geoengineering is pivotal as the world strives to tackle climate change. As global carbon dioxide levels rise, these technologies present a potential solution to mitigate the severe impacts of climate degradation. Advancements in carbon capture can contribute significantly to reduced atmospheric carbon levels, thus aiding in climate stabilization. Furthermore, understanding the future landscape of these technologies helps in crafting policies that support sustainable, large-scale implementations.
Technological Advancements on the Horizon
Several technological innovations are poised to transform the carbon capture landscape. Research is ongoing in areas such as direct air capture and mineralization of CO2, which may offer more efficient ways of removing carbon from the atmosphere. Some key advancements to watch for include:
- Improved Efficiency: New materials for sorbents and solvents can allow for faster, more efficient capture processes.
- Integration with Renewable Energy: Innovations combining carbon capture systems with energy production from renewable sources, like solar or wind, could enhance overall efficacy and reduce costs.
- Enhanced Monitoring Systems: Better tracking technologies will enable more effective assessments of carbon removal processes and their impacts on the environment.
These developments have the potential to reframe our approach to carbon mitigation efforts, making them more viable in diverse contexts.
Forecasting the Role in Climate Mitigation
Carbon capture geoengineering could play a critical role in the broader climate mitigation strategy. The future use of carbon capture technologies can help achieve several goals:
- Meeting Emission Reduction Targets: Many countries have set ambitious targets for greenhouse gas reductions. Carbon capture can facilitate these goals, especially in hard-to-decarbonize sectors.
- Restoration of Ecosystems: Enhanced carbon removal can alleviate the pressure on natural carbon sinks, aiding in the restoration of forests, wetlands, and oceans.
- Long-term Sustainability: As society progresses, maintaining a balanced carbon cycle will be essential for long-term climate health.
In summary, the future of carbon capture geoengineering hinges on further technological advances and its integration into climate policies. These developments will influence not just environmental outcomes but also reshape social and economic dynamics around energy and resource use.
End
Carbon capture geoengineering stands as a pivotal area of exploration in our fight against climate change. Understanding its nuances is essential not only for grasping the technology but also for appreciating its potential impact on global environmental health. This article has navigated through various aspects of carbon capture, from its scientific foundation to its technological innovations.
Summary of Key Points
This article discussed a range of significant topics related to carbon capture geoengineering. Among these, we examined:
- Defining Carbon Capture: Clarified the core concepts and mechanisms behind capturing carbon dioxide from the atmosphere.
- Technological Innovations: Highlighted recent advancements that enhance the efficiency and reduce the costs associated with carbon capture.
- Methods of Carbon Capture: Assessed various techniques, including direct air capture and bioenergy with carbon capture and storage, illustrating their effectiveness and practicality.
- Ecological Impacts: Analyzed both the potential benefits and the risks that might arise from large-scale deployment of carbon capture technologies.
- Socio-economic Considerations: Scrutinized the financial aspects and implications for employment as industries adapt to these new technologies.
- Ethical Debates: Engaged with the moral dilemmas posed by geoengineering methods and the governance challenges they introduce.
- International Perspectives: Reviewed global initiatives that aim to foster collaboration in addressing climate change through carbon capture.
- Future Insights: Concluded with a look at the role of technological advancements and their future in climate mitigation efforts.
Final Thoughts on Implementation
The implementation of carbon capture geoengineering requires careful consideration. It is not merely a technological solution; it must integrate into broader climate policies. An effective strategy must involve collaboration among governments, industry, and research institutions to address potential ethical concerns and socio-economic impacts.
To ensure successful application, it is essential to promote ongoing research into best practices and ensure transparency in deployment. Continuous monitoring will be needed to evaluate performance and make necessary adjustments. As society navigates this complex landscape, a broad understanding of carbon capture technologies will be crucial in shaping policies that are both effective and equitable.
By fostering an informed dialogue, we can focus on responsibly utilizing carbon capture geoengineering as a tool for climate mitigation while mitigating any unintended consequences.