Exploring Kelvin Probe Force Microscopy: Principles and Applications
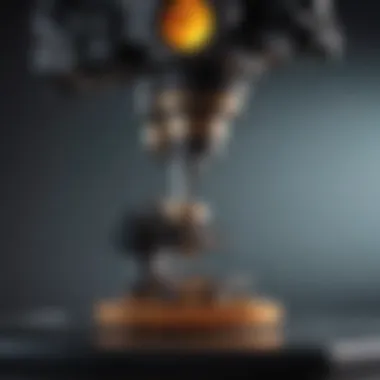
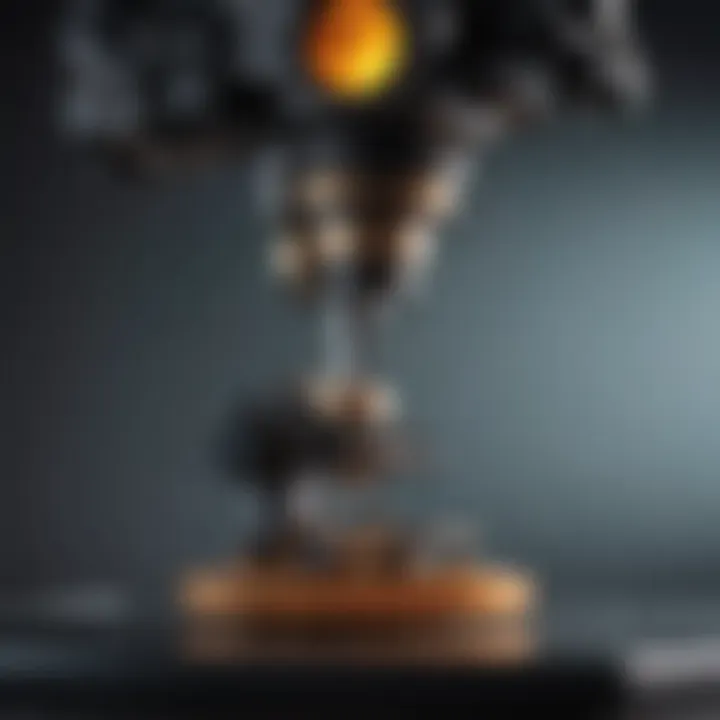
Intro
Kelvin Probe Force Microscopy (KPFM) stands as an advanced technique that bridges electrical measurement with high-resolution atomic force microscopy. This method is essential in understanding electrical properties of materials at a nanoscale. It provides insights into surface potential, work function variations, and other significant characteristics that influence material behavior, particularly in areas like nanotechnology and materials science.
The following sections will delve into the foundational principles of KPFM, its methodological aspects, and its diverse applications in scientific research. This inquiry aims to highlight how KPFM can elucidate complex surface phenomena, thus enriching our comprehension of various materials.
Research Overview
Summary of Key Findings
KPFM has evolved significantly, with advancements enhancing measurement precision and speed. Recent studies show its utility in measuring surface charge distributions in nanostructures. The findings emphasize:
- The accuracy of KPFM in mapping electronic properties at nanoscale.
- Applications in characterizing semiconductor materials.
- Insights into electrostatic forces within functionalized surfaces.
Background and Context
Developed from the principles of scanning tunneling microscopy and atomic force microscopy, KPFM utilizes a conductive probe to detect surface potential differences. This technique combines topographical imaging with simultaneous potential measurement, allowing comprehensive surface studies. It has gained traction since its inception in the 1990s, providing groundbreaking insights in various fields.
The significance of KPFM extends far beyond mere surface mapping. It finds applications in:
- Material Science: Assisting in the development of new materials with tailored properties.
- Nanotechnology: Offering detailed evaluations of nanoscale devices.
- Surface Chemistry: Enabling understanding of chemical interactions at surfaces.
"KPFM bridges the gap between electrical and spatial measurements, providing insights previously unattainable with conventional techniques."
Methodology
Experimental Design
The KPFM setup typically involves a modified atomic force microscope. A conductive AFM tip scans the surface, measuring the electrostatic forces between the tip and the sample. This interaction provides a correlation between topographic features and the electrical properties of the material under study.
Data Collection Techniques
Data are collected by varying the DC voltage applied to the tip. The resulting changes in current allow for the calculation of electrical potential across the surfaces. KPFM employs:
- Frequency Modulation to enhance sensitivity.
- Phase Detection to accurately map potential differences.
Through these techniques, KPFM provides detailed insights into the surface electronic structure of materials, significantly contributing to the advancements in research.
Foreword to Kelvin Probe Force Microscopy
Kelvin Probe Force Microscopy (KPFM) is an advanced technique crucial for the analysis of surface potential at the nanoscale level. It merges principles of electrostatics and atomic force microscopy to provide insights that are vital across various scientific domains. Understanding KPFM is not only important for researchers but also for industries involved in material science, nanotechnology, and chemistry. This section will outline the historical development of KPFM and the basic principles underpinning its operation.
Historical Background
The origins of Kelvin Probe Force Microscopy trace back to fundamental work in the field of electrostatics, with significant contributions from Lord Kelvin in the late 19th century. The initial idea involved measuring the surface potential of materials without physical contact, allowing a more sensitive exploration of surface phenomena. In the 1990s, the coupling of atomic force microscopy with the concepts of electrostatics allowed for the evolution of KPFM into a powerful tool. This innovation enabled scientists to investigate how surface charges influence the properties of materials at nanoscale dimensions, leading to groundbreaking findings in the characterization of surface states.
Basic Principles of Operation
At the heart of KPFM lies the concept of measuring the contact potential difference between a conductive probe and the sample surface. The process can be broken down into two essential modes:
- Dynamic mode: In this mode, the cantilever holding the probe oscillates above the surface. The electrostatic forces between the probe and sample affect the amplitude of oscillation, which is key for obtaining voltage contrast.
- DC bias: A direct current bias is applied to comprehend the forces present on the probe. By analyzing the changes in the cantilever's oscillation, a precise measurement of the surface potential can be extracted.
This method of operation allows KPFM to distinguish electronic properties and surface heterogeneities that are often invisible in traditional microscopy techniques. The ability to measure local variations enhances understanding of materials, particularly at the nanoscale, opening avenues for exploration in various applications.
Design and Components
The design and components of Kelvin Probe Force Microscopy (KPFM) are fundamental to its function and performance. Understanding these aspects is crucial for researchers and professionals aiming to utilize this technique effectively. The design elements not only determine the sensitivity of the measurements but also influence the specific applications in which KPFM can be employed. A thorough comprehension of these systems enables users to maximize the potential of KPFM in various scientific investigations.
Probe Design and Functionality
The probe is the heart of the KPFM system. Its design impacts the resolution and stability of measurements dramatically and therefore its functionality is paramount. The probe typically consists of a conductive tip that interacts with the surface of a sample. Developers often use materials like gold or platinum, as they offer good electrical conductivity and mechanical strength.
A critical aspect of probe design is its geometry. The sharpness of the tip plays a direct role in the ability to resolve features on the surface. An extremely sharp tip can yield higher spatial resolution, allowing for the detection of small variations in surface potential.
In addition to sharpness, the probe must be designed to maintain stabilization during measurements. This involves minimizing external vibrations and thermal fluctuations. A well-designed probe will support high-resolution imaging while ensuring that measurements reflect true surface properties.
Electronic Components and Systems
KPFM relies heavily on sophisticated electronic components to control the measurement process and to process data accurately. The electronics include a feedback loop designed to maintain a constant force between the probe and the sample. This is critical for ensuring reliable measurements of the surface potential.
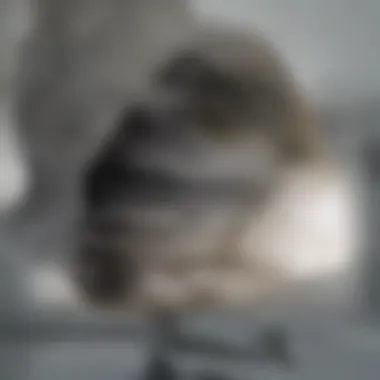
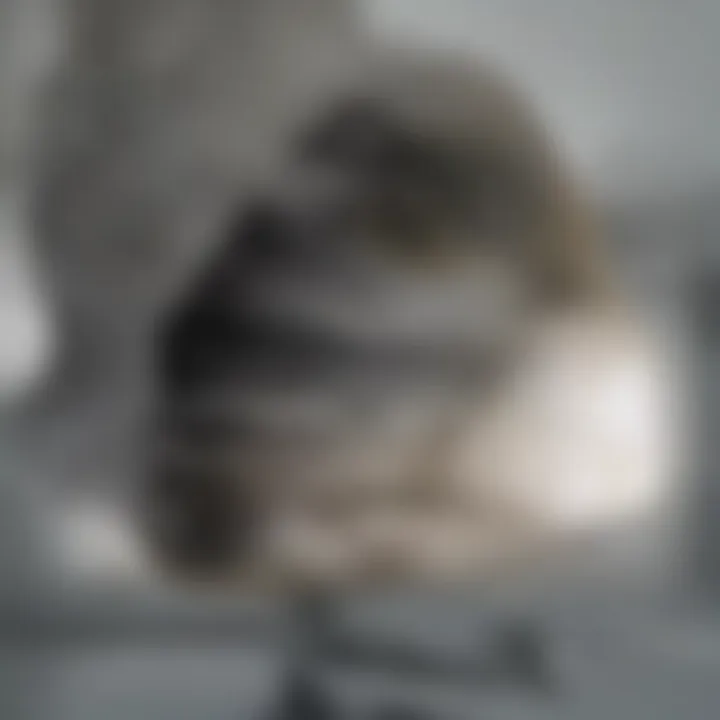
Moreover, the integration of advanced circuitry is essential for processing signals obtained from the probe. The electrical noise must be minimized to improve the accuracy of the readings. Engineers often utilize high-quality amplifiers and filters in their designs to achieve this. The use of digital signal processing techniques is also prevalent to enhance the fidelity of the acquired data.
Various configurations may include an atomic force microscopy setup, which provides mechanical feedback in addition to electronic conditioning of the measurements.
Environmental Control Systems
The environmental conditions in which KPFM operates can greatly influence its measurements. Factors such as temperature, humidity, and ambient vibrations can introduce noise and uncertainty in the data collected. To combat this, KPFM systems are often equipped with environmental control systems.
These systems may include temperature stabilization units and vibration isolation platforms. By regulating the temperature, researchers can minimize thermal drift in measurement readings. Vibration isolation platforms prevent physical disturbances from affecting the precision of the probe’s movements, thereby improving the overall reliability of the technique.
Incorporating environmental control not only enhances the accuracy of KPFM but also expands its applicability in sensitive research areas. This capability allows researchers to explore a broader range of materials and surface phenomena under varied conditions.
Investing time in understanding the design and components of KPFM can significantly enhance its effectiveness in research applications.
In summary, the design and functionality of the probe, along with the electronic systems and environmental controls, form the backbone of KPFM. Each element plays a critical role in ensuring the technique's reliability and precision, ultimately contributing to advancing knowledge in various scientific fields.
Measurement Techniques and Methodologies
Measurement techniques and methodologies form the backbone of Kelvin Probe Force Microscopy (KPFM), establishing how surface potential variations are detected and analyzed. Understanding these methodologies is pivotal for optimizing experiments and interpreting results accurately. The effectiveness of KPFM heavily relies on the precision and reliability of the measurement techniques employed. Consequently, this section will clarify the distinctions between various methodologies and the implications of each on KPFM analysis.
Contact Mode vs. Non-Contact Mode
Contact mode and non-contact mode represent two distinct approaches in the application of KPFM. In contact mode, the probe is in direct contact with the sample surface. This allows for high-resolution measurements but can lead to mechanical wear or alteration of the sample. Therefore, the choice for this mode is critical depending on the sample's characteristics. High spatial resolution is one clear benefit of contact mode, making it suitable for detailed surface topography assessment.
On the other hand, non-contact mode employs a different principle. Here, the probe oscillates near the surface without making contact. This reduces the risk of damaging the sample and enables the examination of softer materials. While this mode may sacrifice some resolution, it compensates by acquiring data from broader areas and preserving sample integrity. Understanding the trade-offs between these modes is essential for researchers. Their choice should align with the specific requirements of the study at hand.
Frequency Shift Detection
At the heart of KPFM is the frequency shift detection technique, which uses changes in the cantilever's oscillation frequency to measure the surface potential. This method is sensitive to very minute variations in potential, allowing researchers to gain insights into electronic properties of surfaces at the nanoscale. The ability to detect frequency shifts relies on a precise calibration of the system. When the tip approaches the sample surface, electrostatic forces alter its frequency of oscillation. By measuring this frequency shift, one can draw conclusions about the surface material's electronic properties.
However, it is crucial to consider environmental factors that could affect frequency measurements. External vibrations or stray electric fields may introduce noise into the data, impacting accuracy. This demands that practitioners implement robust signal processing techniques to mitigate such influences.
Scanning Tunneling Microscopy Integration
An increasingly common strategy in KPFM is the integration with Scanning Tunneling Microscopy (STM). When combined, these two techniques provide an unparalleled view of surface features and electronic states. STM can visualize surfaces at atomic resolution, while KPFM can measure electrostatic forces at these same surfaces. The synergy between these techniques enriches the data one can collect, leading to deeper insights into surface phenomena.
However, the integration of KPFM and STM requires careful calibration and alignment of the systems. It also involves navigating the challenges of data complexity. By merging both methodologies, researchers can potentially culminate in a cohesive understanding of nanoscale systems, informing future developments in material science and nanotechnology.
"Combining KPFM with STM allows scientists to bridge the gap between imaging and electrical characterization in a single experiment."
In summary, the methodologies utilized in KPFM play a significant role in data acquisition. By understanding the nuances between contact and non-contact modes, frequency shift detection, and the integration with STM, researchers can tailor their approaches for greater efficacy in their investigations.
Calibration Techniques
Calibration is vital for ensuring the accuracy and reliability of measurements obtained from Kelvin Probe Force Microscopy (KPFM). As a technique that is sensitive to both environmental factors and material properties, obtaining precise data relies heavily on effective calibration procedures. The calibration process establishes a reference point, allowing researchers to interpret their results meaningfully. Without a solid calibration framework, the insights drawn from KPFM measurements may be inaccurate or misleading, which can affect subsequent research or applications.
Standard Calibration Approaches
Several standard calibration approaches are implemented in KPFM to enhance measurement accuracy. These methods typically involve the use of known materials and reference probes to establish the force and potential characteristics of the system. Here are some prevalent approaches:
- Use of Reference Materials: Known conductive materials serve as reference points. Materials like gold or indium tin oxide (ITO) are commonly used. They enable comparison against the probe response, providing a baseline for assessing changes in surface potential.
- Voltage Calibration: This method adjusts the voltage applied to the probe against a known standard. This ensures the measured electric field corresponds accurately to the applied voltage.
- Determining Work Function: The work function of a material is crucial for KPFM operation. Calibration often involves measuring a material with a well-established work function to ensure correct assessment of sample materials.
- Periodic Calibration Checks: Regular checks of the calibration status are essential. These checks help to ensure consistent measurement over time, especially in dynamic environments where instrument conditions may change.
Implementing these calibration strategies allows researchers to attain reliable data from KPFM, enhancing the validity of their findings.
Challenges in Calibration
Calibrating KPFM systems is not without its challenges. Various factors can complicate the calibration process, leading to less accurate results. Here are some notable challenges:
- Environmental Sensitivity: KPFM measurements can be significantly affected by ambient conditions, such as temperature and humidity. These variations can lead to shifts in measurements, making standard calibration approaches less effective without accounting for environmental controls.
- Complex Material Properties: Different materials exhibit unique electrical characteristics. Some materials pose difficulties in establishing a standard calibration since their response may differ from traditional reference materials.
- Aging of Probes: Probes used in KPFM can degrade over time. The materials and coatings on these probes can alter their properties leading to drifting in measurement reliability. Addressing probe condition is essential for accurate calibration.
"Calibration is a continuous process; researchers must remain vigilant about their calibration standards and procedures to ensure the integrity of their KPFM results."
- Technological Limitations: Not all instruments support advanced calibration features. Some may lack the necessary components to conduct thorough calibration, limiting the precision of acquired data.
In summary, understanding and addressing the challenges in KPFM calibration is crucial for obtaining high-quality data. Proper calibration techniques not only enhance measurement accuracy but also ensure that findings in the realm of surface science remain dependable.
Data Acquisition and Analysis
Data acquisition and analysis are crucial in Kelvin Probe Force Microscopy (KPFM). They directly affect the quality and reliability of the measurements obtained, which are essential for drawing valid conclusions from experiments. Proper data acquisition ensures the signal collected is both accurate and reflective of the surface properties being studied. This quality contributes to the potential applications in various fields such as nanotechnology and material science.
The process begins with the transduction of the signal as the probe interacts with the sample. An effective data acquisition system must have a high sampling rate to capture rapid changes in the electrical potential. More importantly, it should minimize noise interference. The calibration of the equipment and the environmental conditions, including temperature and humidity, are also factors that influence the data's integrity.
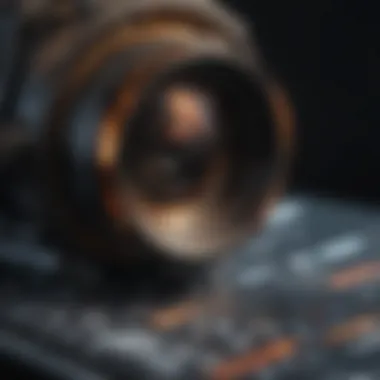
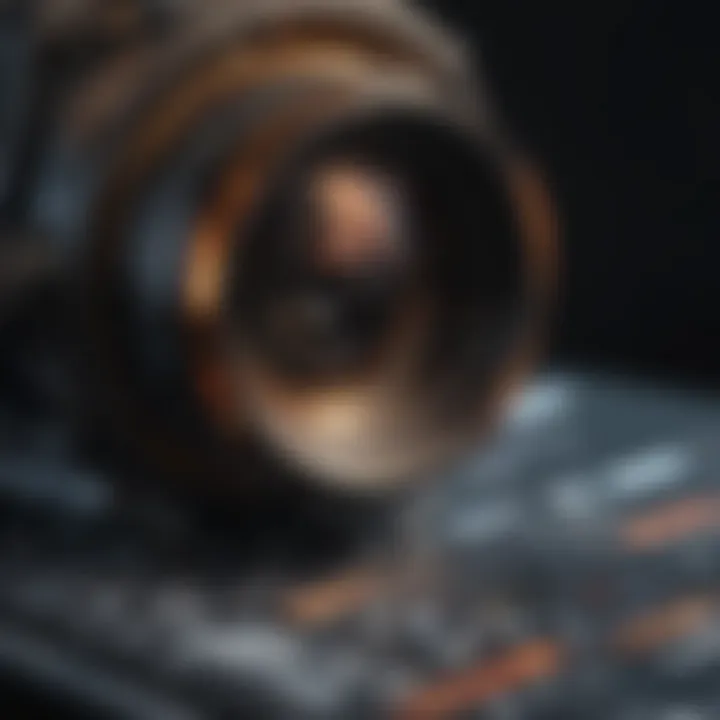
Signal Processing Techniques
Once data is acquired, signal processing techniques play a vital role in enhancing the quality of the information collected. These techniques help to filter out noise and improve signal clarity, making it easier to interpret the results. Common methods include:
- Fourier Transform: This technique converts time-domain data into frequency-domain data, which helps in identifying signal components.
- Wavelet Transform: Useful for analyzing non-stationary signals, this technique allows dissecting signals into various frequency components with better localization.
- Digital Filtering: By applying various filters, researchers can isolate desired signals from background noise.
In KPFM, the choice of signal processing method affects the accuracy of measurement. For instance, improper filtering may lead to misinterpretations of the surface potential, which directly impacts the subsequent analysis.
Image Reconstruction Algorithms
Image reconstruction algorithms are indispensable in translating raw data into usable images and data sets in KPFM. These algorithms take the processed signals and reconstruct them into maps that represent the surface potential of the sample. A few notable techniques include:
- Back Projection: This approach takes data from different angles and projects it back to create a comprehensive image of the surface.
- Iterative Reconstruction: This method refines the image step by step, improving quality with each iteration.
- Model-Based Reconstruction: Utilizing models of expected surface characteristics can help refine the image more effectively by aligning the collected data with theoretical predictions.
The result of applying these algorithms is critical for analytical accuracy. The fidelity of the reconstructed images determines the conclusions drawn about the material being studied.
In KPFM, accurate data acquisition and advanced analysis techniques are essential to achieve reliable results, making them a cornerstone of effective research in nanotechnology and surface science.
Applications of Kelvin Probe Force Microscopy
Kelvin Probe Force Microscopy (KPFM) serves various important roles in several scientific fields. Its capability to provide high-resolution mapping of the surface potential at the nanoscale allows researchers to uncover details that were previously challenging to observe. This technique is pivotal for understanding material properties, enhancing technological applications, and contributing to scientific knowledge. In the following sections, we will explore specific applications of KPFM, emphasizing material science investigations, advancements in nanotechnology, and surface chemistry studies.
Material Science Investigations
In material science, KPFM is essential for examining the electronic properties of materials. The technique provides valuable insights into conductive and semiconductive materials, enabling the mapping of work function variations across a sample's surface. This can help identify defects and inhomogeneities which affect performance. Such investigations are crucial for advancing materials used in electronic devices, batteries, and solar cells. For example, KPFM data can be used to optimize the performance of organic semiconductors by revealing charge carrier distribution, which influences efficiency and stability.
Further, KPFM can assist in understanding how surface treatments or modifications affect materials. By analyzing changes in work function before and after specific treatments, researchers can infer the effectiveness of various methods in enhancing surface properties. This insight is particularly useful in coating technologies, where improvements in adhesion and corrosion resistance are desirable.
Nanotechnology Advancements
KPFM plays a significant role in the field of nanotechnology, where it is utilized to explore the behavior of nanoscale materials. For instance, researchers can assess the electrical properties of nanoparticles and nanostructures. By doing so, they can gather data on charge distribution and identify how external factors influence these properties.
One notable application is in the development of nanoscale transistors. KPFM enables scientists to examine the performance of these devices by providing insights into charge mobility and potential barriers on a nanostructured surface. As technology advances, the ability to manipulate materials at the nanoscale becomes increasingly important. KPFM serves as a tool that researchers utilize to achieve better control and understanding of these nanoscale systems.
Moreover, KPFM also supports the fabrication of innovative materials such as 2D materials like graphene. By studying their unique electrical characteristics, scientists can devise methods to integrate these materials into next-generation electronic and photonic applications.
Surface Chemistry Studies
KPFM is an important asset in surface chemistry, contributing to a deeper understanding of how molecular interactions take place at surfaces. With the ability to measure local surface potentials, KPFM can reveal how chemical modifications influence electron distribution at surfaces. This aspect is particularly beneficial for studying catalysts, where surface interactions are vital for understanding catalytic activity.
Additionally, KPFM aids in investigating surface adsorption phenomena. Researchers can observe how different molecules interact with surfaces, which can inform the design of more effective catalysts and sensors. By capturing potential differences induced by various adsorbates, KPFM highlights the complexities of surface reactivity and molecular orientation.
KPFM not only offers insights into static surface properties but also enables dynamic studies. This provision allows for a more holistic view of surface chemistry over time, crucial in applications such as surface modification and film formation.
Overall, the applications of Kelvin Probe Force Microscopy span critical domains in science and technology. From material science to nanotechnology and surface chemistry, KPFM is a versatile tool that enhances our understanding of fundamental principles and facilitates the development of advanced materials and systems.
Recent Advancements in KPFM
The field of Kelvin Probe Force Microscopy (KPFM) has seen significant developments in recent years. This section emphasizes the importance of exploring such advancements and their implications for various scientific domains. With continuous improvements in KPFM technology, researchers can achieve a higher level of precision and versatility than ever before. It is essential to consider not only the technical enhancements but also their potential to solve complex problems across disciplines like material science, nanotechnology, and surface chemistry.
New Instrumentation Techniques
Recent years have introduced innovative instrumentation techniques in KPFM. These techniques contribute profoundly to improving measurement accuracy and efficiency. One notable advancement is the integration of advanced sensors that enhance signal detection. This allows for increased sensitivity to surface potential variations, leading to clearer and more informative data sets.
Moreover, new calibration protocols have emerged. These protocols facilitate better alignment of the probe, thereby optimizing data acquisition. For instance, employing automated feedback systems has proven effective. This minimizes human error during the adjustment processes, which can significantly alter outcomes.
Another innovative approach involves the combination of KPFM with other microscopy methods. Techniques such as Scanning Tunneling Microscopy (STM) are increasingly paired with KPFM. This hybridization grants researchers insights into both electronic and topographical properties of materials in tandem.
Enhanced Resolution Methods
Enhanced resolution methods represent another area where KPFM has progressed. One effective strategy is the application of advanced signal processing algorithms. By utilizing sophisticated image reconstruction techniques, researchers can obtain higher resolution images of surface features. This increase in resolution allows for a more detailed analysis of surface phenomena.
Furthermore, developments in the probe technology have led to smaller probe sizes. These smaller probes can access finer structures on surfaces. Consequently, the spatial resolution of measurements improves considerably.
Additionally, efforts are underway to improve environmental controls during measurements. Controlling temperature and humidity helps to minimize noise and artifacts in recorded data. This is crucial for accurate analyses in sensitive experiments.
“Recent technological advancements in KPFM not only enhance resolution but also broaden its applicability in various scientific fields.”
Overall, the recent advancements in KPFM solidify its role as a vital tool in modern research. The combination of new instrumentation techniques and enhanced resolution methods fosters a better understanding of materials at the nanoscale level. These innovations open doors for breakthrough discoveries, broadening the horizons of what can be achieved using KPFM.
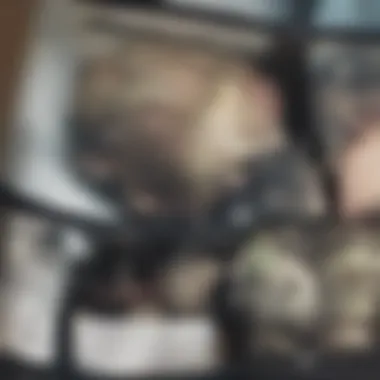
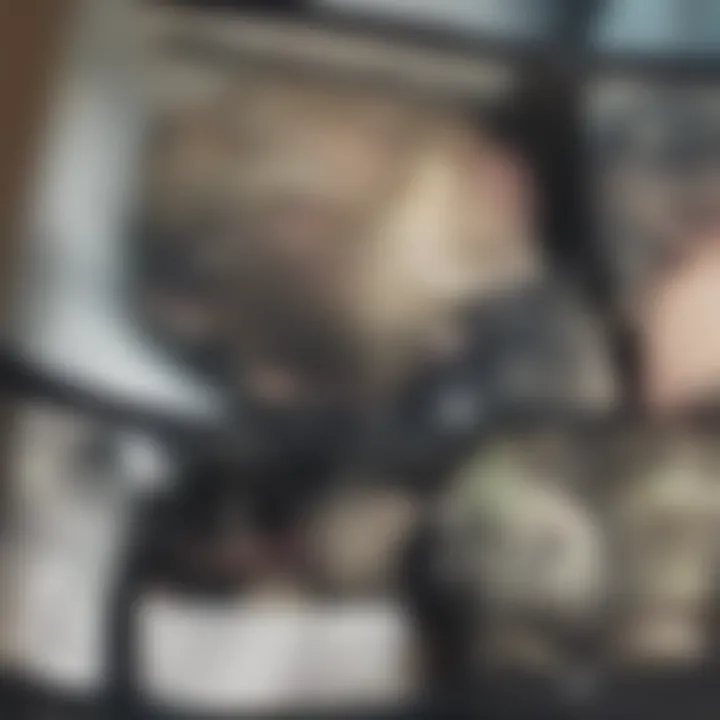
Limitations and Challenges
Understanding the limitations and challenges of Kelvin Probe Force Microscopy (KPFM) is critical for its effective application in scientific research. While KPFM offers valuable insights at the nanoscale, it is not without its constraints that researchers must navigate. Recognizing these limitations enhances the reliability of results and pushes the boundaries of this powerful imaging technique. This section addresses two main challenges: resolution constraints and material compatibility issues.
Resolution Constraints
KPFM provides capabilities to measure the electrical potential of surfaces with impressive precision. However, achieving high resolution can be a significant challenge in practice. The contrast in KPFM images is influenced by several factors such as tip size, mechanical noise, and environmental vibrations.
• Tip Size: The size of the probe tip can greatly affect the resolution. A finer tip will provide better spatial resolution but may also introduce other complications like sensitivity to surface roughness.
• Mechanical Noise: KPFM operates in an environments where mechanical vibrations can distort measurements. External sources of noise can lead to inaccurate readings affecting the quality of the data.
• Environmental Factors: Fluctuations in temperature or humidity may also interfere with the precision of KPFM measurements. Thus, environmental control is vital for maintaining consistent measurements.
To mitigate these resolution issues, researchers often adopt advanced calibration techniques alongside meticulous environmental controls. Investing in high-quality instrumentation can also assist in reducing the negative impacts of these constraints.
Material Compatibility Issues
Another challenge faced when utilizing KPFM is material compatibility. Different materials behave uniquely under the influence of the electric fields used in KPFM, and this can complicate measurements.
• Surface Charge Dynamics: Materials can possess different charging properties, which can distort the measured surface potential. If a surface charges during measurement, it may lead to artifacts in the resulting data.
• Substrate Interactions: The interaction between the probe tip and the substrate may vary significantly. Some materials may undergo physical changes, like oxidation or chemical reaction, when in contact with the probe, affecting the validity of results.
• Electrical Conductivity: Semiconductor materials exhibit diverse electrical properties that can complicate interpretations in KPFM. Researchers must take into consideration how various materials might respond to the electric fields generated by the probe.
"Understanding the underlying behaviors of materials is essential when applying KPFM for accurate evaluations in research."
Addressing material compatibility requires foresight. Researchers should carefully select the materials for study and perform preliminary investigations to understand their characteristics. Coupling KPFM with complementary techniques can also provide a more comprehensive view of the sample.
In summary, the limitations and challenges of KPFM pose significant hurdles that researchers must account for. By acknowledging factors such as resolution constraints and material compatibility issues, scientists can better prepare themselves to achieve reliable and meaningful data.
Future Perspectives of KPFM
The future of Kelvin Probe Force Microscopy (KPFM) is marked by transformative potential. As the demand for precise material characterization grows in various fields, the evolution of KPFM technologies becomes paramount. This future foresight encompasses a range of elements, benefits, and considerations that can lead to substantial advancements in surface science. Understanding these perspectives helps researchers and professionals appreciate the ongoing relevance of KPFM in scientific inquiry.
Potential Research Directions
Research in KPFM is poised to expand across several intriguing avenues in the coming years. Prioritizing these directions can enhance the scope and application of this microscopy technique:
- Substrate Sensitivity: Investigating how varying substrates influence KPFM outcomes can lead to a deeper grasp of charge distribution across different materials. Understanding this could refine calibration methods.
- Time-resolved Measurements: As techniques evolve, developing methods for capturing time-dependent phenomena using KPFM could provide insights into dynamic processes at the nanoscale. This is essential for exploring transient physical reactions.
- Functionalization: Exploring the application of KPFM on functionalized surfaces can uncover new interactions between materials. This may enhance the development of tailored materials for specific applications.
- High-Throughput Methods: The creation of automated systems to conduct KPFM measurements will facilitate large-scale studies. This can significantly speed up the process of material screening and evaluation.
Each of these areas presents opportunities to deepen knowledge while also addressing practical challenges in material characterization.
Integration with Other Techniques
The integration of KPFM with other microscopy and analytical techniques offers an exciting frontier for expanding its capabilities. Combining KPFM with other methodologies may enhance data retrieval, enabling comprehensive studies of material surfaces:
- Atomic Force Microscopy (AFM): Integrating KPFM with AFM can yield topographical information alongside potential measurements, providing a holistic view of surface characteristics. This merged data will be invaluable in material research.
- Scanning Electron Microscopy (SEM): Utilizing KPFM with SEM could offer fruitful insights into the electronic properties of materials at higher resolutions. This can aid in understanding surface modifications at the nanoscale.
- X-ray Photoelectron Spectroscopy (XPS): Combining KPFM with XPS can enhance surface analysis. This synergy allows for correlation between electronic states and chemical composition, leading to rich data sets essential for material scientists.
- Spectroscopic Techniques: When coupled with spectroscopic methods, KPFM can facilitate detailed studies of chemical reactions at surfaces, enhancing our grasp of surface chemistry.
Integrating these techniques not only enriches KPFM's functionalities but also broadens its applicability in interdisciplinary research.
The exploration of future perspectives in KPFM will significantly shape the landscape of surface science and material investigation, making it indispensable for ongoing and future research endeavors.
Understanding these directions not only informs current practices but also inspires the development of innovative approaches to surface characterization. As KPFM continues to evolve, its role will undoubtedly become more central to solving complex material challenges in science and engineering.
Culmination
Kelvin Probe Force Microscopy (KPFM) stands as a significant tool in the scientific community, particularly in the areas of material science, nanotechnology, and surface chemistry. The conclusion of this article encapsulates the essential insights gained throughout the discussion and emphasizes the relevance of KPFM in contemporary research.
One of the primary considerations regarding KPFM is its ability to probe surface properties at unprecedented resolutions. This characteristic is particularly beneficial for studying electrostatic properties, work functions, and charge distributions. These factors are vital for understanding material behaviors in various applications, ranging from semiconductors to biological systems. The article extensively discusses how practitioners can utilize KPFM to extract essential data that informs both academic inquiry and commercial product development.
Summary of Key Insights
The various segments of this article underscore several critical insights about KPFM.
- Historical Development: The evolution of KPFM technology highlights how past innovations in microscopy paved the way for current methodologies.
- Core Principles: The fundamental operational principles, such as frequency shift detection and contact modes, provide a framework for understanding how KPFM differentiates itself from other microscopy techniques.
- Recent advancements: Innovations in instrumentation and resolution have notably expanded the frontiers of research possible with KPFM.
- Limitations: Understanding the limitations, such as material compatibility and resolution constraints, is crucial for researchers who wish to use KPFM effectively.
This multifaceted overview indicates that KPFM is not merely a technique but rather an indispensable tool in advancing the frontiers of surface science research.
The Future of Surface Science Research
Looking forward, the prospects of KPFM are indeed promising. Advancements in complementary techniques, such as scanning tunneling microscopy, are expected to bolster the capabilities of KPFM.
The integration of machine learning algorithms for data analysis is one potential direction. This could lead to more sophisticated interpretations of the complex datasets generated by KPFM, providing deeper insights into material properties. Additionally, the push towards nanoelectronics may find KPFM playing a crucial role in developing future nano-devices with tailored electrical properties.
The continuing evolution of KPFM technology points towards a future where surface phenomena can be dissected with unprecedented clarity, potentially revolutionizing fields like optoelectronics, bioengineering, and energy storage. As research progresses, KPFM is poised to remain a central figure in the landscape of surface science.