Exploring Hydrophobic Membranes in Science
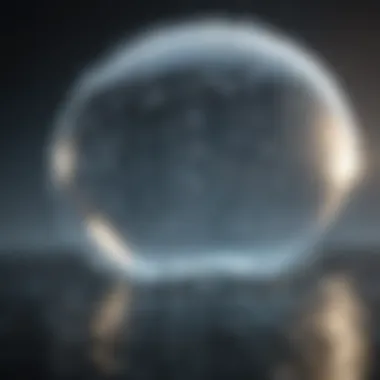
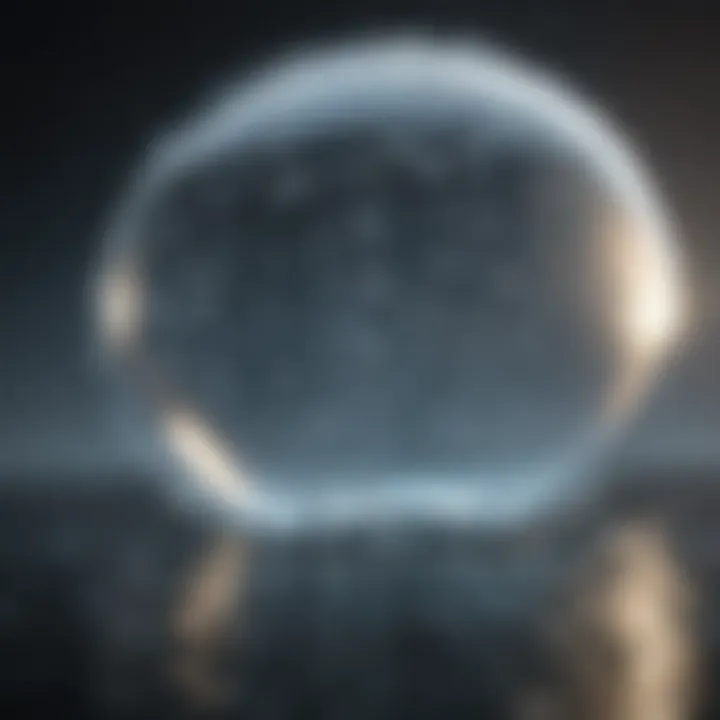
Intro
Hydrophobic membranes are fascinating materials with unique properties that play critical roles in several scientific and industrial applications. These membranes repel water, but allow the passage of gases or organic vapors. This study aims to analyze various aspects of hydrophobic membranes, from their formation to their significance in multiple disciplines like biology, chemistry, and material science.
The importance of hydrophobic membranes lies in their functionality. They are utilized for filtration, separation, and in many advanced technologies. Understanding their mechanisms is essential for researchers and professionals who aim to develop new applications or improve existing ones. As this article unfolds, we will explore the physical properties, formation processes, and applications of these membranes in detail.
Research Overview
Summary of Key Findings
Hydrophobic membranes exhibit distinct characteristics, such as high permeability for specific gases while blocking liquid water. Their effectiveness in applications like water purification and gas separation is notable. The research underscores the advancements in membrane technology, including novel materials and fabrication methods.
Background and Context
The study of hydrophobic membranes spans several decades, gaining momentum as industries seek sustainable solutions for filtration and environmental preservation. Researchers are increasingly focusing on the development of more efficient membranes tailored for specific applications. This trend reflects the growing concern for environmental challenges and the demand for innovative solutions in diverse fields.
Properties of Hydrophobic Membranes
Hydrophobic membranes are defined by several key properties that influence their functionality:
- Water Repellency: The core feature is their ability to prevent water from passing through while allowing the permeation of gases.
- Selective Permeability: These membranes can be engineered to selectively allow certain substances, making them suitable for various applications.
- Mechanical Strength: Hydrophobic membranes must maintain structural integrity under varying pressures and conditions.
This combination of properties makes hydrophobic membranes ideal candidates for applications in areas such as biotechnology and environmental engineering.
Formation Processes
Understanding how hydrophobic membranes are synthesized is crucial. The formation usually involves:
- Phase Inversion: This common method involves transforming a polymer solution into a solid membrane through a phase change.
- Electrospinning: A more advanced technique that produces fine fibers, enhancing membrane surface area and properties.
These processes can significantly affect the performance of the membranes produced.
Applications
Hydrophobic membranes find use in many fields:
- Water Treatment: Efficient at filtering contaminants from water resources.
- Gas Separation: Used in processes like carbon capture and gas purification.
- Medical Applications: Employed in various filtration and separation processes within healthcare settings.
These applications reveal the practicality of hydrophobic membranes in solving real-world problems.
"The development of hydrophobic membranes represents a pivotal advancement in material science, offering solutions that were previously unattainable."
Future Directions
Ongoing research focuses on enhancing the efficiency and selectivity of hydrophobic membranes. Future work aims to:
- Develop membranes from sustainable materials.
- Improve the scalability of manufacturing processes.
- Investigate new applications in energy production and storage.
Understanding these future directions will drive innovations and improve the effectiveness of hydrophobic membrane technology.
This article serves to provide an in-depth look into the world of hydrophobic membranes, paving the way for those interested in advancing their application and understanding of this vital field.
Preface to Hydrophobic Membranes
Hydrophobic membranes play a crucial role in various scientific domains. Their unique properties make them valuable in applications ranging from filtration technologies to biological systems. Understanding these membranes is important due to their ability to selectively allow or restrict the passage of fluids based on their characteristics. This introduction sets the stage for a thorough analysis of hydrophobic membranes, elucidating the benefits and considerations tied to their study.
When we refer to hydrophobic membranes, we typically mean those that repel water while allowing certain other substances to pass through. The implications of this characteristic are far-reaching. For instance, in a biological context, hydrophobic membranes can be crucial for cellular functions, delineating intracellular spaces, and regulating nutrient transport.
In industrial applications, these membranes facilitate processes that require specific separation of liquids without the interference of water, enhancing efficiency in sectors such as wastewater treatment and chemical processing. Furthermore, the development and research surrounding these membranes have evolved over decades, illustrating the growing recognition of their importance.
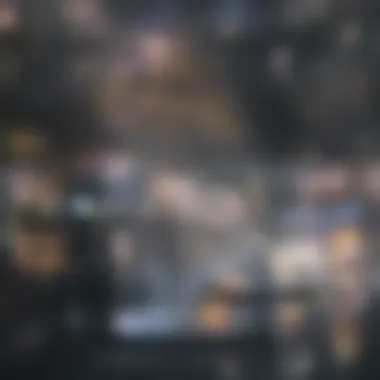
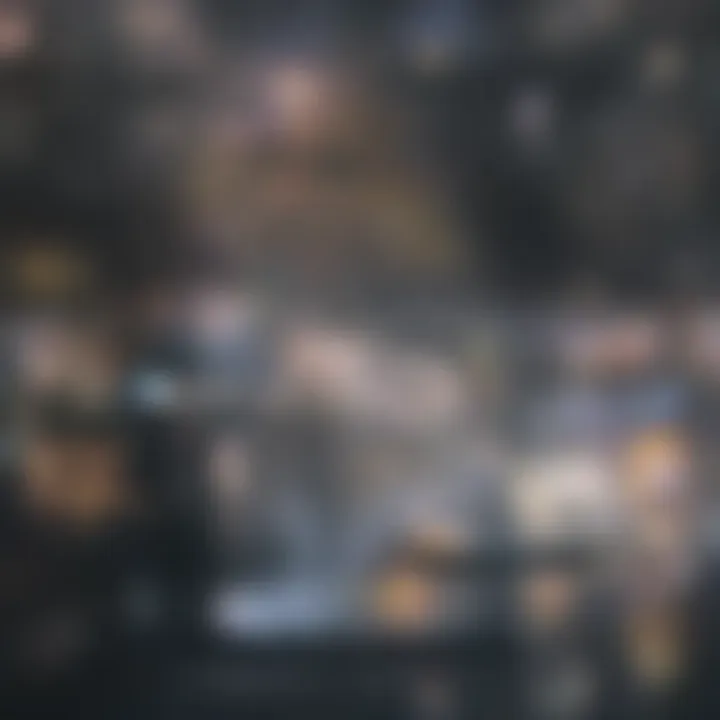
Key Considerations
- Applications: From healthcare to environmental engineering.
- Design: How design affects functionality and application.
- Research: Emerging technologies and methodologies.
Advancing our understanding of hydrophobic membranes opens doors to innovative solutions in numerous fields. This article continues by exploring the definition and characteristics that define these specialized membranes.
Fundamental Properties of Hydrophobic Membranes
Understanding the fundamental properties of hydrophobic membranes is essential for grasping their wide range of applications and significance in various fields. These membranes exhibit unique characteristics, prominently defined by their hydrophobic nature. This property is crucial in controlling interactions with water and various solvents, impacting how they function in practical applications. Moreover, the physical structure and composition of these membranes determine their efficiency and performance across diverse scenarios.
Hydrophobicity Explained
Hydrophobicity refers to the tendency of a material to repel water. In hydrophobic membranes, this characteristic is largely due to the presence of non-polar functional groups in their molecular structure. Commonly observed in materials such as polytetrafluoroethylene (PTFE) and polyethylene, hydrophobic membranes prevent water molecules from permeating through them. This repulsion is significant for applications where moisture resistance is crucial, such as in protective clothing and filters.
The extent of hydrophobicity can be quantified using the contact angle measurement. A contact angle greater than 90 degrees indicates hydrophobic behavior, reflecting how well the surface can repel water. Understanding hydrophobicity informs several practical applications, from the design of waterproof fabrics to enhancing the efficiency of oil-water separation technologies.
Hydrophobic membranes provide superior water resistance, making them instrumental in designing filters and barriers in numerous industries.
Physical Structure and Composition
The physical structure and composition of hydrophobic membranes directly correlate with their functionality. These membranes are often composed of polymer-based materials that can be engineered to enhance their hydrophobic properties. The arrangement of polymer chains influences properties such as porosity, thickness, and mechanical strength. Typically, the membranes can be manufactured using various methods, including extrusion, phase inversion, and electrospinning.
Each manufacturing technique leads to different structural arrangements, which can affect the overall performance. For instance, electrospun membranes demonstrate high surface area and fine fiber morphology, essential for filtration applications.
Materials like polyvinylidene fluoride (PVDF) or PTFE are frequently selected for their stability, durability, and thermal resistance.
In summary, the fundamental properties of hydrophobic membranes play a vital role in their effectiveness for numerous applications. An in-depth understanding of hydrophobicity and the associated physical structures informs the advancements and innovations seen in this area of technology.
Mechanisms of Hydrophobic Membrane Function
The exploration of hydrophobic membrane function reveals a critical understanding of how these structures operate at a microscopic level. These mechanisms play a substantial role in numerous applications, from filtration to chemical separation, making them essential in both industrial and biological contexts. Understanding how these membranes function informs their implementation in various technologies, leading to enhanced performance and efficiency.
Fluid Transport Mechanisms
Fluid transport through hydrophobic membranes hinges on the principle of selective permeability. Such membranes allow only specific molecules to pass while blocking others, depending on their size and chemical nature. This selectivity benefits processes like water purification and drug delivery.
- Diffusion: Molecules move from areas of high concentration to low concentration, utilizing the selective barriers of the membranes. This passive transport does not require energy and is critical in processes such as osmosis.
- Convective flow: In some applications, forced flow of fluids can enhance transport efficiency. Here, pressure gradients drive molecules through the membrane, increasing throughput in filtration systems.
- Active transport: Certain membranes employ energy to move substances against their concentration gradients, enabling the selective uptake of necessary nutrients or removal of waste.
Understanding these transport mechanisms aids researchers and engineers in optimizing the designs and applications of hydrophobic membranes.
Interaction with Solvents
The interaction of hydrophobic membranes with solvents is vital for their performance and stability. Unlike hydrophilic membranes that readily engage with water, hydrophobic membranes exhibit resistance to such interaction. This property allows them to repel water while allowing specific organic solvents or gases to pass through.
- Solvent permeability: The choice of solvent directly influences the membrane's ability to allow certain molecules through. For example, membranes designed to interact primarily with organic solvents are essential in chemical separation processes.
- Phase separation: When a hydrophobic membrane interacts with a solvent, it can lead to the formation of distinct phases, affecting the effectiveness of separation techniques.
- Stability issues: The choice of solvent also raises concerns regarding membrane integrity. Compatibility between hydrophobic material and solvent is crucial to prevent degradation or performance loss.
The careful design and selection of hydrophobic membranes based on solvent interactions can greatly enhance their effectiveness in applications such as filtration, extraction, and drug delivery.
Formation Processes of Hydrophobic Membranes
Understanding the formation processes of hydrophobic membranes is critical in the field of material science and engineering. These processes determine the functional capabilities of the membranes and influence their performance in practical applications. The right formation technique not only impacts the efficiency of the membranes but also their longevity and versatility. This section will discuss key synthesis techniques and self-assembly processes, highlighting their significance, benefits, and considerations.
Synthesis Techniques
Synthesis techniques are essential for creating hydrophobic membranes tailored for specific applications. Some commonly used methods include:
- Phase Inversion: This involves dissolving a polymer in a solvent, followed by the introduction of a non-solvent, causing phase separation. The resultant membrane structure is highly porous and inherently hydrophobic due to the polymer's characteristics.
- Electrospinning: This technique allows for the creation of nanofiber membranes, which can provide enhanced surface area and filtration properties. The fibers can be collected onto a substrate, forming a non-woven membrane that can efficiently repel water.
- Layer-by-Layer Assembling: This process utilizes alternating layers of positively and negatively charged materials to create multilayer membranes. The hydrophobic nature can be introduced through the choice of polymers or surfaces at different stages of assembly.
Each method has its advantages and challenges. For instance, phase inversion tends to produce membranes with uniform thickness, while electrospinning offers flexibility in fiber diameter, which can be advantageous for specific applications in filtration or protective barriers.
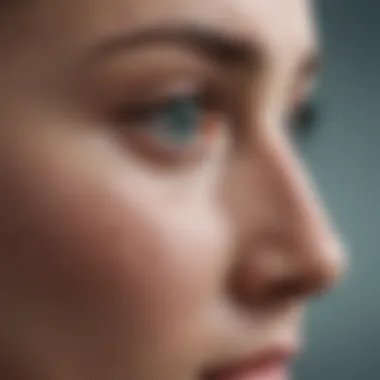
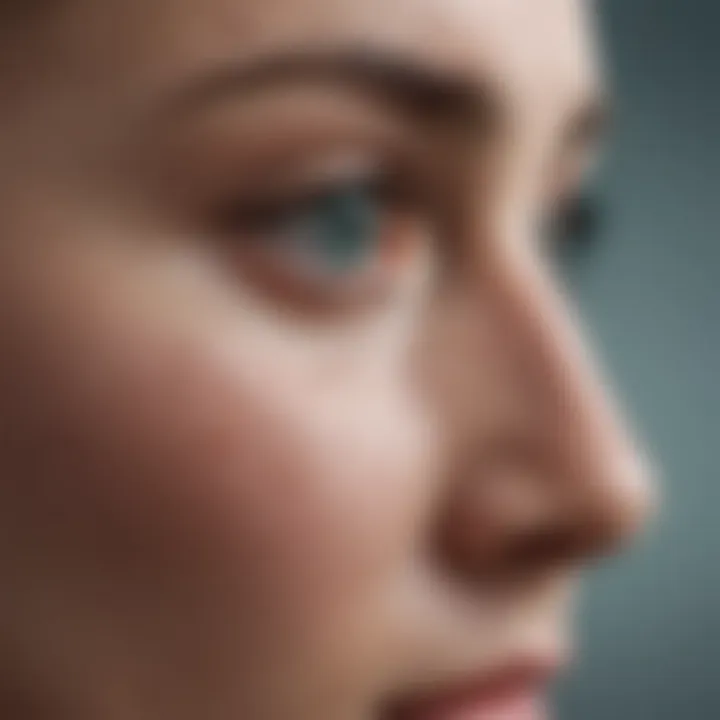
Self-Assembly Processes
Self-assembly is a naturally occurring process critical for forming hydrophobic membranes without extensive external manipulation. This approach utilizes the intrinsic properties of molecules to organize themselves into structured formations. Key self-assembly processes include:
- Self-Organizing Monolayers: Molecules that possess hydrophobic tails can spontaneously organize at interfaces. This leads to the formation of a monolayer that acts as a barrier against water, effectively creating a hydrophobic surface.
- Block Copolymer Self-Assembly: Block copolymers can phase-separate into different domains. When these domains consist of hydrophobic segments, they can be arranged to create membranes with defined structures at the nanoscale.
- Biomimetic Approaches: Drawing inspiration from nature, self-assembly processes mimic natural systems like cellular membranes. These processes can lead to membranes with unique properties, enhancing their performance in biological applications.
Self-assembly methods present an eco-friendly alternative, as they often require less energy and fewer resources compared to traditional fabrication methods. They result in membranes with unique structures that exhibit superior water-repellent properties.
"The formation processes of hydrophobic membranes intertwine chemistry and engineering, producing materials that fulfill diverse roles across industries."
Characterization of Hydrophobic Membranes
The characterization of hydrophobic membranes is crucial for understanding their performance across various applications. These membranes play an essential role in filtration, separation technologies, and many biological processes. By identifying their characteristics, researchers can optimize their design and performance for specific tasks. In this section, we will elaborate on two primary aspects: analytical techniques used for characterization and performance assessment metrics.
Analytical Techniques Used
Characterization of hydrophobic membranes involves several analytical techniques that provide insights into their structure and properties. Here are some key methods:
- Scanning Electron Microscopy (SEM): This method offers high-resolution images of membrane surfaces. SEM helps in observing the morphology and surface structure of hydrophobic membranes, crucial for understanding their performance.
- Atomic Force Microscopy (AFM): AFM provides surface topography at the nanoscale level. This technique is valuable for characterizing roughness and surface features, which directly affect wetting properties.
- Contact Angle Measurement: This technique measures the angle formed between the membrane surface and a droplet of water. This is critical for quantifying hydrophobicity, indicating how a membrane will behave in various conditions.
- Fourier Transform Infrared Spectroscopy (FTIR): FTIR analyzes the chemical composition of membranes. It helps in identifying functional groups and confirming the successful synthesis of hydrophobic materials.
- Porosimetry: Techniques such as mercury intrusion or nitrogen adsorption provide data on pore size distribution. These measurements are key to understanding permeability and separation performance.
Each of these techniques offers unique insights, enabling researchers to fully characterize hydrophobic membranes and tailor their properties for optimal applications.
Performance Assessment Metrics
Performance assessment of hydrophobic membranes requires specific metrics to evaluate their efficiency and suitability for applications. Some of these metrics include:
- Flux Rate: This measures the volume of fluid that passes through a membrane per unit area over time. It is a critical indicator of membrane efficiency. Higher flux rates often correlate with better performance.
- Rejection Rate: This metric evaluates the membrane's ability to exclude specific contaminants or particles. A high rejection rate is desirable for applications in water purification and filtration.
- Selectivity: This refers to the membrane's ability to separate specific molecules based on size or chemical properties. High selectivity enhances the effectiveness of membrane processes.
- Durability: The longevity and stability of membranes under operational conditions are vital. Durability tests help ensure membranes can withstand harsh environments.
- Cost-effectiveness: This metric assesses the economic viability of using a specific hydrophobic membrane in industrial applications. It takes into account factors such as material costs and energy consumption.
Applications of Hydrophobic Membranes
Hydrophobic membranes play a crucial role in numerous fields. Their distinct properties allow them to perform effectively in various conditions. These membranes provide solutions for both biological needs and industrial processes, making them versatile materials in modern technologies. Understanding their applications helps to appreciate their importance and their impact on different industries.
Biological Applications
In the biological field, hydrophobic membranes are integral to various processes. They are commonly used in biosensors, drug delivery systems, and separation processes involving biological fluids. Here are some specific applications in biology:
- Filtration of biomolecules: Hydrophobic membranes are employed to separate proteins and nucleic acids. The right diameter and pore size can allow specific molecules to pass while retaining others.
- Biocompatible materials: Many hydrophobic membranes are designed to be biocompatible, making them suitable for medical implants or devices. This feature is crucial in ensuring that the body does not reject these materials.
- Tissue engineering: These membranes can offer scaffolding for cell growth. They provide a structure that allows cells to adhere and proliferate in controlled conditions, essential for developing tissue constructs.
"The adaptability of hydrophobic membranes makes them effective in various biological applications, ensuring functionality and compatibility with biological systems."
Moreover, in diagnostic applications, hydrophobic membranes facilitate the detection of pathogens, enabling rapid response in clinical settings. This versatility affirms their significance in advancing biomedical technologies.
Industrial Applications
The industrial applications of hydrophobic membranes are equally impressive. They are widely used in sectors such as water treatment, food processing, and chemical manufacturing. Some notable industrial applications include:
- Water purification: These membranes can effectively filter out contaminants from water. They are particularly useful in desalination processes, where they help remove salts and impurities from seawater.
- Gas separation: Hydrophobic membranes are effective in separating gases, which is essential in many industrial processes. For example, they can be used to remove carbon dioxide from natural gas or biogas produced in landfills.
- Food and beverage industry: In this sector, these membranes help in the filtration and clarification of beverages, removing unwanted particulates without affecting the product's flavor or quality.
- Chemical reactors: Hydrophobic membranes assist in creating reactive environments for certain chemical reactions. By controlling the transport of gases and liquids, they help optimize reaction conditions.
The applications highlighted above demonstrate that hydrophobic membranes are not just useful but also indispensable in advancing industrial processes and improving product quality. Their impact across disciplines underscores their versatile nature and potential for future innovations.
Role in Filtration Technologies
Hydrophobic membranes play a crucial role in filtration technologies across multiple industries. Their unique properties allow them to selectively allow certain substances to pass while blocking others. This selectivity is central to various filtration applications, making them essential in processes ranging from water purification to air filtration and even industrial separations.
Membrane-Based Filtration
Membrane-based filtration involves using hydrophobic membranes to separate materials based on size, charge, or chemical affinity. These membranes effectively manage fluid dynamics, making them valuable in various scenarios such as wastewater treatment, food and beverage processing, and pharmaceuticals.
The key benefits of membrane-based filtration include:
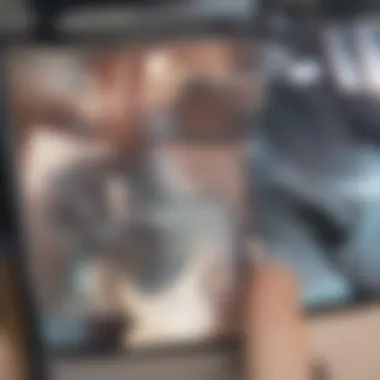
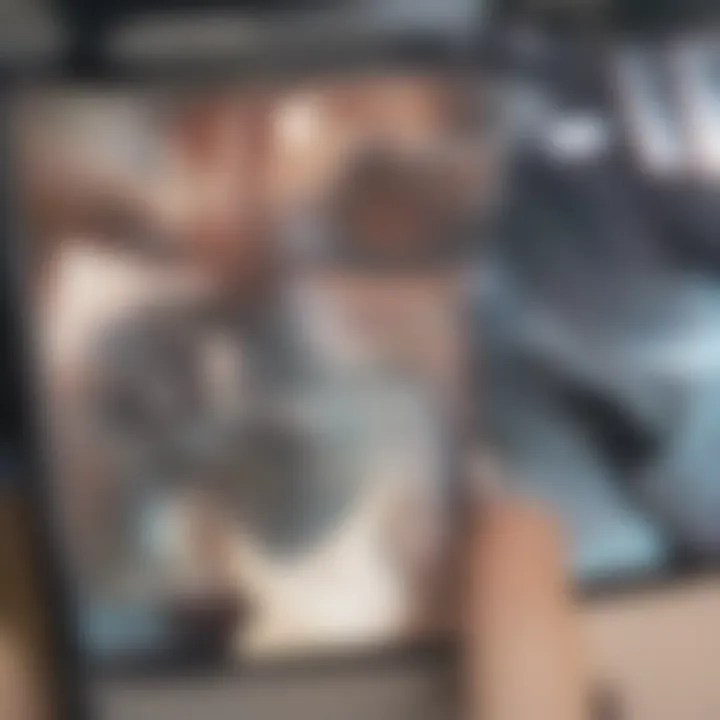
- Efficient Separation: Hydrophobic membranes can separate water from oils and other solvents, which is vital for many industrial processes.
- Energy Efficiency: Many membrane filtration systems require less energy compared to traditional separation techniques, reducing operational costs.
- Scalability: Membrane technology can be easily scaled up or down, allowing for flexibility in application.
In practice, industries utilize various types of hydrophobic membranes based on their specific needs. For example, microfiltration and ultrafiltration membranes are commonly employed to target particulate matter, while nanofiltration membranes are suitable for smaller solute separation.
Environmental Applications
Hydrophobic membranes also have significant uses in environmental applications. They are pivotal in addressing issues like water scarcity and pollution. Applications in this domain include:
- Desalination: Hydrophobic membranes are instrumental in processes that convert seawater into potable water. They allow for the transport of water while effectively rejecting salts.
- Oil-Water Separation: In environmental remediation, these membranes serve to recover valuable oils from water, crucial after oil spills or during industrial processes.
- Air Filtration: In air quality management, hydrophobic membranes can remove volatile organic compounds and other pollutants from the air.
"The versatility of hydrophobic membranes in filtration technologies demonstrates their potential to address contemporary environmental challenges effectively."
Impact on Material Science
The exploration of hydrophobic membranes presents significant implications for material science. These membranes, characterized by their ability to repel water, have transformed various applicationsโfrom water purification systems to advanced biomedical devices. Their unique properties raise intriguing considerations about the materials used in their fabrication.
Material composition plays a crucial role in dictating the performance and efficiency of hydrophobic membranes. High-performance membranes often utilize polymers such as polytetrafluoroethylene (PTFE) or polyvinylidene fluoride (PVDF). These materials are favored for their excellent durability and chemical resistance, making them ideal for diverse applications. The careful selection of these materials impacts not only the functionality but also the long-term stability of the membranes.
Moreover, understanding the interaction between hydrophobic membranes and different substances provides insights into their structural integrity. The fine-tuning of surface characteristics, through methods such as surface modification or incorporation of nanoparticles, can enhance their hydrophobic properties and increase resistance to fouling.
However, the journey is not without its challenges. The engineering aspects of developing these membranes are complex. Designing membranes that can effectively filter specific contaminants while maintaining flow rates is a notable engineering challenge. Balancing these factors requires extensive research and innovative approaches.
As the field of material science continues to evolve, hydrophobic membranes stand at the forefront, highlighting the interplay between material selection and application efficacy.
The ongoing advancements in material chemistry and synthesis methods play a vital role in overcoming these engineering challenges. Researchers are actively exploring new composite materials and hybrid structures that may improve performance metrics while reducing costs. As knowledge expands, the potential for novel applications of hydrophobic membranes is vast, promising to push the boundaries of current technologies.
Future Directions in Hydrophobic Membrane Research
Future directions in hydrophobic membrane research are crucial for understanding how these materials can evolve in response to the changing needs in various fields. As scientific needs and technological landscapes shift, the focus on innovative applications and research initiatives is becoming increasingly pertinent. This exploration can potentially lead to advances that enhance performance, increase efficiency, and open new horizons for hydrophobic membranes in practical uses.
The development of novel hydrophobic membranes can enhance filtration processes, making them more effective against a broader range of contaminants. This importance is reflected not only in their application in industrial use but also in environmental sustainability. As pollution and resource management become global priorities, improvements in hydrophobic membranes offer avenues to address these challenges. As technology advances, understanding the integration of these membranes with existing systems is vital.
Innovative Applications on the Horizon
Recent advancements in material science have led to the exploration of hydrophobic membranes in diverse sectors. One noticeable trend is their potential use in energy-efficient desalination processes. Desalination is critical considering the increasing demand for fresh water; thus, membranes that can effectively separate salt and organic matter without excess energy consumption are highly sought after.
Another promising area is in medical applications. Hydrophobic membranes can provide solutions for biosensors and drug delivery systems. Highly selective membranes can effectively control the release of pharmaceuticals while minimizing side effects. This creates new pathways for targeted therapies and personalized medicine.
Moreover, developments in nanotechnology might allow hydrophobic membranes to be functionalized with nanomaterials. Such innovations could lead to enhanced performance in pollutant removal and increased durability under challenging conditions.
Potential Research Initiatives
To realize the full potential of hydrophobic membranes, several research initiatives require attention. One initiative could involve the investigation of the scalability of novel synthesis techniques. It is vital to design methods that allow for mass production without sacrificing the unique properties of the membranes.
Furthermore, comprehensive studies into surface modification techniques might yield new insights. Understanding the relationship between membrane surface characteristics and their filtration capabilities could lead to performance enhancements that cater to specific applications.
Research focused on the longevity and sustainability of hydrophobic membranes is also necessary. This includes assessing the degradation processes and lifespan of membranes in various environments.
Lastly, fostering collaboration between disciplines such as chemistry, biology, and engineering can create a multidisciplinary approach that bolsters innovative research.
Epilogue
The conclusion of this article serves as a significant synthesis of the multifaceted topic of hydrophobic membranes. Throughout the discussion, we have uncovered their fundamental properties, formation processes, and an array of applications in various scientific fields.
Hydrophobic membranes play a critical role in filtration technologies and biological applications. These membranes demonstrate remarkable capabilities in separating liquids and gases by leveraging their unique properties, which also make them highly suitable for a range of industrial applications.
Importance of Understanding Hydrophobic Membranes
Understanding hydrophobic membranes is essential for several reasons:
- Innovation in Technology: Research into hydrophobic membranes continues to yield innovative applications. This includes advancements in water purification and areas such as biotechnology.
- Interdisciplinary Relevance: The significance of hydrophobic membranes stretches beyond a single field, affecting disciplines such as chemistry, biology, and materials science. As technology evolves, these membranes can lead to breakthroughs across these areas.
- Future Research Potential: Identifying future directions in this field can help prioritize research initiatives. This can enhance our understanding of material properties and improve existing technologies.
"The study of hydrophobic membranes opens avenues for advancements that could change our approach to material sciences and environmental technologies."
Careful consideration of the engineering challenges associated with these membranes is also necessary to maximize their effectiveness and usability.
In summary, hydrophobic membranes are not just a novel material; they represent a pivotal research area that can contribute significantly to scientific progress. Engaging with this field encourages further discovery and innovation that are essential for addressing current and future challenges in various sectors.