Dispersive Infrared Spectrometry: Principles and Innovations
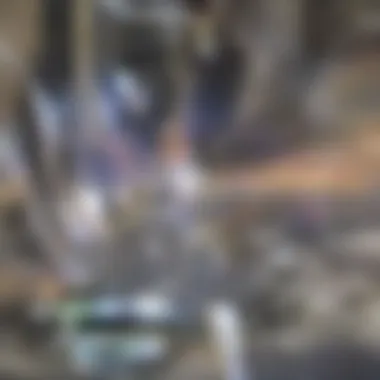
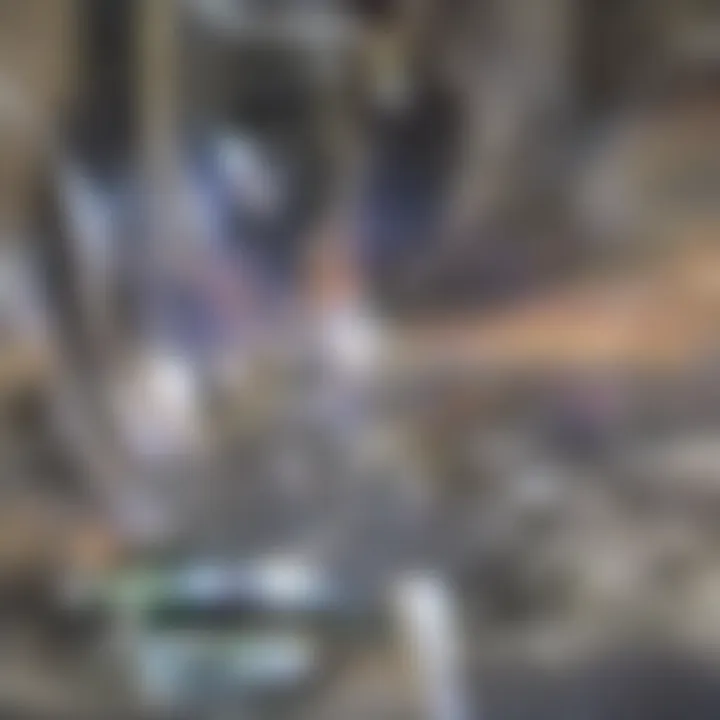
Intro
Dispersive infrared spectrometry holds a pivotal place in the realm of analytical techniques. It is essential for assessing the chemical composition of substances. This method relies on measuring the absorption of infrared radiation by a sample. In particular, dispersive IR spectrometers are designed to separate incoming infrared light into its component wavelengths. This capability is invaluable for both research and practical applications.
Throughout this article, we will explore the fundamental principles that underpin dispersive infrared spectrometry. Additionally, we will delve into its applications across various scientific fields. Technological advancements have propelled this method forward, making it more efficient and user-friendly.
The insights shared here will benefit students, researchers, educators, and professionals interested in this enriching analysis technique.
Research Overview
Summary of Key Findings
Dispersive infrared spectrometry is characterized by several key findings:
- Sensitivity: The technique is notably sensitive, allowing for the detection of trace amounts of substances.
- Versatility: It is applicable in different fields such as chemistry, environmental science, and materials research.
- Platform for Innovation: Recent advancements are focused on enhancing accuracy and functionality across disciplines.
Background and Context
The scientific community has long relied on infrared spectrometry to gather spectral data. Traditional dispersive infrared spectrometers utilize prisms or gratings to achieve spectral resolution. Consequently, they produce high-resolution spectra essential for qualitative and quantitative analysis.
The evolving demands of various research fields have intensified the focus on the innovations within this technology. For instance, advances in optical components as well as data processing techniques have significantly improved the capabilities of modern instruments.
"Dispersive infrared spectrometry provides a landmark in analytical chemistry, merging precision measurement with broad applicability."
In the next sections, we will discuss the operational principles of dispersive infrared spectrometers and evaluate their practical implications across scientific landscapes.
Prologue to Dispersive Infrared Spectrometry
Dispersive infrared spectrometry stands as a pivotal technique in the arsenal of analytical tools available to scientists and researchers. Its significance transcends mere data collection; it serves as a gateway to understanding molecular structures and interactions. This introductory section elucidates the relevance of dispersive infrared spectrometry, painting a picture of its importance in scientific exploration and industry application.
Definition and Overview
Dispersive infrared spectrometry is an analytical technique that measures the absorption of infrared light by a sample. During this process, infrared radiation interacts with the molecules in the material, resulting in energy transitions that can be detected and analyzed. The output of such measurements is typically represented in the form of spectra that detail how much light is absorbed at different wavelengths.
This technique primarily focuses on identifying and quantifying various compounds present in complex mixtures. The ability to resolve peaks in an infrared spectrum allows researchers to differentiate between similar compounds. As such, dispersive infrared spectrometry is particularly valuable in fields such as chemistry, environmental science, and material analysis.
Historical Context
The roots of dispersive infrared spectrometry trace back to the early 20th century. It was here that initial advancements in infrared physics initiated a transformation of spectroscopy. The development of instruments capable of isolating specific wavelengths through dispersion fundamentally altered how scientists engage with material analysis.
One noteworthy milestone was the introduction of the grating spectrometer in the 1920s. These advancements provided the backbone for many modern techniques employed in dispersive infrared spectrometry today. Furthermore, the emergence of Fourier-transform infrared spectrometry in the latter half of the century sparked a new era in spectral analysis, offering enhanced sensitivity and speed. Dispersive techniques remain relevant due to their simplicity and effectiveness in a variety of applications.
As we proceed through the chapters of this article, we will delve deeper into the fundamental principles, components, advantages, limitations, and the evolving landscape of dispersive infrared spectrometry.
Fundamental Principles of IR Spectrometry
Understanding the fundamental principles of infrared spectrometry is essential for appreciating how dispersive infrared spectrometers function. This section highlights the intricacies of infrared radiation and its interaction with matter, as well as how these processes result in distinct transmission and absorption spectra. By grasping these principles, readers can better understand the various applications and technological innovations discussed later in the article.
The Nature of Infrared Radiation
Infrared radiation is part of the electromagnetic spectrum, lying between visible light and microwave radiation. This section of the spectrum is characterized by longer wavelengths and lower frequencies than visible light. Typically, infrared radiation ranges from about 0.75 to 1000 micrometers in wavelength. It is crucial to recognize that different materials absorb infrared radiation at specific wavelengths, leading to characteristic absorption bands.
The energy of infrared radiation correlates to its wavelength. Materials can absorb energy from infrared radiation, which induces molecular vibrations. These vibrations can be stretching or bending of chemical bonds, which results in a unique spectrum for different compounds.
Interaction of Infrared Light with Matter

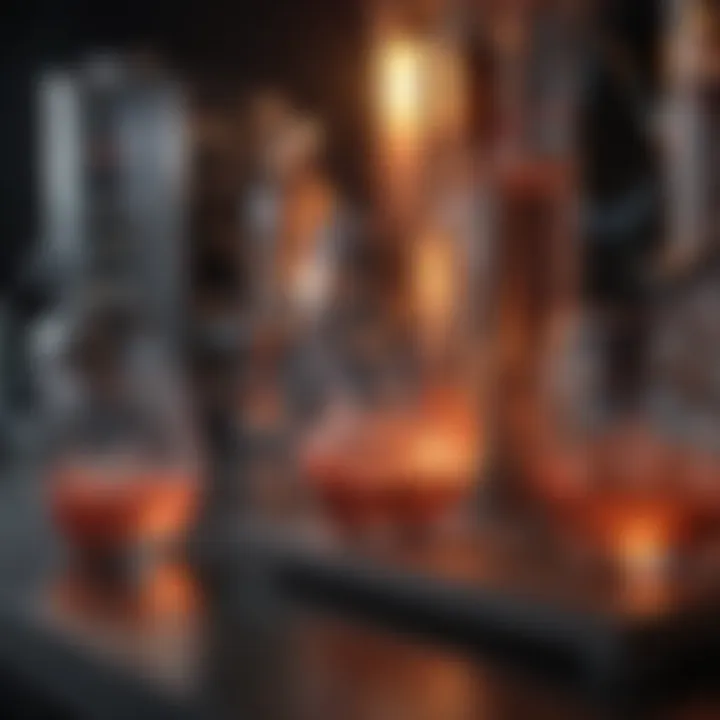
When infrared light interacts with matter, several phenomena can occur. Primarily, absorption and transmission define how molecules respond to IR radiation. Each material's molecular structure determines its interaction with infrared light, leading to two primary concepts:
- Absorption: When infrared light is absorbed by a molecule, it elevates the vibrational energy states of the molecule, making it critical for identifying chemical bonds within a substance.
- Transmission: Certain wavelengths pass through materials without being absorbed. This transmission of light is used to ascertain the properties of the material being analyzed.
Understanding this interaction is essential for analyzing complex mixtures and recognizing distinct compounds within samples. Compounds with different functional groups exhibit a variety of absorption patterns, providing a fingerprint for identification.
Transmission and Absorption Spectra
The transmission and absorption spectra serve as a visual representation of how materials interact with infrared radiation. The transmission spectrum shows how much light passes through a sample, while the absorption spectrum indicates the specific wavelengths absorbed by the material.
Key aspects of these spectra include:
- Wavelength Ranges: Different regions of the infrared spectrum correspond to different molecular vibrations. The mid-infrared range (around 4000-400 cm^-1) is particularly informative for most organic compounds because it correlates to fundamental vibrations of chemical bonds.
- Peak Identification: The presence of distinct peaks in absorption spectra signifies the presence of specific functional groups. For example, a sharp peak near 1700 cm^-1 usually indicates a carbonyl (C=O) group.
By analyzing these spectra, researchers can deduce chemical composition, purity, and structural characteristics of various substances.
"Infrared spectroscopy is an essential tool in analyzing the molecular structure and interactions within complex mixtures."
Components of Dispersive IR Spectrometers
Dispersive infrared spectrometers rely on several vital components to enable the precise analysis of materials through their infrared absorption characteristics. Understanding these components clarifies their operational roles and advantages. Each part, from the source of infrared radiation to the detectors, contributes to the overall functioning and efficacy of the instrument. Analyzing these elements provides insights into how they work together to achieve reliable measurements in various applications.
Source of Infrared Radiation
The source of infrared radiation is crucial for the spectrometer's operation. Typically, this source is either a thermal emitter or a non-thermal light source. Thermal sources, such as globar or Nernst glowers, provide a broad spectrum of infrared light. This wide emission range enables detection of a variety of sample types across multiple applications.
Non-thermal sources, like laser diodes, offer more specific wavelengths and allow for pinpointed analysis of certain chemical species. A quality source is necessary for adequate signal intensity and wavelength range, consequently affecting the overall resolution of the spectrometer.
Optical Elements
Optical elements in dispersive IR spectrometers determine how the infrared radiation is manipulated and directed toward the sample and the detector. These elements include prisms, dichroic mirrors, and lenses. Each plays a distinct role in the spectrometric process.
Prisms
Prisms are a fundamental optical element that disperses infrared light based on wavelength. A typical prism’s key characteristic is its capacity to separate light into its constituent wavelengths effectively. This capability makes prisms especially beneficial for obtaining high-resolution spectra. The unique feature of prisms is their material composition, often made from mid-infrared transparent substances like potassium bromide or germanium, which enhances their utility in the infrared range. However, the main disadvantage might be in the form factor and potential complexity in alignment during instrumentation setup.
Dichroic Mirrors
Dichroic mirrors contribute significantly by reflecting certain wavelengths while allowing others to pass through. This key characteristic makes them essential for filtering unwanted wavelengths from the signal path. Their unique feature is that they can be specifically designed to target and enhance certain infrared spectra; this is crucial in applications requiring precise wavelength management. Despite their benefits, dichroic mirrors might add complexity and require careful calibration within the spectrometer's optical path.
Lenses
Lenses in dispersive IR spectrometers are instrumental for focusing and directing infrared light toward the detector or sample. They can shape the beam and improve the efficiency of light transmission. The key characteristic of lenses is that they provide versatile options for focusing light, enhancing the spectrometer’s capabilities. Their unique features, such as being made from materials like germanium or chalcogenide glasses suitable for IR wavelengths, add to the advantages they offer. However, improper lens alignment or selection can lead to aberrations, impacting data quality.
Detectors
Detectors play a pivotal role in converting the incoming infrared radiation into measurable signals. Different types of detectors, such as thermocouples, pyroelectric detectors, and photoconductive detectors, have unique properties that suit them for various applications. Each detector type impacts the instrument's sensitivity, response time, and overall analytical capabilities.
Understanding how these components work together is essential for leveraging the full potential of dispersive IR spectrometry in scientific analysis.
Advantages of Dispersive IR Spectrometry
Dispersive infrared spectrometry has several key advantages, which greatly enhance its utility across various scientific fields. Understanding these benefits is essential for comprehending the relevance of this technology.
Resolution and Sensitivity
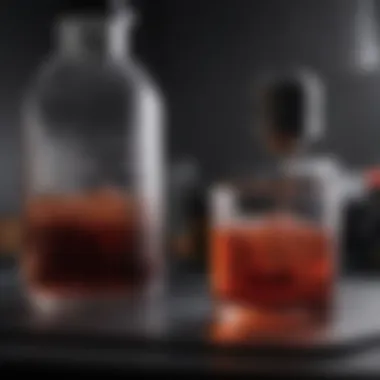
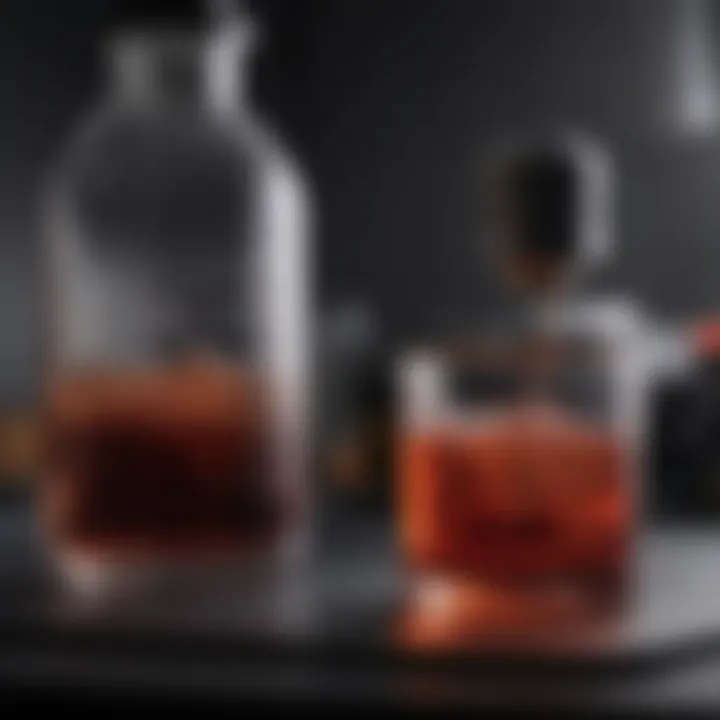
One of the primary advantages of dispersive infrared spectrometry lies in its high resolution and sensitivity. The design of these spectrometers allows for better separation of spectral lines, leading to clearer and more informative spectra. Higher resolution enables the detection of subtle differences in chemical composition. This precision is crucial in research settings where distinguishing between compounds is necessary, especially in complex samples.
Moreover, the sensitivity of dispersive IR spectrometers is often superior to other infrared methods. They are capable of detecting low concentrations of analytes, making them effective for trace analysis. This characteristic is particularly advantageous in environmental monitoring and quality control in food industries.
Range of Applications
The versatility of dispersive infrared spectrometry extends to a wide range of applications. In chemical analysis, it plays an important role in identifying functional groups and determining molecular structure. The ability to analyze various samples adds significant value, as practitioners can utilize this technique in diverse fields such as:
- Material Science: Identifying material compositions and studying phase transitions.
- Environmental Monitoring: Assessing air quality and analyzing pollutants.
- Food Quality Control: Ensuring safety and quality by detecting contaminants and adulterants.
Furthermore, its integration with other analytical methods enhances its applications. Combining dispersive infrared spectrometry with liquid chromatography or mass spectrometry expands its capabilities in complex analyses, providing detailed insights into sample matrices.
The adaptability of dispersive infrared spectrometry ensures its relevance in contemporary research and industrial applications, making it an indispensable tool in many laboratories.
In summary, the advantages of dispersive infrared spectrometry, primarily its high resolution and sensitivity and its broad range of applications, demonstrate its importance. These aspects contribute to its standing as a preferred technique within various scientific disciplines.
Limitations of Dispersive IR Spectrometry
Understanding the limitations of dispersive infrared spectrometry is essential for a well-rounded view of its role in scientific inquiry. While this technique offers numerous advantages, it is not without its challenges. The limitations can affect precision, efficiency, and applicability in certain scenarios. In this section, we will explore these limitations, focusing on key aspects that researchers and practitioners must consider.
Sample Preparation Challenges
Sample preparation is often the most crucial step in obtaining reliable results from dispersive infrared spectrometry. Many substances require specific forms or conditions for analysis. Solid samples, for example, may need to be ground into fine powders or mixed with a suitable matrix, which can introduce variability. Furthermore, not all materials are amenable to IR analysis; some may produce weak signals that are difficult to interpret.
Additionally, the physical and chemical properties of the sample must be considered.
- High moisture content can affect the spectral output.
- Contaminants may interfere with measurements, leading to erroneous conclusions.
It is imperative for users of dispersive IR spectrometry to develop robust sample preparation protocols that minimize these issues. This involves thorough understanding of the sample’s characteristics and the formulation of consistent procedures.
Time Consumption in Measurement
The time required for measurements using dispersive infrared spectrometry can hinder its effectiveness in high-throughput settings. Each sample must be carefully aligned and analyzed, a task which can be labor-intensive. Additionally, acquiring and processing spectra can take significant time unless automated systems are employed. This becomes particularly evident when dealing with multiple samples or when rapid results are needed.
While advancements have improved the speed and efficiency of dispersive IR spectrometry, it still cannot match the rapid analysis capabilities offered by some alternative techniques, such as near-infrared spectroscopy. Researchers must weigh the benefits of dispersive IR spectrometry against the time constraints of their specific applications and might need to allocate sufficient resources for sample processing and analysis.
"Understanding limitations is key to leveraging the strengths of dispersive infrared spectrometry effectively."
Overall, while dispersive infrared spectrometry remains a powerful analytical tool, it is essential to acknowledge and address its limitations. A careful approach to sample preparation and an awareness of the time investment required can lead to more accurate results and effective use of this method in various scientific domains.
Applications of Dispersive IR Spectrometers
Dispersive infrared (IR) spectrometry plays an important role in multiple scientific fields. Its applications range from chemical analysis to food quality control, each demonstrating distinct benefits and capabilities. Understanding these applications is essential for researchers and practitioners who utilize this technology. This section will explore the various uses of dispersive IR spectrometers, emphasizing their significance and impact in several domains.
Chemical Analysis
One of the primary uses of dispersive IR spectrometers is in the realm of chemical analysis. This technique enables the identification and quantification of chemical compounds present in a sample. When infrared light interacts with a substance, specific wavelengths are absorbed, which corresponds to the distinctive vibrational modes of the molecules within that substance. This results in a spectrum unique to each compound, thus facilitating identification.
- Compound Identification: By analyzing the absorption peaks in the spectrum, chemists can determine the functional groups present in an organic compound.
- Quantitative Analysis: The intensity of the absorption bands can provide information about the concentration of specific compounds within a sample.
- Monitoring Reactions: Dispersive IR can track changes in chemical composition during reactions, offering insights into chemical kinetics and mechanisms.
Material Science
In the field of material science, dispersive IR spectrometry serves as a powerful tool for studying the properties of materials. The technique can assess the composition and molecular structure of polymers, ceramics, and composite materials. This information is critical for developing and optimizing new materials.
- Characterization of Materials: By obtaining the IR spectra of materials, scientists can identify functional groups and assess material purity.
- Thermal Analysis: Dispersive IR can evaluate thermal stability through thermogravimetric analysis combined with IR measurements.
- Material Development: Researchers can utilize IR spectrometry to experiment with new materials, enabling the design of materials with customized properties tailored for specific applications.
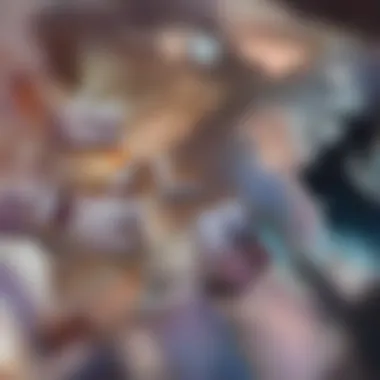
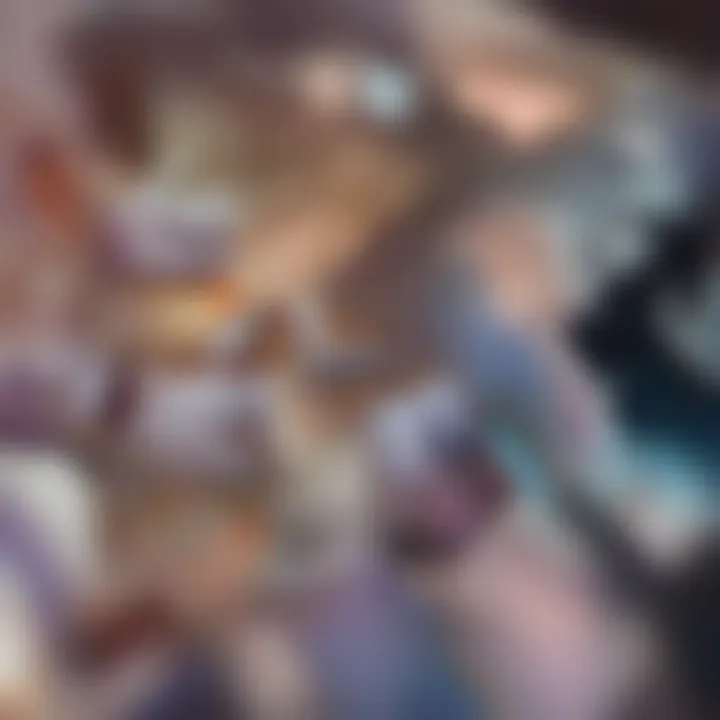
Environmental Monitoring
Dispersive IR spectrometry is instrumental in environmental monitoring. It provides valuable data concerning air quality, soil composition, and water pollutants. The ability to identify and quantify hazardous substances is critical for environmental protection and regulatory compliance.
- Air Quality Analysis: The technique can detect and measure concentrations of gases such as carbon dioxide, methane, and volatile organic compounds (VOCs).
- Water Quality Assessment: By analyzing water samples, researchers can identify contaminants, helping organizations to develop strategies for pollution control.
- Soil Analysis: Dispersive IR aids in evaluating soil samples, revealing information about organic matter content and pollutants.
Food Quality Control
Food safety and quality assurance have become increasingly important in the food industry. Dispersive IR spectrometry plays a crucial role in ensuring compliance with safety standards and meeting consumer expectations. This method allows for quick and accurate assessments of food products.
- Ingredient Verification: The technology can verify the composition of food ingredients, ensuring that products meet labeling claims.
- Contaminant Detection: Dispersive IR is effective in identifying harmful substances, including pesticides and additives.
- Quality Assessment: Through spectral analysis, food scientists can evaluate the freshness and quality of products, helping to reduce waste and ensure consumer safety.
The applications of dispersive IR spectrometry are vast, providing essential insights across various fields, from ensuring food safety to monitoring environmental health.
Current Trends in Dispersive IR Spectrometry
Understanding current trends in dispersive infrared spectrometry is vital as it highlights how the field continues to evolve. These trends reflect ongoing advancements in technology, methods, and applications that enhance the effectiveness of IR spectrometers. Awareness of these trends allows researchers and professionals to stay informed about the capabilities of modern spectrometry and adapt accordingly.
Miniaturization and Portability
The trend toward miniaturization in dispersive IR spectrometry is influential. As researchers seek greater ease of use and flexibility, portable spectrometers have become necessary. Smaller devices maintain essential features of larger models while being easily transportable. This portability opens avenues for data collection in remote locations or on-site environments, which is beneficial for field studies and real-time monitoring.
The miniaturization also leads to cost-effective solutions. Development of compact components allows companies to design more affordable instruments without compromising performance. These advancements cater to increasing demand in both academic and industrial settings, where quick analysis is crucial.
Integration with Other Techniques
Another significant trend is the integration of dispersive IR spectrometry with complementary methods. Combining techniques like mass spectrometry or gas chromatography enhances analytical capabilities. This synergy results in a more profound understanding of chemical compositions and structures.
For instance, coupling IR spectroscopy with chromatography helps in identifying various compounds in complex mixtures. This is particularly useful in fields such as pharmaceuticals and material science. Various combinations can optimize performance, leading to more precise measurements and faster analysis.
"Integrating dispersive IR spectrometry with other analytical techniques can enhance the depth of data analysis, revealing insights that may go unnoticed when using isolated methods."
Software and Data Analysis Advances
In the era of big data, software solutions and data analysis methods are crucial. Developments in machine learning and artificial intelligence have transformed how spectroscopic data is interpreted. These sophisticated algorithms can quickly analyze large datasets, identifying patterns and anomalies that human analysts might overlook.
Moreover, improved software interfaces streamline user operations, allowing researchers to focus on data interpretation rather than technical difficulties. Enhanced visualization tools aid in comprehending complex data sets more intuitively. Consequently, the advancements in software mark a significant step forward in optimizing the utility of dispersive IR spectrometers.
Future Prospects of Dispersive IR Spectrometry
The future of dispersive infrared spectrometry holds significant promise as advancements in technology pave the way for enhanced capabilities and wider applications. This section will explore key developments expected to shape the field, focusing on innovations in detector technology and the opportunities for expanding applications. These aspects are critical in determining how dispersive IR spectrometry will remain relevant in various scientific disciplines.
Innovations in Detector Technology
One of the most notable areas of advancement is in detector technology. Traditional detectors like thermocouples and pyroelectric devices have served their purpose, yet newer detectors like quantum cascade lasers and photodiode arrays are gaining traction.
These innovative detectors provide several benefits:
- Increased Sensitivity: Enhanced sensitivity allows for the detection of lower quantities of samples, which is crucial in fields like environmental monitoring.
- Faster Response Times: Modern detectors can capture data more rapidly, improving the overall efficiency of the analysis process.
- Wider Wavelength Range: Innovative detectors are capable of detecting a broader spectrum of infrared wavelengths. This expands the potential for analyzing a diverse range of substances.
Such advancements ensure that dispersive IR spectrometry is not only precise but also adaptable to changing research needs. With these innovations, researchers can expect to achieve results that were previously unfeasible, thereby furthering the applications of this method.
Expanding the Range of Applications
As the capabilities of dispersive infrared spectrometry grow, so too does the scope of its applications. Various sectors can benefit from the enhanced technology these advancements provide. Several areas where dispersive IR spectrometry is poised to expand include:
- Biomedical Research: The ability to analyze biological samples with precision could lead to breakthroughs in disease diagnostics and treatment assessment.
- Pharmaceutical Development: Enhanced capabilities can streamline drug formulation processes by allowing faster and more accurate chemical analysis.
- Nanotechnology: As materials at the nanoscale require specific analysis techniques, dispersive IR spectrometry could be adapted to study these novel materials effectively.
"Expanding applications profoundly influence how we approach complex problems in science and technology."
The potential for growth in these application areas is vast, and with continued investment in research and development, dispersive infrared spectrometry is set to meet the challenges of emerging fields. As methodologies evolve, each advancement opens new avenues for innovation, ultimately contributing to a deeper understanding of materials in various contexts.
In summary, the future of dispersive IR spectrometry is bright, driven by innovations in detector technology and the expanding range of applications. As scientists explore the limits of this technology, it is likely to play a central role in various significant advancements in analytical chemistry and related fields.