In-Depth Exploration of Hydrodynamics: Principles and Applications
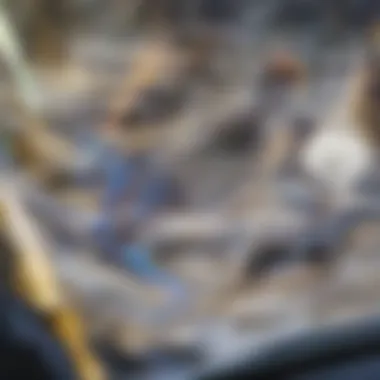
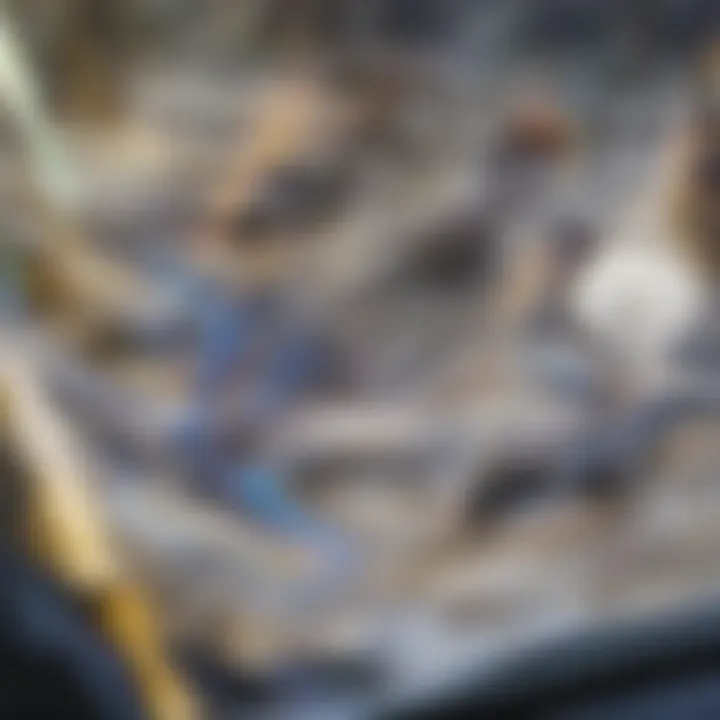
Intro
Hydrodynamics is a branch of fluid mechanics that focuses on the behavior of fluids in motion, a critical area of study that intertwines various scientific and engineering disciplines. Understanding hydrodynamic principles is essential for applications ranging from civil engineering to meteorology and even biophysics. The core concept revolves around how fluids interact with their surroundings, influenced by factors such as pressure, velocity, and viscosity.
In this article, we will explore both the foundational theories and the more advanced principles of hydrodynamics. We will delve into how these principles are applied in real-world situations, making connections between theoretical models and practical outcomes. Thus, this exploration aims not only to inform but also to enhance the reader’s appreciation for the complexities of fluid motion.
Research Overview
Summary of Key Findings
Recent studies in hydrodynamics have highlighted several critical insights:
- The importance of Reynolds number in characterizing flow regimes
- Development of computational fluid dynamics (CFD) tools for simulation
- Influence of turbulence on drag and lift in various engineering applications
Hydrodynamics research has advanced significantly, revealing complexities in turbulent flow and improving accuracy in predictive modeling. These findings are invaluable for industries that rely on fluid behaviors, such as aerospace and marine engineering.
Background and Context
The origins of hydrodynamics can be traced back to ancient civilizations, where scholars began to study water motion's practical implications. Still, it was not until the 18th century that significant theoretical frameworks emerged, particularly from notable figures like Daniel Bernoulli and Leonhard Euler. Their work laid the groundwork for understanding fluid behavior under various conditions.
In the modern context, hydrodynamics has further evolved with the aid of advanced technologies. Researchers leverage high-performance computing to solve complex equations governing fluid behavior, leading to practical applications in aircraft design and hydraulic systems. The continuous rise in computational capabilities signifies an era of great potential for further advancements in this discipline.
Methodology
Experimental Design
The research methodologies employed in hydrodynamics often include both theoretical and experimental approaches. For theoretical analysis, fluid dynamics equations such as the Navier-Stokes equations are central to predicting fluid flow behavior.
Experimental designs typically involve wind tunnel tests or water channel studies, providing tangible insights into fluid dynamics under controlled conditions.
Data Collection Techniques
Data collection in hydrodynamic research relies on various techniques:
- Pressure measurement using manometers or pressure sensors
- Velocity profiling through laser Doppler anemometry
- Visualization techniques such as particle image velocimetry (PIV)
These methods allow for precise monitoring of fluid movement, aiding in the understanding of complex dynamics at play in different scenarios.
Understanding Hydrodynamics
Hydrodynamics serves as a pivotal aspect of fluid mechanics, focusing specifically on fluids in motion. Understanding hydrodynamics is essential because it provides insights into how forces interact with fluids, which is fundamental in many scientific and engineering contexts. The applications reach from designing efficient watercraft to analyzing natural river systems, influencing both technology and environmental science.
It is vital to grasp the foundational principles of hydrodynamics to understand complex phenomena like turbulence, wave behavior, and fluid interaction with solid surfaces.
Definition and Scope
Hydrodynamics, in its simplest form, pertains to the study of fluids in motion. This includes liquids, gases, and plasmas. The field encompasses various sub-disciplines, such as aerodynamics, which focuses on gas behavior, and hydrology, which studies water movement.
The scope of hydrodynamics stretches across multiple domains, including engineering, physics, and environmental studies. By employing mathematical models and experimental setups, engineers and scientists analyze and predict fluid behavior under diverse conditions. This analysis is crucial for ensuring the reliability and efficiency of systems involving fluid flow, such as pipelines, pumps, and wings of aircraft.
Historical Background
The roots of hydrodynamics date back to the ancient civilizations that first studied the movement of water. Figures like Archimedes made significant contributions, particularly with his principle concerning buoyancy. Fast forward to the 18th century, where Daniel Bernoulli's work laid the groundwork for understanding energy conservation in fluid motion. His equation, known as Bernoulli's Equation, is a cornerstone in the study of hydrodynamics.
The development continued with the Navier-Stokes equations in the 19th century, providing a comprehensive mathematical framework for the analysis of steady and unsteady flows. These equations describe the motion of fluid substances and have become vital for advanced studies in colleges worldwide.
Importance in Various Disciplines
Hydrodynamics plays a critical role in a variety of fields:
- Engineering: In aerospace engineering, understanding hydrodynamics is key for optimizing aircraft and spacecraft designs. Efficiency is paramount, and too much drag can compromise performance.
- Environmental Science: Rivers and lakes are influenced by hydrodynamic principles. Knowledge of fluid motions assists in managing reservoirs and predicting pollution dispersal.
- Biology: Fluid dynamics has implications in understanding blood flow in animals, aiding medical advancements and surgical techniques.
- Renewable Energy: The design of turbines and other energy generation systems relies on understanding water and wind flow dynamics to maximize efficiency.

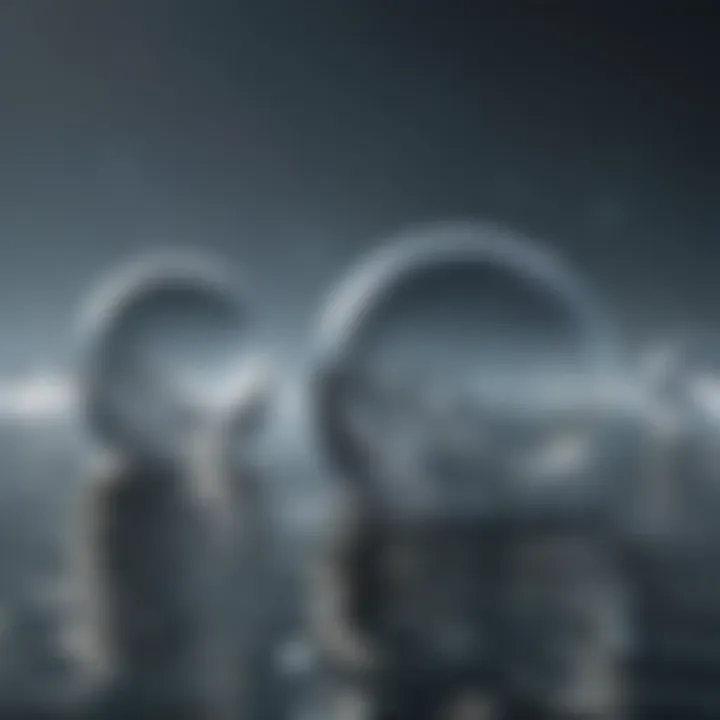
The interconnectedness of these disciplines showcases how hydrodynamics extends beyond traditional boundaries, influencing innovations and research across a wide array of fields.
Theoretical Foundations
Understanding the theoretical foundations of hydrodynamics is essential for grasping its principles and applications. These foundations offer a framework that connects the physical and mathematical aspects of fluids in motion. They dictate how we analyze fluid behavior and predict how various systems will perform under different conditions. This section covers critical components, including fluid properties, fundamental equations, and dimensional analysis, laying the groundwork for applying hydrodynamic principles effectively in diverse contexts.
Fluid Properties
Density
Density plays a vital role in hydrodynamics. It refers to the mass per unit volume of a fluid. In the context of hydrodynamics, density affects buoyancy, pressure, and flow characteristics of fluids. A key characteristic of density is that it dictates how fluids interact with solid bodies. This makes density a fundamental property that is crucial for designing systems related to fluid transport, storage, and mixing. Moreover, varying density can indicate different substances in a mixture, allowing for density-based separation techniques.
A unique feature of density is its variation with temperature and pressure. As temperature increases, most fluids experience a decrease in density, which can affect flow regimes in natural and engineered systems. This property can be both an advantage and a disadvantage; while it allows for efficient thermal regulation in certain processes, it can lead to complications in predicting flow behavior across temperature gradients.
Viscosity
Viscosity measures a fluid's resistance to deformation and flow. In hydrodynamics, it significantly influences how fluids move, especially in laminar flow conditions. This property is essential for applications such as lubrication and fluid transport. The key characteristic of viscosity is its ability to govern the rate at which a fluid flows under an applied force. This makes viscosity a pivotal aspect for simulations in computational fluid dynamics.
Viscosity presents unique challenges in hydrodynamic analysis. It can increase with temperature, leading to non-Newtonian behavior in some cases, where the flow characteristics of a fluid change with the rate of shear stress. Understanding viscosity becomes critical in applications such as designing hydraulic systems, where maintaining optimal flow rates is crucial. Its complex behavior can complicate mathematical modeling, but this complexity also adds depth to our understanding of fluid dynamics.
Surface Tension
Surface tension is the elastic-like force existing at the surface of a fluid. This property results from cohesive forces between fluid molecules, allowing them to resist external forces. In hydrodynamics, surface tension influences phenomena like droplet formation and wave behavior. The key characteristic of surface tension is its ability to create interfaces between different fluids and between fluids and gases. This is particularly relevant in applications such as inkjet printing and spray technology.
A unique feature of surface tension is its role in stabilizing bubbles and droplets in fluids. This can be an advantage in processes where control over particle size is critical, like emulsions in food products. However, the same property can lead to difficulties in mixing and dispersion, revealing a potential disadvantage in other contexts. Understanding surface tension thus enhances our ability to manipulate fluid behavior in various applications.
Fundamental Equations
Bernoulli’s Equation
Bernoulli’s Equation relates the pressure, velocity, and elevation of a fluid in steady flow. Its fundamental aspect is the conservation of energy principle applied to fluid motion. This equation is a powerful tool in hydrodynamics, aiding in the analysis of fluid behavior in various contexts, such as pipe flows and aerodynamics. The key feature of Bernoulli’s Equation is that it underscores the interaction of pressure and velocity, demonstrating that an increase in the velocity of a fluid correlates with a decrease in pressure.
Adequately applying Bernoulli’s Equation provides insights into flow characteristics without requiring complex calculations. However, it has limitations. It assumes incompressible, non-viscous fluids in steady flow, which may not hold true in all scenarios. Therefore, while it is an excellent starting point, Bernoulli's principles should be applied with caution in complex fluid dynamics situations.
Navier-Stokes Equations
The Navier-Stokes equations extend the study of fluid dynamics beyond the limitations of Bernoulli’s equation. They describe the motion of viscous fluid substances and are fundamental to understanding complex fluid flow conditions. The key aspect of the Navier-Stokes equations is that they incorporate viscosity and can model a wide range of flow behaviors, from laminar to turbulent flows. These equations are essential for contemporary computational fluid dynamics simulations.
One unique feature of the Navier-Stokes equations is their ability to predict turbulence, a significant challenge in fluid dynamics. However, solving these equations analytically is often complex and sometimes impossible for certain flow conditions. This presents both a challenge and an opportunity for research in hydrodynamics, as improvements in computational methods continue to evolve.
Dimensional Analysis
Reynolds Number
The Reynolds number is a dimensionless quantity used to predict flow patterns in different fluid flow situations. It is calculated by the ratio of inertial forces to viscous forces and is a crucial element of dimensional analysis in fluid dynamics. The key characteristic of the Reynolds number is its ability to categorize flows as either laminar or turbulent, providing insights into how fluid systems behave.
Utilizing the Reynolds number helps in scaling experiments and predicting the behavior of fluid systems in engineering applications. A high Reynolds number indicates turbulent flow, while a low number signifies laminar flow. Understanding this property allows engineers and scientists to anticipate and control flow conditions effectively.
However, the Reynolds number also has its limitations. It does not capture all fluid characteristics and behaviors, especially in complex flows involving multiple phases. Therefore, while it serves as an effective tool for initial assessments, practitioners should consider additional factors in designing fluid systems.
Froude Number
The Froude number is another important dimensionless quantity. It compares inertial forces to gravitational forces, especially in open channel flows. Its key characteristic is that it serves as a useful parameter in determining wave propagation and flow regime transitions.
Utilizing the Froude number allows engineers to assess the potential for wave action and flow patterns in various applications, particularly in hydraulic engineering. Its significance lies in its applicability to problems involving gravity-driven flows, such as rivers and spillways.
A unique aspect of the Froude number is that it helps in analyzing flow stability and behavior under different loading conditions. However, like the Reynolds number, it may oversimplify fluid behavior and must be used judiciously when addressing complex fluid dynamics scenarios.
The understanding of these theoretical foundations is critical for advancing both academic research and practical applications in hydrodynamics. They provide the tools necessary for scientists and engineers to design efficient systems and predict fluid behavior under different conditions.
Experimental Techniques
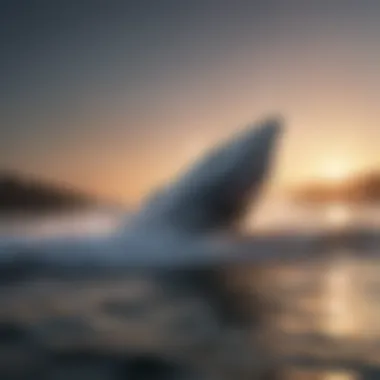
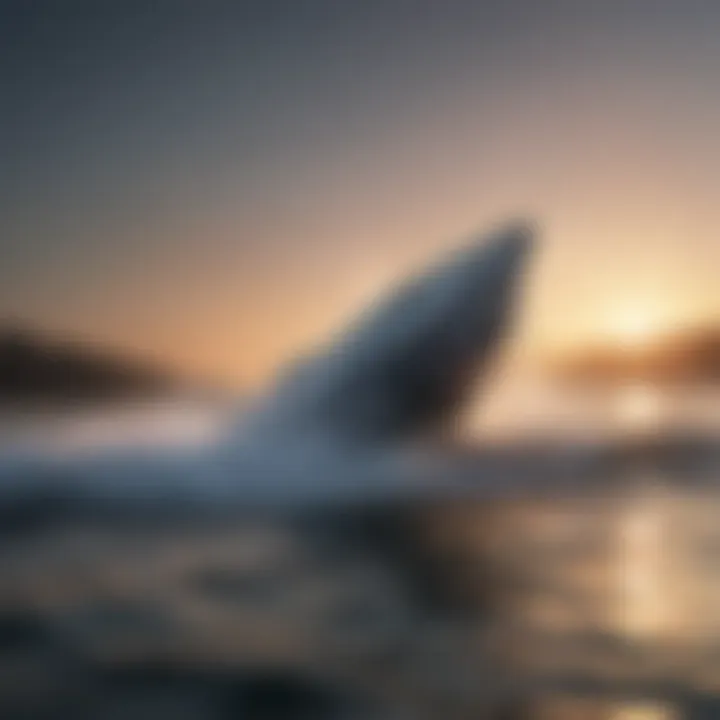
Experimental techniques are crucial when it comes to hydrodynamics. They provide the means to visualize fluid flow, measure fluid properties, and validate theoretical models. These techniques help researchers gather data that reflects how fluids behave under real-world conditions. Understanding fluid dynamics requires both theoretical and empirical approaches, and these techniques play an integral role in bridging that gap.
Flow Visualization
Flow visualization allows scientists to observe the motion of fluids. It makes complex flow patterns accessible for analysis and understanding. This technique is essential for developing models of fluid motion, which can inform various applications.
Particle Image Velocimetry
Particle Image Velocimetry (PIV) is a sophisticated technique widely used in hydrodynamics studies. This method employs laser light and digital cameras to capture images of seeded particles suspended in the fluid. The notable aspect of PIV is its ability to provide detailed velocity measurements of fluid flow at multiple points in a given area simultaneously. This unique feature makes it a powerful tool for analyzing intricate flow patterns in both laboratory and field conditions. The benefit of PIV lies in its accuracy and high spatial resolution. However, it does have limitations, such as the necessity for seed particles to be present in the fluid, potentially influencing the flow itself.
Laser Doppler Velocimetry
Laser Doppler Velocimetry (LDV) is another cutting-edge technique used for measuring fluid velocity. This method relies on the Doppler effect, which occurs when a laser beam is scattered by particles in the fluid. A significant characteristic of LDV is its non-intrusive nature. By allowing measurements without physical contact, it minimizes disturbance to the flow being studied. This approach is beneficial in various applications, including both minute and large-scale fluid dynamics. The key advantage of LDV is its ability to provide accurate measurements in turbulent flows. Nevertheless, it can be expensive and complex to set up, which might limit its usage in some circumstances.
Measurement Instruments
Measurement instruments are vital tools in hydrodynamics. They help quantify various parameters like flow rate, velocity, and pressure, which are critical for understanding fluid behaviors. The accuracy and reliability of these instruments significantly influence the quality of the data collected during experiments.
Pitot Tubes
Pitot tubes are instruments used to measure fluid flow velocity. They function based on the difference between dynamic and static pressure. A key characteristic of Pitot tubes is their simplicity. They are inexpensive and easy to use, making them a popular choice in both educational and professional settings. Their unique feature is their ability to provide measurements in a broad range of conditions, including high-speed flows. However, they have limitations when used in flows with high turbulence because they can yield inaccurate readings.
Flow Meters
Flow meters are devices designed to measure the volume or mass of fluid moving through a system. They come in various types, including mechanical, electromagnetic, and ultrasonic flow meters. A significant aspect is their versatility in application; flow meters can be used in various contexts, from industrial systems to environmental monitoring. Their unique feature is the ability to provide real-time measurements, which is critical for monitoring fluid dynamics effectively. The advantages include ease of use and the ability to handle different fluid types. However, they can be susceptible to calibration issues and may require regular maintenance to ensure accurate readings.
Common Applications
Hydrodynamics plays a significant role in various practical fields. Understanding the ways in which fluid dynamics apply can enhance designs, improve efficiency, and solve real-world problems. In engineering, environmental sciences, and even aerodynamics, the principles of hydrodynamics offer essential insights that promote innovation and resilience.
Engineering Applications
Hydraulic Systems
Hydraulic systems are a crucial application of hydrodynamics. They use pressurized fluids to transmit energy. This allows for powerful machines with comparatively low energy input. The key characteristic of hydraulic systems is their ability to multiply force, making them popular in construction and automotive applications. For instance, hydraulic lifts and braking systems leverage this principle.
One unique feature of hydraulic systems is the incompressibility of fluids, which leads to predictable and reliable behavior. However, one must also consider the downsides, such as potential fluid leaks and the need for regular maintenance. This interplay of advantages and disadvantages underscores the importance of well-designed hydraulic systems in engineering.
Ship Design
Ship design is another prominent aspect within engineering applications. It involves creating vessels that navigate fluid environments effectively. The key characteristic here is the balance between buoyancy and drag. Well-designed ships enhance energy efficiency and performance while reducing environmental impact.
A critical feature of ship design is utilizing hydrodynamic shapes. These shapes minimize drag and resistance in water, improving fuel efficiency. However, achieving the optimal design can be challenging and may require expensive materials or extensive testing. Understanding these factors helps highlight the importance of hydrodynamics in ship design, contributing to safer and more efficient vessels.
Environmental Sciences
River Hydraulics
River hydraulics represents an essential area of study in environmental sciences. It focuses on the behavior of water flow in river systems and how this flow impacts ecosystems. A key aspect of river hydraulics is the assessment of flow regimes, which affects sediment transport and habitat availability.
A notable benefit of studying river hydraulics is its application in flood management. By understanding how rivers behave during high flow, engineers can develop strategies to mitigate flooding risks. However, modeling river hydraulics can be complex due to varying conditions such as obstructions and turbulence. That's why having accurate models is crucial for predicting river behavior in different scenarios.
Ocean Circulation
Ocean circulation is another vital part of environmental sciences. It examines how water moves across the globe and influences weather patterns. This understanding contributes to climate science and marine ecology. The key characteristic of ocean circulation is its vast scale, which integrates complex interactions between wind, temperature, and salinity.
One unique challenge with ocean circulation studies is representing the three-dimensional aspects in models. This complexity can lead to inaccuracies if not addressed properly. Nevertheless, the benefits of comprehending ocean circulation encompass better climate models and improved fishing practices, proving the significance of hydrodynamics in this domain.
Aerodynamics and its Relationship with Hydrodynamics
Aerodynamics explores the behavior of air in motion, often sharing principles with hydrodynamics. Both fields study fluid motion, but focus on different media—liquids for hydrodynamics and gases for aerodynamics. Understanding this relationship can lead to advancements in various applications, such as optimizing vehicle performance and developing more efficient aircraft designs.
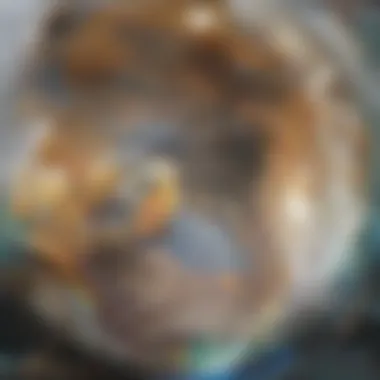
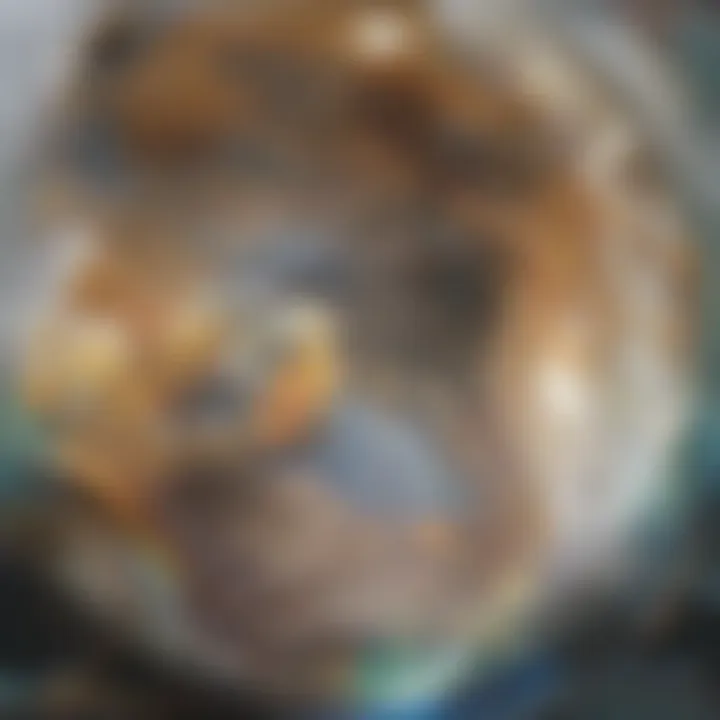
The interplay between aerodynamics and hydrodynamics illustrates the universal laws of fluid behavior. Such insights empower engineers and designers to create solutions that meet the demands of both land and sea.
"The boundaries between aerodynamics and hydrodynamics often blur, highlighting a fascinating aspect of fluid dynamics that is essential for innovation across many fields."
Recent Advances in Hydrodynamics
Recent advances in hydrodynamics play a crucial role in the continued evolution of fluid dynamics research and its applications. The field has seen significant developments that enhance understanding of complex behaviors of fluids and improve practical engineering solutions. These advances are especially important in addressing the challenges posed by new materials and current environmental concerns. This section will focus on two key areas: Computational Fluid Dynamics and Smart Materials and Fluid Interaction. Both areas reflect the ongoing innovation in hydrodynamics and its interdisciplinary nature.
Computational Fluid Dynamics
Computational Fluid Dynamics (CFD) has become a cornerstone in the study of hydrodynamics. It employs numerical analysis and algorithms to solve and analyze problems involving fluid flows. The growth of computational power has allowed for the simulation of various fluid behaviors that were previously too complex to model accurately.
- Precision and Predictive Power: CFD enables detailed predictions of fluid behavior under various conditions. Engineers can simulate scenarios that give insight into performance and safety in engineering projects, such as in aerodynamics or hydrodynamic design.
- Applications Across Fields: The influence of CFD stretches across multiple domains including aerospace, marine engineering, and even medical applications where fluid dynamics is critical.
- Integration with Machine Learning: Recent trends in CFD include integrating machine learning algorithms. This has enhanced prediction capabilities and reduced computational time. By processing vast amounts of data, these algorithms can identify patterns that humans may overlook.
"The growth of Computational Fluid Dynamics has democratized access to complex fluid simulations, putting advanced tools in the hands of engineers and researchers worldwide."
Smart Materials and Fluid Interaction
The study of smart materials and their interaction with fluids presents another exciting frontier in hydrodynamics. These materials, which can change properties in response to external stimuli (like temperature, electric field, or pressure), offer new possibilities in the design of systems that require precise fluid control.
- Adaptive Responses: Such materials can adapt their behavior in real-time to optimize fluid flow. For instance, using smart materials in pumps or valves may lead to enhanced efficiency and performance.
- Environmental Impact: The use of smart materials can also contribute to sustainability by optimizing energy usage and reducing waste in fluid transport systems.
- Research and Development Opportunities: Ongoing research in this area seeks to explore new combinations of materials and the effects on fluid characteristics. Researchers are investigating how these materials can be deployed in varied environments, including extreme conditions.
Challenges and Future Directions
The field of hydrodynamics faces several challenges as it evolves. These challenges not only affect researchers but also engineers and environmental scientists who rely on fluid dynamics for a variety of applications. Understanding these challenges can shape future research directions, push the boundaries of technology, and influence sustainable practices.
Complex Fluid Behaviors
Complex fluids exhibit behaviors that do not conform to traditional Newtonian principles. Examples include polymer solutions, colloidal suspensions, and biological fluids. The non-linear dynamics in these materials can lead to unexpected results in flow behavior. Researchers are increasingly focused on unraveling the complexities of these fluids through experimental and computational techniques.
Some key areas of concern include:
- Viscoelasticity: Many complex fluids display both viscosity and elasticity, affecting flow dynamics. Understanding how to model these behaviors is critical.
- Phase transitions: Fluid properties can change under temperature or pressure alterations. This can drastically alter performance in processes like drug delivery or food manufacturing.
- Turbulence: Predicting turbulent flow in complex fluids remains a significant hurdle. Traditional models often fail in such cases, necessitating innovative solutions.
As we delve deeper into these complex fluid behaviors, advancements in computational methods and experimental tools will be necessary to truly comprehend interaction at the microscopic level.
Sustainability and Hydrodynamic Design
Sustainability is becoming a vital consideration in hydrodynamic design. Engineers are tasked with creating systems that minimize environmental impact while maintaining performance. This intersection of hydrodynamics and sustainability presents unique challenges and opportunities.
Key considerations include:
- Energy-efficient designs: Optimizing hydrodynamic systems for lower energy consumption is crucial. This includes the design of efficient propellers, turbines, and pumps that can operate effectively while reducing energy use.
- Environmental impact assessments: Projects must consider how hydrodynamic systems can affect local ecosystems. This involves understanding sediment transport, erosion, and changes in water quality.
- Innovative materials: Using smart materials that respond to environmental conditions or fluid behaviors can enhance sustainability. These materials can minimize waste and improve the longevity of hydrodynamic systems.
Emphasizing sustainability in hydrodynamic design is a forward-looking approach, necessary for integrating environmental stewardship with practical engineering solutions. It aligns with broader societal goals and ensures the longevity of ecosystems while supporting technological advancements.
"Hydrodynamics must evolve to embrace sustainability, combining the need for efficiency and environmental responsibility."
Finale
The conclusion of this article encapsulates the integral role of hydrodynamics in both theoretical and practical domains. It emphasizes how understanding fluid behavior not only advances scientific knowledge but also enhances engineering applications. Hydrodynamics merges principles of physics, mathematics, and engineering, creating an essential foundation for students, researchers, and professionals. The significance of hydrodynamics is evident in various real-world applications, such as environmental monitoring, ship design, and the development of advanced fluid measurement instruments.
Summary of Key Insights
This article highlights central themes in hydrodynamics. Each section provided detailed insights into:
- Fluid Properties: Understanding how density, viscosity, and surface tension affect fluid motion.
- Fundamental Equations: The importance of equations such as Bernoulli’s and Navier-Stokes in predicting fluid behavior.
- Experimental Techniques: Various methods for visualizing and measuring fluid flow.
- Common Applications: An overview of how hydrodynamics applies to engineering and environmental sciences.
- Recent Advances: Discussion of computational fluid dynamics and smart materials.
- Challenges and Future Directions: Examination of complex fluid behaviors and sustainable designs.
This narrative brings together the essence of hydrodynamics, showcasing how it influences many fields of study.
Implications for Future Research
Looking ahead, research in hydrodynamics holds promising avenues for exploration. Future studies could consider:
- Developing methodologies that apply advanced computational techniques to address complex fluid interactions.
- Investigating the effects of climate change on river hydraulics and ocean circulation patterns.
- Enhancing the integration of smart materials into fluidic systems for improved efficiency.
- Examining sustainable designs in hydrodynamics, focusing on eco-friendly engineering solutions.
Such research could lead to breakthrough technologies that not only improve our understanding of fluid dynamics but also address pressing global challenges, making your contributions valuable in advancing the field.