Understanding Generator Models: A Comprehensive Guide
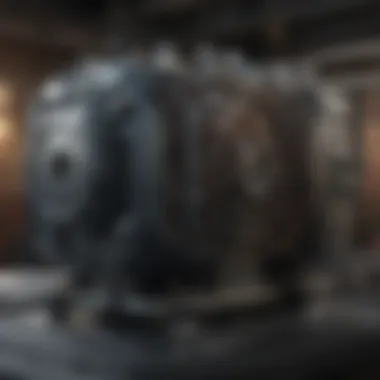
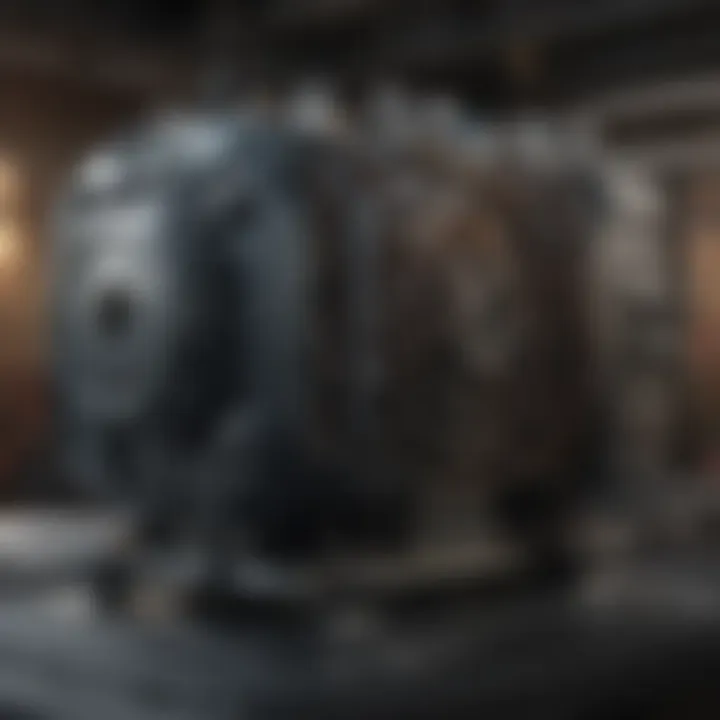
Intro
Generator models play an essential role in electrical engineering, especially in the field of power generation and distribution. A deep understanding of these models is fundamental for both the design and operation of electrical systems. This article aims to unpack the complexities of generator models through an organized approach that emphasizes both theoretical principles and practical applications.
Research Overview
Summary of Key Findings
Generator models can be classified into various types based on their purpose and complexity. Common types include synchronous, asynchronous, and induction generators, each serving different functions in the energy production landscape. Key findings indicate that the choice of generator model significantly affects efficiency, reliability, and overall performance.
The study reveals that mathematical formulations are critical for accurately simulating generator behavior. These formulas account for numerous factors like load conditions, frequency, torque, and phase angle. Understanding these complexities aids engineers in optimizing system performance.
Background and Context
Historically, generator models have evolved alongside technology. The development of electrical machines dates back to the early 19th century. From Thomas Edison's direct current systems to Nikola Tesla's alternating current innovations, the foundations of generator modeling were laid. Today, there is a heightened emphasis on renewable sources like wind and solar, necessitating robust generator models to ensure efficiency in diverse conditions.
Advanced computational methods have also contributed to the evolution of generator modeling, making the process more precise. As a result, educational institutions and professionals are increasingly investing in knowledge surrounding generator behaviors.
Methodology
Experimental Design
To effectively study generator models, strategic experimentation is vital. This typically involves computer simulations that model different generator types under varying conditions. The experiments must simulate real-world scenarios to extract valuable insights about each generator's operational limits and performance metrics.
Data Collection Techniques
Data collection in this realm often incorporates both qualitative and quantitative methods. Simulators create extensive datasets that capture behavior across loads. Additionally, real-world field tests provide empirical data, crucial for validating theoretical models. Collaboration between academic institutions and industry can yield a comprehensive data pool to enhance understanding further.
Ultimately, these findings aim to provide a thorough examination of generator models, paving the way for future innovations.
"Understanding generator models not only empowers engineers but also fuels advancements in electrical technology, driving sustainable practices forward."
In the sections that follow, we will dive deeper into the various types of generator models, mathematical formulations, and their practical applications, ensuring a cohesive narrative throughout the article.
Prelude to Generator Models
Generator models serve as a critical element in the realm of electrical engineering, encapsulating the essence of power generation and distribution. Understanding these models is vital not only for academic scrutiny but also for practical applications in varied sectors, including renewable energy, industrial setups, and power systems management. A comprehensive grasp of generator models facilitates efficient energy conversion, enhances system reliability, and promotes innovation in technology.
Definition and Importance
Generator models are mathematical and conceptual representations of how generators function under specific conditions. They delineate the relationship between electrical output and mechanical input, allowing engineers to simulate performance and predict behavior under varying loads. The importance of generator models lies in their foundational role in power system studies. They enable the analysis of stability, efficiency, and overall system performance. By applying these models, one can optimize generator design and operational parameters, thus improving energy efficiency and reducing losses.
"Generator models are not just theoretical; they are the backbone of modern energy systems, guiding decision-making and system design."
These models contribute significantly to the advancement of technologies aiming for cleaner, more efficient energy. Additionally, they are pivotal in implementing smart grid technologies, where accurate modeling predicts how generators will respond to dynamic changes in demand. Moreover, they help in the allocation of resources, impacting both economic viability and environmental sustainability.
Historical Context
The evolution of generator models mirrors the advancement of electrical engineering itself. Early generators, such as the Faraday disc, laid the groundwork for understanding electromagnetic induction. As time passed, the need for more reliable and efficient generating units necessitated the development of advanced models. In the 20th century, theoretical advancements came about with the formulation of mathematical tools that described generator behavior under diverse operational settings.
With also the integration of digital technology, contemporary modeling techniques emerged, enabling more precise simulations. These modern models account for numerous factors, such as load variations and operational constraints, ensuring realistic portrayals of generator performance. The historical trajectory of generator models highlights a continuous quest for optimization, shaping how societies harness and utilize energy today.
Fundamental Principles of Generators
Understanding the fundamental principles of generators is essential to grasp their operation and significance in the field of electrical engineering. These principles lay the groundwork for the design, function, and application of various generator models. By delving into the core concepts, one can appreciate not only how generators convert mechanical energy into electrical energy but also the factors that influence their efficiency and reliability.
Electromagnetic Induction
Electromagnetic induction forms the heart of generator operation. This principle, discovered by Michael Faraday, states that a change in magnetic flux can induce an electromotive force (EMF) in a conductor. In practical terms, when a conductor moves through a magnetic field, an electrical current is generated. This is critical for both AC and DC generators, as they rely on mechanical motion to drive this process.
The efficiency of electromagnetic induction is influenced by several factors:
- Strength of magnetic field: The stronger the magnetic field, the higher the induced EMF.
- Speed of motion: Faster motion of the conductor through the magnetic field leads to greater induction.
- Orientation of the conductor: The angle at which the magnetic field intersects the conductor affects the amount of induced current.
In generators, the design often incorporates permanent magnets or electromagnets to create a stable magnetic field, enhancing the induction process. This principle is not just theoretical; it has practical applications in everything from small-scale generators used in backup power systems to large residential and industrial power generation.
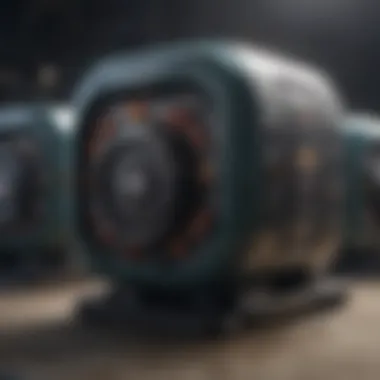
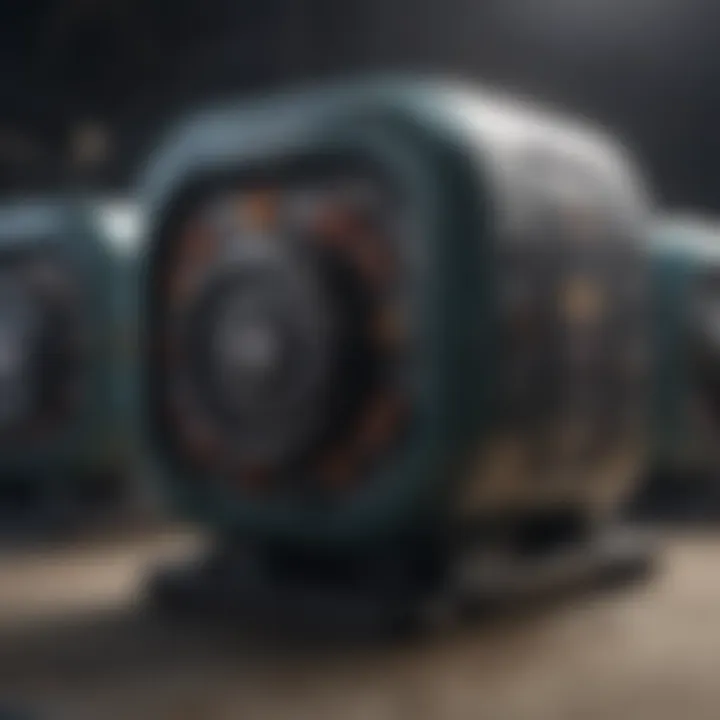
Energy Conversion Mechanism
The energy conversion mechanism in generators is where the transformation of energy types occurs. Generators typically convert mechanical energy, often derived from sources like wind or steam, into electrical energy through electromagnetic induction. This involves several stages:
- Mechanical Input: Energy is supplied to the generator, usually through a rotating shaft that is attached to a turbine.
- Induction of EMF: As discussed earlier, the mechanical motion interacts with the magnetic field, resulting in the generation of electricity.
- Output of Electrical Energy: The generated electrical current is then channeled out of the generator for use in power systems or for consumer applications.
The efficiency of energy conversion is paramount. High efficiency assures that more of the input mechanical energy translates to useful electrical energy. Several design elements are crucial here:
- Material Selection: Using high-quality conductive materials can minimize energy losses.
- Windings Configuration: The layout of windings affects the output and efficiency.
- Load Matching: Properly matching the generator to its load enhances performance and longevity.
"In essence, understanding these fundamental principles is essential for optimizing generator design and functionality across various applications."
These principles not only highlight the mechanics of energy transformation but also underscore the ongoing advancements in generator technology, which aim to enhance efficiency and broaden applications. As such, a firm grasp of these concepts is imperative for anyone engaged in the field or studying generator models.
Types of Generator Models
Understanding the various types of generator models is critical in electrical engineering. These types dictate how energy is generated and converted, influencing not only performance but also applicability in different contexts. By categorizing generators into AC and DC models, we can better grasp their design and operational characteristics. Each category encompasses specific types, each offering distinct features that suit different applications. Therefore, this section elucidates the types of generator models, emphasizing their unique elements and underlying benefits.
AC Generators
AC generators play a significant role in power generation and distribution. They are designed to produce alternating current, which is the primary form of electricity used in homes and industries. Within this category, we can find both synchronous and asynchronous generators.
Synchronous Generators
Synchronous generators are noteworthy for their ability to produce electricity at a constant frequency. This feature is highly valuable as it allows for stable and efficient power supply to the grid. The key characteristic of synchronous generators is their rotor speed, which is synchronized with the frequency of the current produced. As a result, they are a beneficial choice for large-scale power plants, where stability and reliability in electricity supply are paramount.
One unique feature of synchronous generators is the use of excitation systems that provide the necessary magnetic field for energy conversion. These systems enhance operational control and can improve overall efficiency. Nonetheless, synchronous generators demand precise control to maintain synchronism with the grid, which can introduce technical complexity.
Asynchronous Generators
Asynchronous generators, also referred to as induction generators, are distinct in their operations. They do not require synchronization with the power grid, which gives them flexibility in various applications. A key characteristic of asynchronous generators is that they can operate above or below synchronous speed, making them suitable for wind turbines and other dynamic systems.
Their unique feature lies in the simplicity of their design and the reduced need for intricate control systems. This makes them attractive in scenarios like renewable energy systems. However, they often have power quality issues and may require additional equipment to stabilize voltage and frequency.
Generators
DC generators produce direct current, which was widely utilized in the early days of electrical engineering. They remain relevant today, particularly in applications requiring low voltage and adjustable output. DC generators can be classified into separately excited and self-excited types.
Separately Excited
Separately excited generators are known for their precise output voltage control, making them suitable for laboratory and testing environments. The key characteristic of this type lies in the fact that the field winding is powered by an external source. This allows for a wide output voltage range and stability in performance.
The unique aspect of separately excited generators is their sensitivity to load changes, offering quick response times. This adaptability is essential in applications requiring reliable voltage control but also introduces practical disadvantages—such as dependence on external power sources.
Self-Excited
Self-excited generators, in contrast, generate their field excitation from the current produced. This self-sufficient mechanism is a key characteristic that allows these generators to independently operate without needing an external power source. This feature makes them an attractive option for isolated systems or backup power generation.
However, self-excited generators can have limitations in output voltage consistency, particularly under varying load conditions. Their control system complexity is also a consideration, as it may impact performance under dynamic conditions.
The choice between these generator types ultimately depends on the specific application requirements and the context in which they are to be employed.
In summary, comprehensively understanding AC and DC generators—with their specific types and operational characteristics—provides insights into their applications and benefits, setting the foundation for further exploration into mathematical modeling and practical applications in the forthcoming sections.
Mathematical Modeling of Generators
Mathematical modeling of generators serves a critical role in understanding and predicting the behavior of generator systems under various conditions. By employing mathematical frameworks, engineers and researchers can simulate how generators react to changes in input or load. This modeling provides insights into performance, efficiency, and potential issues that may arise during operation. With the increasing complexity of electrical systems and the integration of renewable energy sources, accurate modeling becomes more crucial.
State-Space Representation
State-space representation is a powerful approach for modeling dynamic systems like generators. It encapsulates the system's states and outputs through a set of linear equations. This method enables the representation of systems with multiple inputs and outputs, making it suitable for complex generator setups, particularly in power systems.
The state-space approach allows for the analysis of system stability and response to various inputs. It simplifies the design of control strategies, which is vital for operational efficiency. By focusing on the internal states and their evolution over time, engineers can predict how changes in one component affect the entire system's performance.
Equations Governing Generator Behavior
The equations governing generator behavior form the bedrock of understanding how generators function. These equations describe how electrical energy is converted and controlled within any generator system.
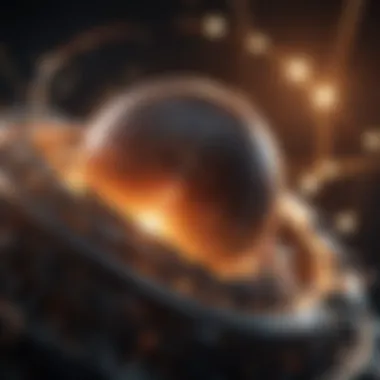
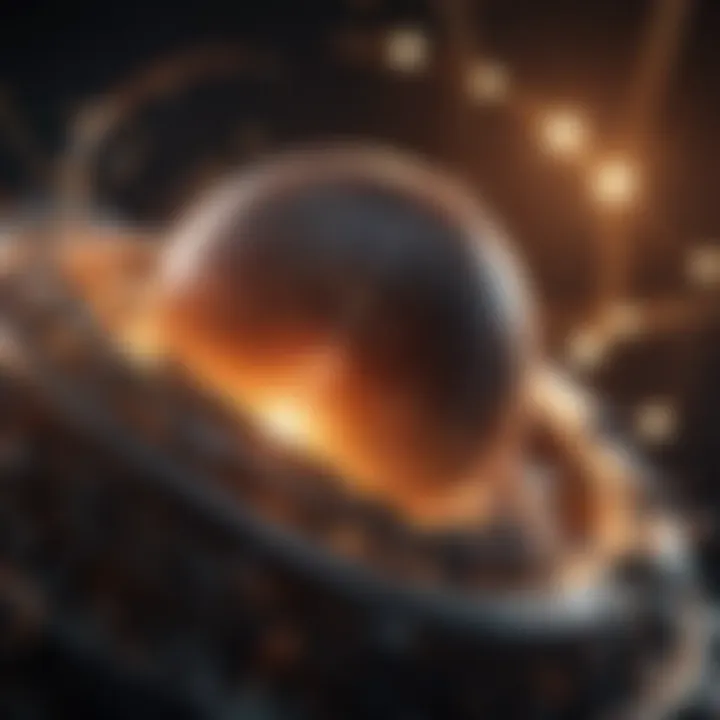
Basic Load Flow Equations
Basic load flow equations are fundamental in power system analysis. They describe energy distribution in electrical networks. These equations are beneficial as they detail how power flows across different components of a system. Their simplicity allows engineers to perform quick calculations to assess system reliability and efficiency rapidly.
One unique feature of basic load flow equations is their ability to handle both AC and DC systems effectively. Their widespread adoption comes from their utility in planning and evaluating the operational performance of power distribution systems. However, while effective for steady-state analysis, these equations may not capture transient dynamics, a limitation that advanced modeling techniques often address.
Dynamic Simulation Equations
Dynamic simulation equations provide a detailed perspective on how generators behave over time, especially under varying operational conditions. These equations consider both the immediate response of the system and its time-dependent characteristics. Their integration is essential for forecasting how generators react during faults or changes in load.
The key characteristic of dynamic simulation equations is their ability to simulate the system's response to disturbances. This feature aids in designing robust control systems. As power systems evolve, dynamic simulation becomes crucial in ensuring reliability and efficiency. However, the complexity required for accurate dynamic modeling can result in increased computational requirements and needs for detailed input data, making it a trade-off between fidelity and resource consumption.
In summary, mathematical modeling, including state-space representation and governing equations, allows engineers to design optimized, scalable, and resilient generator systems.
Applications of Generator Models
The applications of generator models extend far beyond theoretical interests. They play a crucial role in numerous practical fields, primarily affecting our power systems and fostering the growth of renewable energy initiatives. Understanding these applications is essential for professionals, researchers, and students, as they represent the real-world impact and utility of generator models. Here, we will delve into two key areas: power system analysis and renewable energy systems.
Power System Analysis
Generator models serve as a backbone for effective power system analysis. They help to create accurate simulations of power systems, which can greatly inform decision-making processes. Key components include load flow analysis, stability analysis, and contingency analysis.
- Load Flow Analysis: This aspect involves calculating the voltages, currents, and power flows in a network. Accurate models provide insights into how generators respond under different load conditions. This is vital for ensuring stability and reliability in power distribution.
- Stability Analysis: Stability is essential to maintain the continuous operation of power systems. Models help to assess how changes, such as a sudden generator failure or fluctuation in load demand, affect overall system stability. This understanding is crucial in designing resilient power systems.
"Generator models are integral in evaluating how electrical systems behave under various scenarios, supporting the planning and operation of power networks."
The insights gained from power system analysis can lead to better management strategies and mitigation measures. This not only increases the efficiency of energy production but also enhances the security of the energy supply. Additionally, they provide a platform for training future engineers, allowing for hands-on experience with dynamic system behavior.
Renewable Energy Systems
With the rising focus on sustainability, generator models are becoming increasingly important in the context of renewable energy systems. The integration of solar, wind, and other renewable sources into traditional power grids presents unique challenges that necessitate sophisticated modeling solutions.
- Grid Integration: The output from renewable sources like solar panels and wind turbines is often variable. Generator models help in predicting and managing these variations, enabling smoother integration into the grid. This is essential for reducing reliance on fossil fuels and transitioning to cleaner energy sources.
- Performance Optimization: By employing generator models, we can assess the efficiency of various renewable energy systems. For instance, models can simulate how wind patterns affect turbine output or how solar irradiance influences panel performance. This data is crucial for optimizing energy production and reducing costs.
Overall, the application of generator models in renewable energy systems not only supports the transition towards greener alternatives but also enhances the operational efficiency of these new technologies. As professionals and researchers work towards a sustainable energy future, an understanding of these applications remains vital.
Advantages of Generator Models
Generator models hold a significant position in the field of electrical engineering, primarily due to their crucial role in the efficient production and management of electrical energy. Understanding their advantages can greatly enhance our approach toward integrating these systems into wider applications. As power demands grow, generator models provide innovative solutions to meet these needs while minimizing resource wastage. The following are key aspects of the advantages associated with these models.
Efficiency in Energy Production
Generator models are designed to optimize energy production through various mechanisms. Efficiency is measured based on how effectively a generator converts mechanical energy into electrical energy. Various factors contribute to achieving high efficiency, including the internal components of the generator, the design of the system, and the operational conditions under which it functions.
Some important factors to consider include:
- Design Optimization: Generators are often tailored in design to fit specific applications, ensuring that they function at their optimal capacity.
- Load Management: Smart generator models integrate load management algorithms that optimize the usage of generated electrical energy. This leads to lower operational costs while maximizing performance.
- Use of Advanced Materials: Modern generators utilize high-performance materials which enhance not only efficiency but also the longevity of the components.
These efficiencies translate directly into cost savings and reduced environmental impact, making generator models not only practical but also indispensable in today's energy landscape.
Scalability and Adaptability
Another critical advantage of generator models is their scalability and adaptability. These models can be modified or expanded in response to changing energy needs without sacrificing performance. This quality supports both large industrial facilities and small scale operations, proving versatile across diverse sectors.
Key points include:
- Modular Design: The modular aspect allows for easy upgrades and expansions. For instance, if power output needs increase, additional modules can be added without redesigning the entire system.
- Application Versatility: Generator models are used in various settings ranging from renewable energy projects to conventional power plants. This flexibility is essential as it allows operators to pivot based on available resources and energy demands.
- Smart Integration: With the rise of smart technologies, generator models are compatible with IoT devices that monitor performance in real-time. This real-time data can lead to quick adaptations to changing operational conditions.
"Understanding the benefits of generator models enables industries to make informed decisions to optimize energy production and sustainability standards."
By emphasizing efficiency as well as scalability, organizations can not only enhance their production capabilities but also contribute to a more sustainable future.
Disadvantages and Challenges
In understanding generator models, it is not only essential to explore their various advantages but also to critically assess their disadvantages and challenges. This section aims to deliberate on specific elements that hinder the full application and effectiveness of generator models within the field of electrical engineering. Identifying these drawbacks brings clarity to decision-making and may assist researchers and practitioners in innovating solutions to these pressing issues.
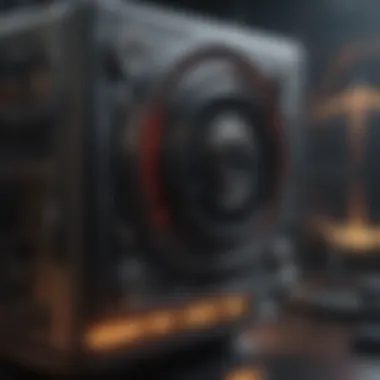
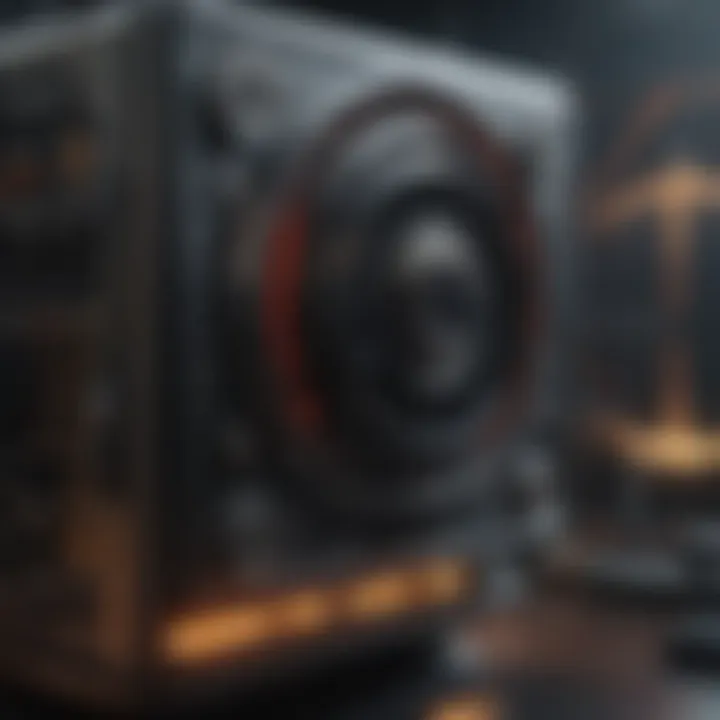
Technical Complexity
The technical complexity of generator models can pose significant obstacles for users. These models often require a strong background in electrical engineering principles and mathematics for accurate interpretation and implementation. For novice users, the steep learning curve may result in misapplications or misinterpretations of the generator behavior.
Moreover, the intricacies involved in the mathematical modeling of generator functions can lead to errors if not properly understood. Here are some aspects of this complexity:
- Advanced Mathematics: The use of differential equations, Laplace transforms, and numerical analysis can be daunting for individuals with insufficient training in these areas.
- Model Calibration: Ensuring that the model accurately reflects real-world behavior often necessitates extensive calibration, which requires both time and expertise.
- Technology Integration: As technology evolves, integrating these models with new systems, like smart grids, can introduce additional layers of complexity.
This level of sophistication is not inherently negative; however, it does necessitate thorough training and awareness of the limitations found within various models. Emphasizing ongoing education and development in this area is key to overcoming these barriers in practical applications.
Cost Considerations
Cost considerations are another crucial aspect when examining the challenges associated with generator models. The expenses incurred can limit the feasibility of implementing sophisticated generator systems in various projects, especially for startups and smaller enterprises. The following points detail these financial implications:
- Initial Investment: High-quality generator models can entail significant upfront costs. From software licenses to hardware for simulation purposes, these initial expenditures can deter newcomers.
- Maintenance and Upgrading: Operating and maintaining complex generator models often involves ongoing costs, including necessary updates and periodic recalibration to ensure accuracy.
- Training Costs: To effectively utilize generator models, organizations may need to invest in training personnel. These training programs can be expensive and time-consuming, further straining budgets.
In summary, while generator models offer various advantages, their complexity and cost are not to be overlooked. Engaging with these issues is essential for a realistic understanding of their practicality in real-world applications.
Given the daunting nature of these challenges, continuous exploration into more intuitive modeling approaches and cost-effective solutions is vital for enhancing accessibility and lowering barriers to entry in generator technology.
Future Trends in Generator Modeling
The field of generator modeling is continuously evolving. As electrical engineering integrates more with digital technologies, understanding the future trends in generator modeling becomes crucial. This exploration isn't just academic; it offers practical benefits for efficiency, reliability, and sustainability in power systems. Upcoming advancements can directly influence important aspects, making generators more responsive and adaptable in varying conditions.
Advancements in Simulation Technologies
Simulation technologies play a vital role in the future of generator modeling. They provide a platform to analyze and predict generator performance under different scenarios without the need for physical prototypes. With increased computational power and sophisticated algorithms, simulations can delve deeper into the dynamics of generator systems.
Key benefits of these advancements include:
- Enhanced Accuracy: Modern simulations can model complex behaviors, leading to more precise results.
- Real-Time Analysis: Simulating systems in real-time can help engineers make immediate decisions, improving response times.
- Cost Reduction: By predicting issues before implementation, companies can save on resources and reduce waste.
Moreover, simulation tools have become more user-friendly. Software like MATLAB and Simulink allows for interactive modeling, making it accessible for both students and professionals. Enhanced visualization tools help in better interpretations of data, thereby improving overall system design and analysis.
Integration with Smart Grid Technologies
The integration of generator modeling with smart grid technologies is not just a trend but a necessity for modern power systems. Smart grids utilize modern communication tools and information technology to optimize the delivery of electricity. When combined with advanced generator models, intelligent algorithms can facilitate better management of electricity generation.
Considerations for this integration include:
- Demand Response: Generators can adjust output based on real-time demand, improving efficiency.
- Renewable Resource Integration: It allows seamless integration of renewable energy sources like solar and wind, which are highly variable.
- Enhanced Grid Stability: With accurate and timely data from generators, smart grids can better manage fluctuations in power supply and demand, enhancing stability.
In summary, the collaboration between generator modeling and smart grid technology presents a more effective approach to energy management. This integration not only enhances operational efficiency but also furthers the goal of sustainability in energy production.
"Future trends in generator modeling stand to redefine our approach to energy management, ensuring both efficiency and sustainability in an evolving landscape."
As these trends evolve, they will shape the engineering practices of tomorrow, helping both students and professionals navigate the new technological landscape.
Finale
The conclusion of the article serves as a pivotal segment, synthesizing the complex narratives that unfold within the extensive discussion of generator models. This section not only encapsulates the key ideas presented but also sheds light on their overarching significance in the field of electrical engineering. Understanding these models is essential for students, researchers, educators, and professionals alike, as they are integral in the advancement of technology and energy solutions.
It is important to recognize that generator models are more than academic constructs. They play a critical role in power systems, influencing efficiency and adaptability. By reviewing the various types of models, their advantages, and challenges, readers are equipped with knowledge that can be applied practically, thereby facilitating better decision-making and innovation in engineering practices.
Moreover, this concluding segment emphasizes the relevance of staying attuned to the future trends delineated in earlier sections. The landscape of energy is rapidly shifting with advancements in technology, and generator models will continue to evolve in response to these changes. The integration with smart grid technologies is particularly noteworthy, as it underscores a commitment to enhancing reliability and sustainability in energy production.
"The future of energy relies on our ability to adapt and innovate with the tools we have, including generator models that shape the landscape of power generation and distribution."
In summary, the conclusion distills the essence of the discourse, highlighting that deeper exploration of generator models is both a necessity and a pathway for future development in energy engineering.
Summary of Key Points
This section reaffirms the core insights from the article regarding generator models. Below are the key points:
- Definition and Importance: Generator models are essential for understanding energy systems.
- Fundamental Principles: Concepts like electromagnetic induction form the basis of generator functionality.
- Types of Models: AC and DC generators are two primary forms, each with unique characteristics and applications.
- Mathematical Modeling: State-space representations and governing equations are crucial for simulating behavior.
- Applications: These models find use in power system analysis and renewable energy systems.
- Advantages and Disadvantages: While efficiency and scalability are benefits, technical complexity and costs present challenges.
- Future Trends: Advancements in simulation and integration with smart grid technologies will dictate the future of generator models.
Implications for Future Research
The implications for future research are manifold, inviting scholars and professionals to delve deeper into unexplored territories within generator modeling. Areas to consider include:
- Enhanced Simulation Techniques: Research can focus on developing more sophisticated simulation methodologies that account for diverse operational scenarios.
- Energy Storage Integration: Future models could examine how to best incorporate energy storage solutions, enhancing flexibility and reliability in power systems.
- Sustainability Assessments: Investigating the environmental impact of various generator models may yield insights that promote greener technologies.
- Smart Grid Compatibility: Research aimed at understanding how generator models can evolve to align with emerging smart grid frameworks will be essential for future innovations.
- Interdisciplinary Approaches: Collaborations across engineering disciplines can yield novel insights, leading to breakthroughs in generator model efficacy and application.
Effective research in these areas can significantly contribute to the field's knowledge base and lead to innovations that improve energy systems worldwide.