Protocols for CRISPR-Cas9: A Comprehensive Guide
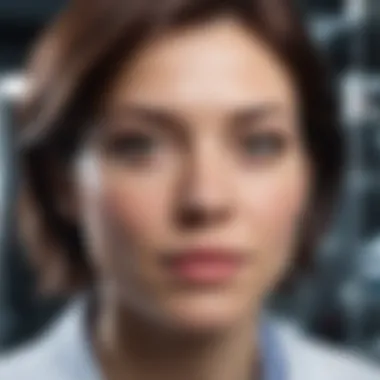
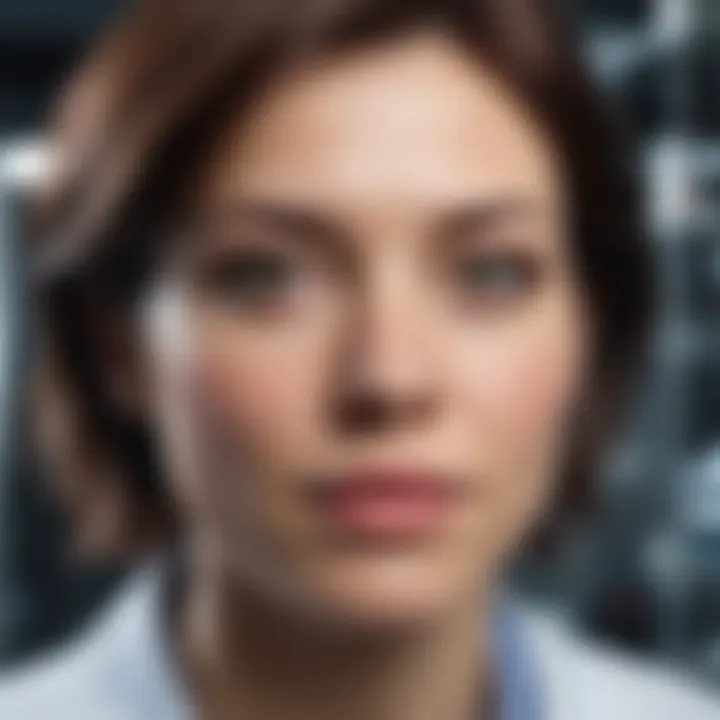
Intro
CRISPR-Cas9 technology represents a revolutionary advancement in the field of genetic engineering. It allows for precise modifications to DNA sequences, offering promising applications in medicine, agriculture, and biological research. Understanding the protocols involved in utilizing this powerful technology is essential for both students and professionals aiming to harness its full potential. This article provides a thorough examination of the methodologies, emphasizing various stages from design to implementation.
Research Overview
Summary of Key Findings
The exploration of CRISPR-Cas9 protocols reveals several key factors that significantly influence the success of gene editing. These factors include the accuracy of target selection, the efficiency of delivery methods, and rigorous validation processes. Understanding these components helps researchers design effective experiments that yield reliable results.
Background and Context
Gene editing has evolved dramatically over the years, but CRISPR-Cas9 stands out due to its simplicity and efficiency. Originating from a naturally occurring defense mechanism in bacteria, this technology was adapted for use in molecular biology, enabling a level of precision previously thought to be unattainable. The increasing popularity of CRISPR-Cas9 is evidenced by the rapid growth of related research and its applications in various fields, including therapeutic development and agricultural improvements.
Methodology
Experimental Design
An effective experimental design is fundamental in CRISPR research. This involves several critical steps:
- Identifying the specific gene or genomic region targeted for modification.
- Selecting appropriate guide RNA sequences that match the target DNA.
- Choosing a delivery system, such as plasmids or viral vectors, to introduce the CRISPR components into target cells.
By carefully considering these elements, researchers can optimize their experiments to achieve the desired genetic modifications.
Data Collection Techniques
Data collection is vital in assessing the efficacy of CRISPR-Cas9 applications. Common techniques include:
- DNA sequencing: To confirm successful edits.
- Polymerase Chain Reaction (PCR): For amplifying DNA regions of interest.
- Western blot analysis: To evaluate protein expression levels following gene editing.
Utilizing these methods enables researchers to gather clear, quantitative data, ultimately improving the reliability of their findings.
"The integration of effective data collection techniques is as crucial as the gene editing process itself."
Understanding the protocols associated with CRISPR-Cas9 technology equips researchers with the tools necessary to navigate the complexities of gene editing while ensuring precision and accuracy in their work.
Prelude to CRISPR-Cas9 Technology
The CRISPR-Cas9 technology represents a groundbreaking innovation in the field of gene editing. Understanding this technology is essential for those engaged in biological sciences, genetics, and biotechnology. The ability to edit genes with precision enables researchers to explore genetic functions, correct genetic disorders, and even enhance agricultural crops. This section will provide crucial insight into the underlying principles that govern CRISPR-Cas9, paving the way for comprehension of its applications and implications.
Historical Background
CRISPR, short for Clustered Regularly Interspaced Short Palindromic Repeats, has its origins in a natural defense mechanism found in bacteria. This system was first described in 1987, but it wasn’t until later studies that its potential for gene editing was recognized. In 2012, a landmark paper by Jennifer Doudna and Emmanuelle Charpentier demonstrated that the CRISPR-Cas9 system could be engineered to target specific DNA sequences in eukaryotic cells. This seminal work set in motion a series of developments that made CRISPR technology widely accessible and applicable for various scientific inquiries.
The evolution of CRISPR-Cas9 reflects a confluence of interdisciplinary research efforts. Each advancement in its understanding opened new avenues for application across genetics, microbiology, and medicine. Researchers could now manipulate genes in ways that were previously difficult or even impossible, enhancing the capacity to innovate in diverse fields.
Mechanism of Action
The mechanism of CRISPR-Cas9 is both elegant and efficient. The system relies on two primary components: the Cas9 protein and the guide RNA (gRNA). The guide RNA is designed to match a specific target sequence in the genome. Once introduced into the target cell, Cas9 uses the gRNA to locate the desired DNA sequence with high specificity. The Cas9 protein then cuts the DNA at that location, leading to a double-strand break.
Following this break, the cell’s natural repair mechanisms are activated. The repair process can lead to one of two outcomes. It can result in non-homologous end joining (NHEJ), which often introduces small insertions or deletions at the cut site, potentially disrupting the target gene. Alternatively, if a repair template is provided, homology-directed repair (HDR) can occur, allowing for precise modifications, such as gene correcting or inserting a new gene.
In summary, the CRISPR-Cas9 system harnesses the existing cellular machinery for gene editing, offering a straightforward yet robust method for genomic modification. This has significant implications not only for basic research but also for therapeutic applications in medical science.
"CRISPR-Cas9 redefines our approach to genetic alteration, offering simplicity and precision that was not possible before."
Understanding the historical background and mechanism of action of CRISPR-Cas9 is foundational for users aiming to design protocols and implement gene editing in their own research endeavors.
Overview of Protocol Development
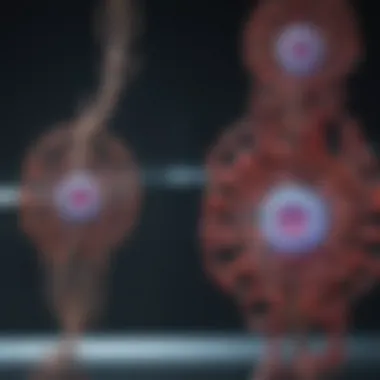
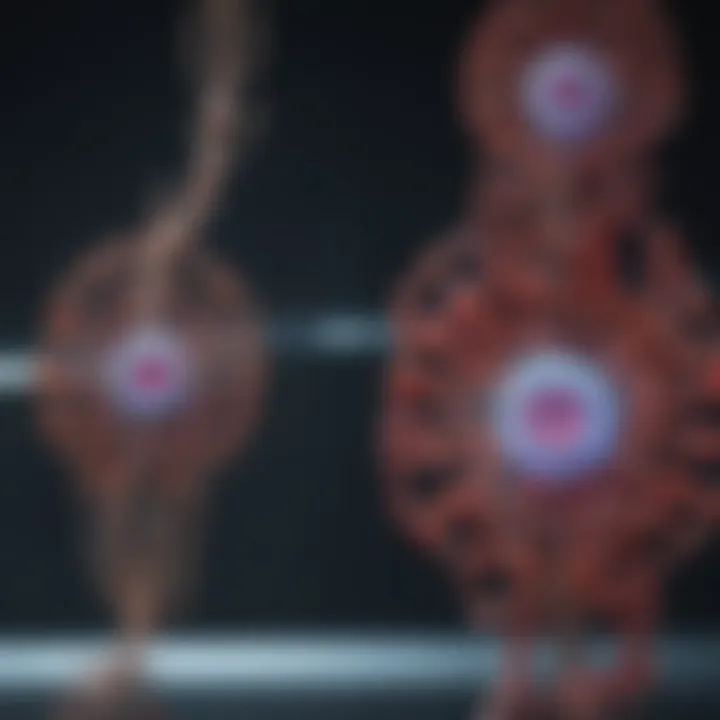
The development of protocols in CRISPR-Cas9 gene editing is pivotal. These protocols serve as a blueprint for researchers, guiding them through the complexities of gene manipulation. A well-defined protocol enhances reproducibility and ensures that experiments yield reliable results. Properly structured protocols can also facilitate collaboration among researchers by providing a clear methodology to follow.
When developing a CRISPR protocol, several critical components must be considered. It is essential to understand how these components interact and the role they play in achieving successful gene editing. Each protocol must address specific aspects such as the type of vector, guide RNA (gRNA) design, and transfection methods that best suit the organism being studied.
Furthermore, considerations for protocol design should include factors like the target site within the genome, the delivery methods for the CRISPR components, and the timing of each step in the process. Each of these elements can significantly impact the success rates of gene editing attempts.
In addition, examining previously established protocols offers insight into effective strategies and frequent challenges. Learning from existing methods allows researchers to avoid pitfalls, thereby increasing the chances of successful experimentation. Overall, investing time in developing comprehensive protocols is a critical step toward advancing research in CRISPR technology.
Key Components of CRISPR-Cas9 Systems
Understanding the key components of CRISPR-Cas9 systems is fundamental for any experimental design. The primary elements include the cas9 nuclease, a guide RNA, and the DNA target sequence.
- Cas9 Nuclease: This enzyme plays the central role in the editing process. It makes double-strand breaks at specific locations in the target DNA, which facilitates the gene editing process.
- Guide RNA (gRNA): The gRNA is crucial for directing the Cas9 enzyme to the correct genomic location. A well-designed gRNA is essential for ensuring that the right DNA sequences are targeted. This component determines the specificity of the gene editing outcome.
- Target DNA Sequence: This is the specific section of the genome that is to be edited. The choice of target site is critical as it significantly impacts the efficiency and accuracy of the CRISPR system.
These three components work together harmoniously to realize effective gene editing.
Considerations for Protocol Design
When embarking on protocol design for CRISPR-Cas9 experiments, several considerations must guide the process.
- Target site: The selection of the target site within the genome must be made carefully. Factors such as accessibility, presence of PAM sequences, and gene function should all influence this choice.
- Delivery Method: Choosing the right delivery method for the CRISPR components is vital. Different methodologies exist; these include plasmid-based, viral vector, or ribonucleoprotein delivery methods. Each has its advantages and limitations, depending on the cell type and specific research goals.
- Validation Techniques: After editing, confirming that the intended modifications are present is important. This can involve sequencing the edited region or using specific assays to measure gene expression.
Additionally, it is essential to address safety and ethical considerations during protocol design. Compliance with relevant biosafety regulations ensures that the experiments are conducted responsibly and ethically.
By thoroughly reflecting on these dimensions, researchers can develop robust protocols that enhance the success rates of CRISPR-Cas9 experiments.
Vector Construction for CRISPR-Cas9
Vector construction is a crucial component of the CRISPR-Cas9 gene-editing process. The vector acts as a delivery system for the components necessary for genome modification, such as the Cas9 endonuclease and the guide RNA (gRNA). An appropriate vector ensures that these elements are successfully introduced into the target cells. This section delves into the considerations and techniques involved in constructing vectors for optimal results in gene editing.
Choosing the Right Vector
Choosing the right vector can significantly influence the efficiency and specificity of CRISPR-Cas9 applications. The choice is often dictated by the type of target organism, the desired editing outcome, and the method of delivery. Here are several factors to consider:
- Type of vector: There are several types of vectors available, including plasmids, viral vectors, or transposons. Each has its advantages and specific applications.
- Promoter selection: A strong promoter is critical for ensuring adequate expression of gRNA and Cas9. The choice of promoter can affect the regulation and level of gene editing.
- Size limitations: Some delivery methods may restrict the size of the vector. Ensure the vector can accommodate both Cas9 and gRNA while retaining functionality.
- Target cell type: Some vectors perform better in specific cell types. It is important to select vectors compatible with the target organism's cellular environment.
In summary, selecting the appropriate vector involves balancing these factors according to the research goals and the specific context of the experiment.
Cloning Techniques
Cloning techniques are essential for the construction of effective CRISPR-Cas9 vectors. These methods allow for the insertion of desired sequences into a vector backbone. Here are some commonly used techniques:
- Restriction Enzyme Cloning: This technique uses specific enzymes to cut DNA at known sequences, allowing for the insertion of the gRNA sequence into the vector. This is a traditional approach, effective for well-characterized sequences.
- Gibson Assembly: While notably versatile, Gibson assembly allows for the seamless joining of multiple fragments of DNA without the use of restriction enzymes. This method can save time and increase cloning efficiency.
- Golden Gate Cloning: This method combines the use of restriction enzymes with a ligation reaction. It offers a quick and efficient way to construct vectors by enabling modular assembly.
- PCR Amplification: Standard polymerase chain reaction can be used to amplify the DNA sequence of interest before ligation or further processing. This technique is helpful for generating gRNA sequences rapidly.
Utilizing these cloning techniques will ensure the successful and accurate construction of vectors for CRISPR-Cas9 applications.
Guide RNA Design and Synthesis
The design and synthesis of guide RNA (gRNA) are pivotal steps in CRISPR-Cas9 gene-editing protocols. The efficacy of CRISPR-Cas9 relies heavily on the precise design of the gRNA, which directs the Cas9 enzyme to the intended target site within the genomic DNA. When constructed correctly, the gRNA not only enhances the specificity of the gene-editing process but also minimizes off-target effects, which is crucial for maintaining genomic integrity.
Target Site Selection Criteria
Selecting the appropriate target site is a fundamental responsibility when designing a gRNA. Key criteria to consider include:
- PAM Sequence: The protospacer adjacent motif (PAM) is essential for Cas9 binding. Commonly, the sequences recognized by popular Cas9 variants such as SpCas9 require a PAM of "NGG" directly following the target site. Without this, Cas9 will not cleave the DNA effectively.
- Specificity: The gRNA should be designed to match the target sequence precisely to increase specificity. Any mismatches can lead to off-target effects. A careful evaluation of potential off-targets using bioinformatics tools can aid in selecting the right target site.
- Accessibility: The DNA region should be accessible, free from tightly bound proteins or structural barriers that could impede Cas9's access to the DNA.
- Positioning: Consideration of the gRNA’s position within the gene is important. Targeting early exons may be more effective than targeting introns, as this often results in a complete knockout of the gene.
Synthesis Methods for gRNAs
The synthesis of gRNAs can be accomplished through various established methods, each with unique advantages:
- In Vitro Transcription: This method utilizes DNA templates to produce RNA via RNA polymerase. It is a commonly used approach due to its scalability and efficiency in generating large quantities of gRNA.
- Chemical Synthesis: For shorter gRNAs, chemical synthesis can be a more straightforward approach. It allows for precise control over the sequence and modifications that can enhance stability and binding affinity.
- Cloning and Plasmid-Based Systems: Some researchers opt to clone the gRNA sequence into plasmids containing transcriptional elements. This allows for the generation of gRNAs within live cells, providing a self-contained system for inducing gene edits.
- Combined Methods: For certain applications, researchers may use a combination of these methods, such as synthesizing a short gRNA that is then incorporated into a plasmid to create a longer, more stable form for in vivo studies.
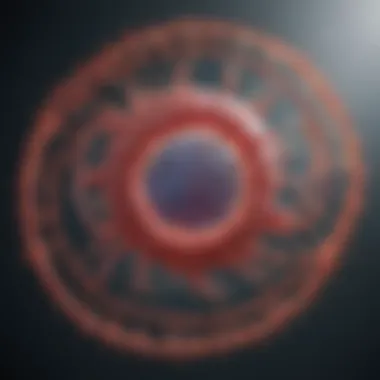
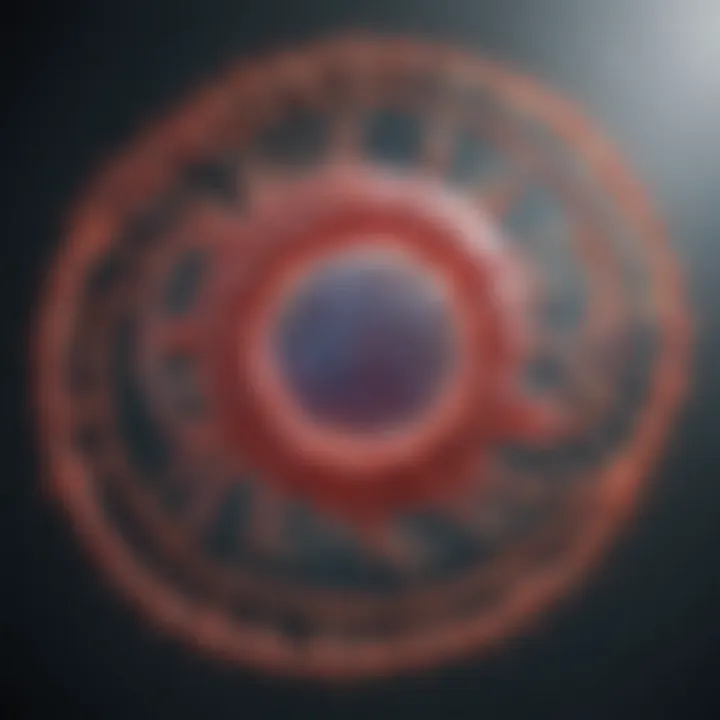
"The success of CRISPR-Cas9 gene editing hinges on the precision of gRNA design and synthesis, which are critical determinants of experimental outcomes."
Transfection Protocols
Transfection protocols are crucial for the success of CRISPR-Cas9 gene editing. This section explores the significance of transfection, delving into specific elements and considerations that impact the efficiency of introducing CRISPR components into target cells. Understanding the nuances of transfection methods enables researchers to optimize their workflows effectively, leading to enhanced editing outcomes.
Methods for Effective Delivery
Effective delivery of CRISPR components, including the Cas9 protein and guide RNA, is essential. Various methods exist for transfecting these molecules into cells.
- Lipofection: This method utilizes liposomes to facilitate the uptake of nucleic acids. It is popular due to its simplicity and efficiency with many cell types.
- Electroporation: In this technique, electrical pulses create temporary pores in cell membranes, allowing the entry of CRISPR components. It can be particularly effective for hard-to-transfect cell types.
- Viral Vectors: Lentiviruses and AAVs can mediate the delivery of CRISPR elements. They have a high transduction efficiency and can integrate into the host genome, providing long-term expression of the CRISPR system.
- Microinjection: This method directly injects CRISPR components into the nucleus of cells. It is labor-intensive but allows for precise delivery, particularly useful in zygote editing.
When selecting a delivery method, consider the following:
- The specific cell type you are working with.
- The intended duration of expression of the CRISPR system.
- The desired efficiency and specificity of the editing process.
Analyzing Transfection Efficiency
After transfection, it is critical to assess the efficiency of the process. Various methods can determine how many cells successfully incorporated the CRISPR components:
- Fluorescent Markers: Using fluorescently tagged gRNAs or Cas9 can help visualize which cells have incorporated these elements.
- PCR Analysis: Polymerase chain reaction can confirm the presence of edits in the target genomic region. Specific primers can amplify the modified genes, indicating successful transfection.
- Sequencing: Next-generation sequencing provides a detailed assessment of editing outcomes, revealing potential off-target effects and confirming successful modifications.
Effective analysis of transfection efficiency not only informs the success of CRISPR applications but also aids in iterative improvements of the protocols.
By systematically investigating transfection methods and their efficiencies, researchers can refine their protocols, ensuring that CRISPR-Cas9 remains a versatile tool for gene editing.
Genomic Integration and Editing Verification
Genomic integration and editing verification are crucial steps in the CRISPR-Cas9 workflow. Once gene editing has been performed, it is essential to confirm that the desired modifications have been integrated into the genome precisely as intended. This not only validates the success of the gene editing endeavor but also ensures that any off-target effects or unintended changes have not occurred, which could lead to undesirable consequences.
The benefits of careful genomic integration verification are substantial. First, it provides confidence in the experimental results. This is especially important in research settings, where findings can lead to further studies or even clinical applications. Second, it safeguards against potential ethical concerns. With gene editing technologies gaining prominence, demonstrating accountability and accuracy in genetic modifications is vital for public trust and regulatory compliance.
When considering genomic integration verification, researchers must pay attention to several elements. These include the location of the edit, the nature of the modification, and the overall stability of the genetic alterations. A thorough assessment includes both qualitative and quantitative analyses, employing various methodologies such as sequencing and PCR (Polymerase Chain Reaction).
Assessing Editing Outcomes
To assess editing outcomes, various approaches can be utilized. Sanger sequencing is often used to confirm the presence of the desired mutations. It offers high accuracy and is particularly effective for small edits within a specific region. Additionally, Next-Generation Sequencing (NGS) provides a more comprehensive view. This method allows the evaluation of several targets simultaneously, making it ideal for larger studies.
Researchers might also rely on T7 endonuclease assay, which detects mismatches in DNA. This technique can quickly indicate whether a successful edit has occurred at specified target sites.
Tools for Validation
Validation is not complete without using tailored tools that enhance the accuracy of results. Here are some common tools used in verifying CRISPR edits:
- CRISPR-specific primers: These primers target the regions adjacent to the cut site to ascertain whether the desired alteration is present.
- Sequencing software: Tools like BLAST (Basic Local Alignment Search Tool) allow for the comparison of sequence data, ensuring that the edits match expectations.
- High-Resolution Melting (HRM) analysis: This method distinguishes between wild-type and edited sequences based on the melting behavior of DNA.
By employing a combination of these methods, researchers can strengthen their validation processes, ensuring that the outcomes of their gene editing experiments are sound and reliable.
"Thorough validation is imperative to uphold the integrity of CRISPR research, confirming both efficacy and safety in applications."
Safety and Ethical Considerations
The integration of CRISPR-Cas9 technology into various fields commands a rigorous examination of safety and ethical considerations. As this gene-editing tool is increasingly applied in research and therapy, stakeholders must remain vigilant regarding the potential implications and risks. Understanding both biosafety levels and ethical implications provides a framework for responsible research involving CRISPR-Cas9.
Biosafety Levels and Regulations
Biosafety is a critical aspect when working with genetically modified organisms. The Centers for Disease Control and Prevention (CDC) has outlined biosafety levels (BSL), which categorize laboratories based on the infectious agents they handle. For CRISPR research, adhering to these regulations is essential to protect personnel, the public, and the environment.
- BSL-1: This level involves microorganisms that do not cause disease in healthy humans and poses minimal risk. Basic laboratory practices are sufficient for handling.
- BSL-2: This level pertains to agents that pose moderate hazards. Specific precautions like limited access and decontamination procedures are necessary.
- BSL-3: Involves potentially lethal agents and requires controlled access. This involves more stringent safety measures, including specialized ventilation.
- BSL-4: This highest level is meant for pathogens that pose a severe risk. Laboratories operating at this level have extreme safety protocols, which may not be needed for most CRISPR applications but are crucial in certain contexts.
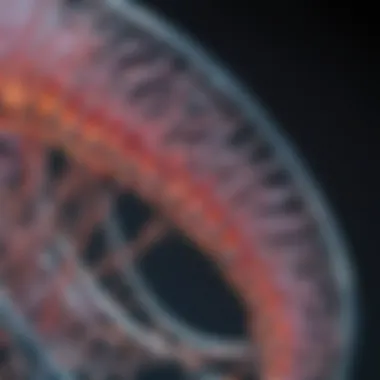
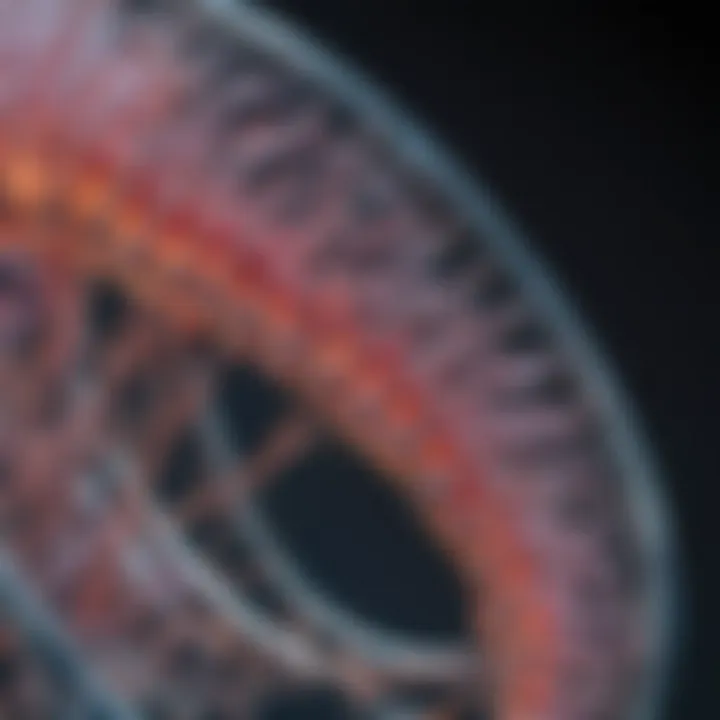
Researchers must also stay current on local regulations and institutional guidelines. Compliance with these biosafety levels ensures that experiments are conducted safely.
Ethical Implications of Gene Editing
The ethical implications surrounding CRISPR-Cas9 technology remain complex and controversial. The ability to edit genes presents opportunities along with moral dilemmas that must be navigated carefully. Some considerations include:
- Germline Editing: Modifying human embryos poses profound ethical questions. The alterations can be passed on to future generations, raising concerns about consent and unforeseen consequences.
- Equity in Access: As CRISPR technology evolves, ensuring equitable access is vital. This includes considering how socio-economic factors could influence who benefits from gene-editing advancements.
- Potential for Misuse: The dual-use nature of CRISPR poses risks of bioterrorism or misuse for non-therapeutic enhancements, prompting calls for strict regulations and oversight.
"The rapid advancement in gene editing technology like CRISPR demands an equally rapid development of ethical guidelines to keep pace with scientific innovation."
Troubleshooting Common Issues
In scientific research, the troubleshooting process is vital for success, especially when using complex technologies like CRISPR-Cas9. Understanding common issues that arise during gene editing can save time and resources. This section delves into key problems faced during CRISPR experiments and presents a structured approach to resolve them.
Identifying Common Pitfalls
Several frequent issues can hinder CRISPR experiments. Here are some notable ones:
- Inefficient Targeting: Low efficiency in gene editing is often linked to poorly designed guide RNAs (gRNAs) or suboptimal delivery methods.
- Off-Target Effects: Unintended edits may occur, leading to additional, undesired changes in the genome. This can complicate results and interpretation.
- Cell Toxicity: The presence of Cas9 nuclease can induce cellular stress. High concentrations or improper delivery can lead to the death of target cells.
- Incomplete Integration: Sometimes, the intended edits do not integrate properly into the genome, which can result in partial or absent modifications.
Identifying these pitfalls is essential for a smoother workflow. Researchers must remain vigilant during the design and execution of experiments.
Solutions to Enhance Protocol Success
To address the common issues discussed, researchers can adopt several strategies that enhance the success of CRISPR protocols:
- Optimize gRNA Design: Utilize tools like CRISPOR or Benchling to accurately design gRNAs. Choosing target sites with high specificity minimizes off-target effects.
- Use Controlled Delivery Methods: Techniques such as electroporation or the use of viral vectors should be optimized based on cell type. This can help improve transfection efficiency while reducing toxicity.
- Monitor Cell Viability: Perform assays to evaluate cell health post-transfection. This information can guide adjustments in the protocols.
- Validate Results Thoroughly: Implement robust validation methods such as sequencing and PCR. These approaches confirm that edits are made precisely and persistently.
- Adjust Cas9 Concentration: Careful titration of Cas9 can prevent cellular stress. Start with lower concentrations and gradually increase, monitoring for signs of toxicity.
"Understanding and addressing common issues can significantly improve experimental outcomes in CRISPR technology."
By following these recommendations, researchers can reduce the likelihood of encountering issues and enhance the overall effectiveness of their CRISPR-Cas9 protocols.
Future Directions in CRISPR Research
The future of CRISPR research appears promising and pivotal in shaping various scientific fields. As gene editing technology evolves, it opens avenues for both practical applications and ethical considerations. Researchers focus on enhancing existing methodologies and exploring new applications. Understanding these future directions can significantly impact the effectiveness and safety of gene editing.
Emerging Applications
New applications of CRISPR technology continue to arise, demonstrating its flexibility across different research domains. Key areas include:
- Agricultural Biotechnology: Modifying crops for disease resistance, pest tolerance, and improved nutritional content is a vital focus. CRISPR facilitates precise changes, unlike traditional methods, leading to faster advancements in food production.
- Medical Therapeutics: The use of CRISPR for curing genetic disorders is gaining momentum. Treatments for conditions such as cystic fibrosis and sickle cell disease show promise, pushing the boundaries of what is possible in personalized medicine.
- Gene Drives: This technology alters the inheritance patterns of genes in wild populations, potentially controlling invasive species or vector-borne diseases like malaria. While promising, this approach requires careful consideration of ecological impacts.
The emerging applications underline the necessity of rigorous research protocols to ensure safety and efficacy. Researchers must be vigilant to avoid unintended consequences in both environmental and health contexts.
Innovations in CRISPR Technology
Innovations are integral to the ongoing refinement of CRISPR technology. Some noteworthy advancements include:
- Base Editing: This method allows researchers to convert one DNA base pair into another without introducing double-strand breaks. This precision reduces the potential for off-target effects, making gene editing more efficient and safer.
- Prime Editing: Often referred to as a "search-and-replace" method for DNA, prime editing offers even higher accuracy than traditional techniques. It provides a tool for correcting genetic mutations, which can lead to breakthroughs in various genetic disorders.
- Delivery Mechanisms: Innovations in how CRISPR components are delivered into cells are crucial. Using nanoparticles or viral vectors can enhance targeting and reduce immune responses, improving the overall success rate of genome editing efforts.
These innovations aid in addressing some existing limitations within CRISPR methodologies, enhancing their effectiveness in both therapeutic and research settings. Continued investment in research and development is essential for realizing the full potential of these technologies.
Closure
The conclusion serves as a vital section within the context of CRISPR-Cas9 gene editing protocols. It encapsulates the main findings and presents the significant implications for researchers and practitioners in the field. The importance of having a structured conclusion cannot be understated. It allows for a synthesis of the key points discussed and highlights the ongoing relevance of CRISPR technology. This section helps to solidify the information presented earlier and implies the practical applications in various scientific contexts.
With the continuous evolution of gene editing techniques, it becomes crucial for researchers to stay updated and understand the implications of their work. By summarizing the essential components addressed throughout the article, the conclusion also serves as a thoughtful reminder of the complex landscape of CRISPR-Cas9 protocols. It would enhance a reader's understanding deepening their grasp of the technology's potential and fostering innovation.
Summary of Key Points
- CRISPR-Cas9 is a revolutionary gene-editing technology with a wide range of applications from basic research to clinical therapies.
- Understanding the protocol development process is essential to ensure effective implementation and achieve desired outcomes.
- Key components of CRISPR systems include guide RNA design, vector choice, and the methodology of transfection, all of which require careful consideration.
- Addressing safety and ethical concerns is crucial, given the power of gene editing technology.
- Ongoing troubleshooting techniques can significantly impact the success of CRISPR methods, guiding users to optimize their protocols.
Final Thoughts on CRISPR-Cas9 Protocols
As the field of gene editing expands, the CRISPR-Cas9 protocols remain central to understanding and utilizing its capabilities. Meticulous attention to detail in protocol design ensures accuracy and reliability in experiments. Researchers must embrace a mindset of continuous learning and adaptation. Emerging technologies such as base editing and prime editing signal a new era of precision in gene editing. This evolution implies that staying informed about advances in CRISPR technology will be fundamental.
In sum, the insights presented in this article not only position students and professionals for success but also encourage deeper engagement with CRISPR science. As knowledge of gene editing grows, so does the potential for innovative solutions in medicine, agriculture, and beyond.