An In-Depth Exploration of Power Generation Turbines
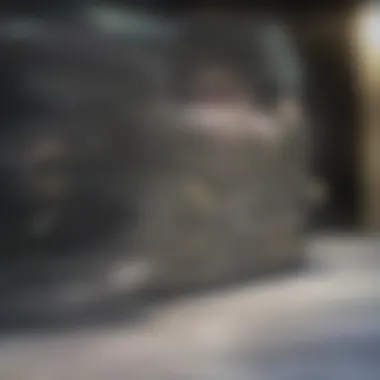
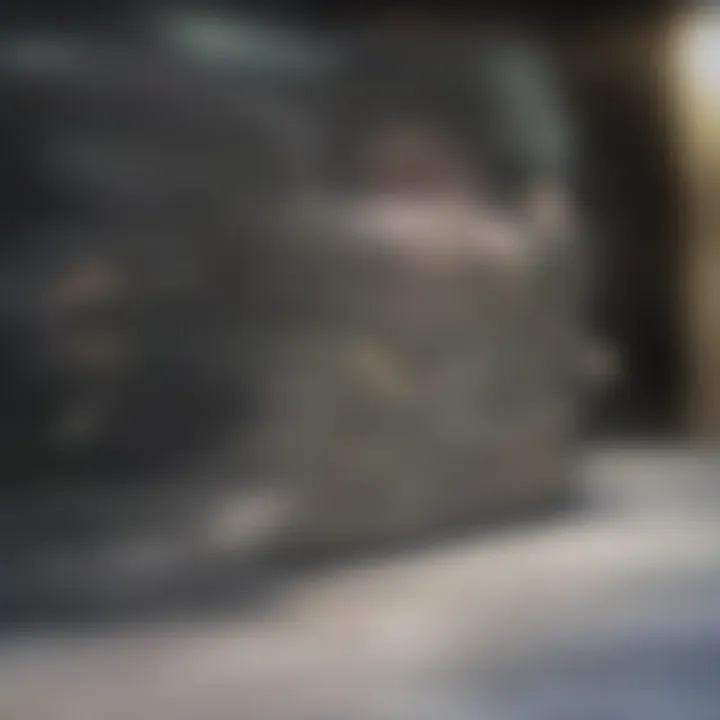
Intro
Power generation turbines play an essential role in the global energy landscape. They are the engines that drive various methods of energy production, underpinning both traditional and renewable systems. With the increasing demand for energy and the ongoing transition to sustainable sources, understanding the intricacies of turbine technology becomes more critical than ever.
This exploration will provide a detailed survey of power generation turbines, touching on their history, mechanical functionalities, efficiency metrics, and evolving designs in light of environmental concerns. Throughout this examination, we will highlight the relevance of turbines not only in current energy systems but also in future innovations.
Preamble to Power Generation Turbines
Power generation turbines are central to modern energy production. Their efficiency and reliability make them indispensable across various energy sectors. This article aims to thoroughly explore the key elements of turbines, emphasizing their role and relevance in contemporary energy systems.
Defining Power Generation Turbines
Power generation turbines convert energy from various sources into mechanical energy. These machines operate on principles involving fluid dynamics and thermodynamics. At a basic level, turbines can be defined as devices that harness the energy of flowing fluids—such as gases, steam, or water—to produce rotational energy. The rotational motion generated can then drive generators, which produce electricity.
The primary categories of power generation turbines include steam, gas, hydraulic, and wind turbines. Each type operates on distinct principles and is more suited for specific applications. This diversity illustrates the adaptability and innovative design characteristic of turbine technology.
Historical Context and Development
The evolution of power generation turbines is marked by significant milestones. The inception of steam turbines in the late 19th century transformed industrial processes. Sir Charles Parsons developed the first successful steam turbine, paving the way for efficient power generation.
As time progressed, advancements in technology led to the rise of gas turbines. These engines utilized combustion gases to generate power and quickly gained popularity due to their operational flexibility and low start-up times.
Hydraulic and wind turbines also saw considerable innovation. Hydraulic turbines emerged alongside the development of hydroelectric power in the early 20th century. Wind turbines have undergone a renaissance in recent decades, driven by the demand for sustainable energy sources.
Overall, the historical development of turbines reflects a continuous pursuit of efficiency and sustainability in energy production. Understanding this context is essential to appreciate the advanced turbine systems in operation today.
Types of Power Generation Turbines
The categorization of power generation turbines is essential to understanding their functionalities and applications. Each type serves specific purposes and operates based on distinct principles. Recognizing these types allows for a better grasp of how energy is harnessed and utilized in various sectors, contributing significantly to our energy landscape. Below, we explore four major types of turbines commonly used today: steam, gas, hydraulic, and wind turbines.
Steam Turbines
Steam turbines are a cornerstone of industrial power generation, particularly in thermal power plants. They operate on the principle of converting thermal energy from steam into mechanical energy. This process begins when water is heated in a boiler, creating steam that expands through the turbine blades. As the steam passes, it causes the blades to spin, which turns a generator to produce electricity.
Steam turbines have several benefits. They are highly efficient, especially in large-scale operations. Their design allows for high operational flexibility, making them suitable for various fuel sources, including coal, natural gas, and nuclear. However, their significant drawback involves water usage and the thermal pollution associated with the discharge of cooling water.
"Steam turbines play a crucial role in balancing electricity demand and supply, particularly during peak hours."
Gas Turbines
Gas turbines, also known as combustion turbines, are rapidly growing in popularity for power generation. They utilize natural gas or liquid fuels, promoting lower carbon emissions than traditional steam turbines. In a gas turbine, air is compressed and mixed with fuel, then ignited. The high-temperature combustion gases expand rapidly and drive the turbine blades, converting energy into mechanical power.
The advantages of gas turbines include their quick startup times and relative efficiency, making them ideal for peaking power plants. Additionally, their compact design allows for flexible installation options. Despite these benefits, gas turbines are susceptible to efficiency losses at lower loads and can require extensive maintenance due to their operational conditions.
Hydraulic Turbines
Hydraulic turbines, or hydro turbines, harness the kinetic energy of flowing or falling water. They are integral to hydroelectric power plants. Water flow turns the turbine blades, which spins a generator. Two main categories exist: impulse turbines and reaction turbines. Impulse turbines rely on water jets, while reaction turbines use submerged blades operating in pressurized water.
The significance of hydraulic turbines lies in their ability to produce large amounts of stable electricity with minimal environmental impact when designed and located appropriately. They also provide excellent scalability and can support grid stability. However, the establishment of hydroelectric projects can impact local ecosystems and water supplies, raising ecological and social considerations.
Wind Turbines
Wind turbines convert the kinetic energy of wind into mechanical energy, showcasing a transformative approach to renewable energy sources. As wind flows over the blades, it causes them to rotate, turning a generator to create electricity. Wind turbines are often found in wind farms, where multiple units are grouped to enhance output.
The key advantage of wind turbines is their zero emissions during operation, making them a vital part of green energy initiatives. They are versatile in deployment, from small residential units to large offshore or onshore wind farms. Challenges include dependence on wind patterns and potential noise and aesthetic impacts on landscapes. The efficiency of a wind turbine is highly influenced by its design and the wind conditions of its location.
Understanding different types of power generation turbines enhances our comprehension of energy dynamics. Each type contributes uniquely to the energy grid, reflecting the continuous evolution of technology and environmental considerations.
Mechanisms of Operation
Understanding the mechanisms of operation in power generation turbines is crucial. It not only highlights how these turbines function but also reveals their efficiency and effectiveness in energy conversion. Each type of turbine employs slightly different principles, but the core ideas behind their operation remain consistent across various models. By exploring these mechanisms, we gain insights into performance metrics, energy output, and design considerations that influence the overall sustainability and reliability of power generation systems.
Basic Principles of Turbine Operation
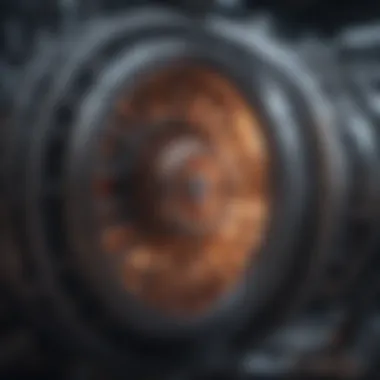
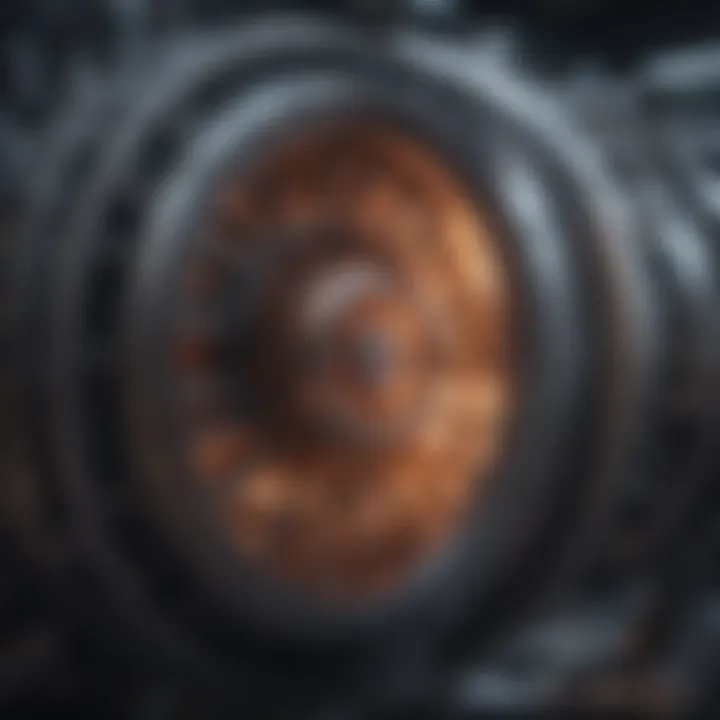
The basic principle behind turbine operation relies on fluid dynamics. For steam turbines, high-pressure steam is directed onto blades, causing them to rotate. In gas turbines, hot gases from combustion spin a rotor. Hydraulic turbines utilize flowing water to turn their blades. Wind turbines harness kinetic energy from the wind. Despite the differences in input energy sources, all turbines transform kinetic or thermal energy into mechanical energy, and subsequently into electrical energy through generators.
Several factors influence how effectively turbines convert energy:
- Type of fluid: The nature of the fluid (steam, gas, or water) directly affects efficiency. Each fluid has unique properties.
- Blade design: The shape and configuration of the blades significantly impact performance. More aerodynamic blades can harness more energy.
- Rotational speed: Optimal speed is vital for performance. Too slow may not generate sufficient power, while too fast can lead to inefficiencies.
Thus, the careful design of each turbine's components plays a significant role in its operation and overall efficiency.
Energy Conversion Processes
Energy conversion in power generation turbines can be viewed in layers. First, the input energy from steam, gas, water, or wind initiates the motion of the turbine blades. This kinetic energy subsequently transfers into mechanical energy as the blades turn the rotor. The mechanical energy is then converted into electrical energy through an attached generator.
The efficiency of this conversion process can be affected by:
- Heat losses: In steam and gas turbines, wasted heat can diminish efficiency. Strategies like combined cycle systems help reduce these losses.
- Friction: Mechanical friction within the turbine parts can lead to energy losses. Selecting materials that minimize friction is important.
- Maintenance: Regular upkeep ensures that parts are functioning optimally, which directly affects conversion efficiency.
Understanding these processes allows for improvements in turbine technology. Innovations can promote higher efficiency levels and reduce environmental impacts. This is particularly relevant as the demand for cleaner energy sources rises.
In summary, the mechanisms of turbine operation are fundamental to their role in energy generation. Recognizing the principles of operation along with energy conversion processes aids in advancing technology that meets modern energy needs.
Efficiency Factors in Turbine Performance
The performance of power generation turbines is significantly influenced by various efficiency factors. Understanding these factors is crucial for optimizing energy production and ensuring economic operation. Overall efficiency encompasses thermodynamic and mechanical performance, alongside the impacts of design. Key to this discussion is the importance of enhancing turbine efficiency for reducing operational costs and maximizing output.
A highly efficient turbine not only saves fuel and minimizes emissions but also improves the overall integrity of energy systems. In many energy plants, noticeable improvements in turbine efficiency can lead to appreciable cost savings and better resource management.
Thermodynamic Efficiency
Thermodynamic efficiency refers to the efficiency gained in the conversion of thermal energy into mechanical energy. This type of efficiency is governed by the thermodynamic cycle in which a turbine operates.
The Rankine cycle is common in steam turbines, where water is heated to produce steam, which then drives the turbine. The Carnot efficiency sets the theoretical limit of thermodynamic efficiency, determined by temperatures of heat sources and sinks. The closer a turbine operates to this ideal, the better its thermodynamic efficiency.
It is essential to monitor temperature and pressure conditions to optimize the working fluid without overstressing the materials involved. When designing or upgrading turbines, improvements in thermal efficiency frequently lead to better overall performance even for small energy generation adjustments.
Mechanical Efficiency
Mechanical efficiency pertains to the effectiveness with which the mechanical components of the turbine convert input energy into useful mechanical energy. It takes into account losses due to friction, abrasion, or aesthetic issues within the turbine
For instance, bearings, seals, and other moving parts can contribute to energy losses if they are not designed correctly. Hence, techniques like advanced materials and coatings can minimize friction and ensure long-lasting operation. The mechanical design should foster reduced energy loss while enhancing reliability.
A focus on good lubrication practices helps prolong service life and boosts mechanical efficiency. The correct balance of materials and components can transform the operational capacity of a turbine.
Impact of Design on Efficiency
The design of a turbine is paramount to its efficiency factors. Key design elements include blade shape, size, and material, as these influence both aerodynamic performance and structural integrity.
For instance, blades must be carefully engineered for optimal airflow, which in turn enhances conversion efficiency. Computational Fluid Dynamics (CFD) is often used in designing these components. Additionally, turbine configuration affects how energy is harnessed from the primary energy source.
By integrating advanced design principles and modern technology, turbine manufacturers can realize substantial improvements in efficiency. Furthermore, prototyping and testing new designs under varied conditions can yield critical data for refining future iterations.
Ultimately, the combined effect of thermodynamic and mechanical efficiencies, alongside thoughtful design choices, can empower turbines to perform at their peak, reducing costs and environmental impacts.
Applications of Power Generation Turbines
The applications of power generation turbines are diverse and significant. They serve as the backbone of energy production across multiple sectors, from industrial settings to renewable energy systems. Understanding the specific applications of these turbines is crucial for assessing their impact on the overall energy landscape.
Industrial Applications
In industrial settings, power generation turbines are paramount for converting various forms of energy into mechanical power. Industries like manufacturing and processing rely on steam and gas turbines to drive machinery and facilitate production processes. The ability to generate large amounts of energy efficiently is essential. Industrial turbines increase productivity and reduce operating costs.
In practice, steam turbines are often used in boilers to convert heat energy into electricity. They can operate on various fuels, including coal and natural gas.
- Key Benefits:
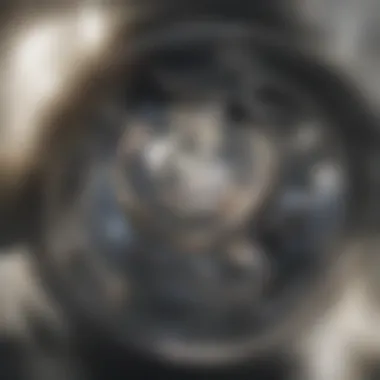
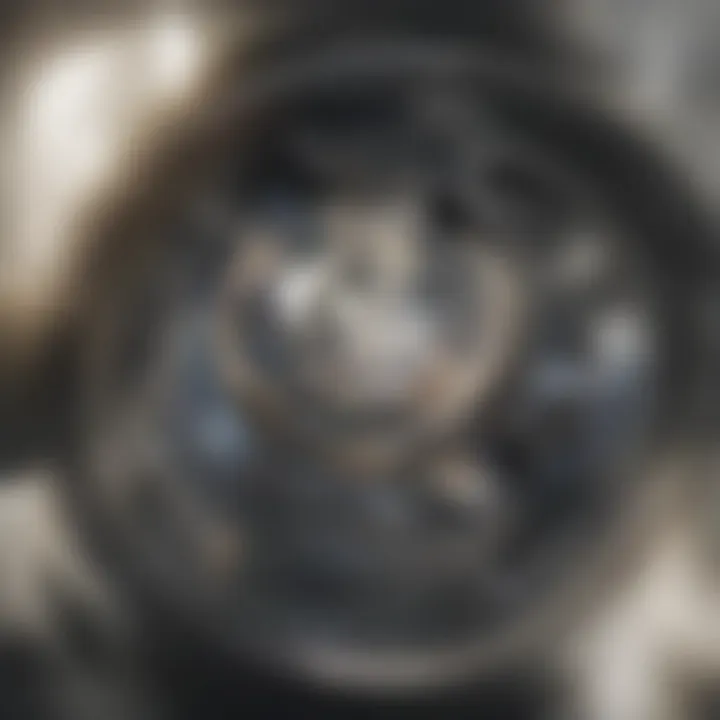
- High efficiency under large loads.
- Reliability and durability.
- Capability to adjust output based on demand.
Additionally, gas turbines are popular for their quick start-up times and relatively lower emissions compared to traditional coal systems. They are favored in scenarios where flexibility is required, responding to fluctuating energy demands.
Power Plants
Turbines play a critical role in power plants, forming the core of electricity generation. From nuclear to fossil fuel power plants, different turbine types facilitate energy conversion. For example, in fossil fuel power plants, steam is generated from burning coal or gas, then directed to steam turbines. This process is efficient and capable of producing substantial electricity.
In contrast, in nuclear power plants, steam produced during the nuclear fission process is used to rotate turbines. Utility companies invest in turbine technology to optimize output and efficiency.
- Considerations for Power Plants:
- Load management: Ensures a stable power supply.
- Efficiency upgrades: New turbine designs can improve energy output.
- Environmental regulations: Compliance with emissions standards.
Power plants also rely on gas turbines for peaking power generation. These turbines can handle the rapid changes in load demanded by the grid, making them vital for energy stability.
Renewable Energy Integration
The integration of turbines with renewable energy sources represents a key advancement in sustainable energy production. Wind turbines convert kinetic energy from wind into mechanical power, which is then transformed into electricity. This shift has been critical in reducing reliance on fossil fuels.
Solar thermal plants also utilize turbines, where sunlight is used to heat a fluid, producing steam that drives turbines.
- Benefits of Renewable Integration:
- Decrease in greenhouse gas emissions.
- Diversification of energy sources.
- Enhance energy security through local production.
Innovations in turbine technology are driving improvements in efficiency and adaptability, making it feasible to harness more energy from renewable sources, even in varying environmental conditions. As renewable energy becomes more integrated into the energy mix, the role of turbines in capturing and converting energy will also expand.
"The future of energy relies significantly on the effective applications of power generation turbines within diverse energy systems."
In summary, the applications of power generation turbines are extensive. Their roles in industrial settings, power plants, and renewable energy systems highlight their importance in today's energy landscape. As technology advances, the possibilities for turbine applications will continue to grow.
Impact of Renewable Energy Technologies
The growing emphasis on renewable energy technologies has significantly reshaped the landscape of power generation, particularly in the context of turbines. As we confront the repercussions of climate change and the depletion of fossil fuels, the transition to sustainable energy sources has become imperative. This section discusses the impact of renewable energy technologies on turbine design and operation, emphasizing the necessity and benefits of such adaptations.
Adapting Turbines for Sustainable Use
In the quest for sustainable power generation, existing turbine designs need to evolve. Adaptation involves modifying traditional turbine mechanisms to function efficiently with renewable sources, such as wind and solar energy, which provide energy in a more variable and unpredictable manner compared to conventional sources.
- Wind Turbines: These turbines have been at the forefront of renewable technology. Their designs incorporate materials that can withstand higher loads due to wind variability. Techniques like predictive maintenance algorithms enhance performance and reliability.
- Hydrogen Turbines: Emerging technologies such as hydrogen production promise to drive a new wave of turbine adaptations. These turbines must manage different combustion properties without compromising efficiency.
- Other Renewable Sources: Biomass and ocean energy present unique challenges. Turbines need to be adept at converting energy from these sources, paralleling advancements in hydraulic turbines.
The significance of adapting turbines lies in their ability to harness energy from diverse, sustainable inputs while reducing reliance on fossil fuels.
Innovations in Turbine Design
Innovation is essential for enhancing turbine efficiency in the context of renewable energy. The emergence of digital tools and advanced materials significantly influences turbine design.
- Smart Turbines: Integration of IoT (Internet of Things) technologies allows for real-time data collection. Smart turbines provide insights into operational efficiency, facilitate predictive maintenance, and minimize downtime.
- Advanced Materials Science: The use of lighter, stronger materials like carbon fiber composites can lead to more efficient turbine blades. These materials can withstand higher operational demands while optimizing performance in severe weather conditions.
- Aerodynamic Enhancements: Research into turbine blade shapes has led to the development of blades that maximize energy extraction from wind. Innovations which allow for dynamic adjustment of blade angles optimize energy capture as wind conditions change.
The innovations not only signify a response to the needs of renewable energy but also an ongoing pursuit of greater efficiency and performance in power generation through turbine technology.
"Modern turbine technologies not only adapt to renewable energy but also redefine the parameters of efficiency and performance across generations."
Environmental Impacts of Turbines
The discussion around power generation turbines encompasses significant environmental aspects. Understanding the impacts of these technologies is crucial for developing sustainable energy practices. This section examines two primary concerns: noise pollution and the ecological footprint of turbine systems. Both elements are essential to consider when evaluating the overall efficiency and acceptability of turbine technologies in modern society.
Noise Pollution Considerations
Noise pollution is a pressing concern for many communities situated near turbine installations. Gas and steam turbines, while efficient in energy production, generate considerable sound during operation. The noise levels can interfere with local wildlife and affect human health, particularly in residential areas.
- The sound pressure levels of gas turbines typically range between 85 and 110 decibels, depending on the design and technology used.
- Long-term exposure to such noise can lead to stress, sleep disturbance, and increased risk of cardiovascular diseases for nearby residents.
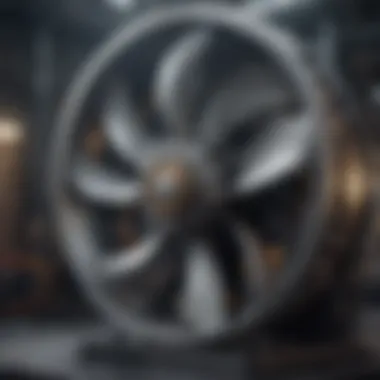
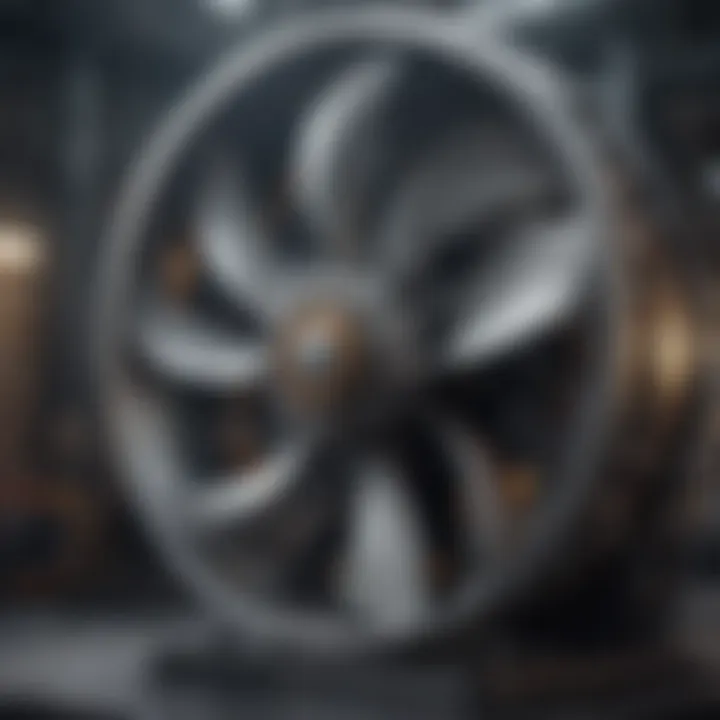
Mitigation measures exist to lessen the impact of this noise. Key strategies include:
- Sound barriers constructed from sound-absorbing materials
- Optimized turbine design to reduce operational noise
- Strategic placement of turbines in less populated areas
Advanced technologies are being explored to further reduce turbine noise. Research in this area is aiming to develop ultra-quiet turbines that utilize innovative engineering solutions.
"Addressing noise pollution not only enhances community acceptance but also balances the relationship between energy production and environmental health."
Ecological Footprint
The ecological footprint of power generation turbines is critical in the discussion of sustainability. The construction, operation, and eventual decommissioning of turbines impose demands on land, water, and resources. This impact varies significantly between different types of turbines.
- Steam turbines require substantial water for cooling processes, leading to concerns about water use in arid regions.
- Wind turbines, although considered renewable, occupy large swathes of land, potentially disrupting local wildlife habitats.
- Hydraulic turbines can alter aquatic environments, affecting fish populations and water quality.
To minimize environmental impacts, companies are adopting practices such as:
- Lifecycle assessments to evaluate the total ecological impact from manufacturing to decommissioning
- Habitat restoration efforts post-installation
- Innovative design concepts aiming for reduced land and resource use, such as floating wind turbines in offshore locations
Future Trends in Turbine Technology
The power generation sector is undergoing rapid transformations, and the future trends in turbine technology play a critical role in this evolution. As the world moves toward a more sustainable energy landscape, it is imperative to understand how advancements in turbine design, digitalization, and material sciences can lead to efficient energy conversion. The relevance of these developments cannot be overstated, as they not only enhance performance but also align with global efforts to mitigate climate change and promote renewable energy.
Digitalization and Smart Turbines
Digitalization is revolutionizing various industries, and turbine technology is no exception. The integration of Internet of Things (IoT) devices and sensors allows for real-time monitoring of turbine operations. This connectivity enables operators to track performance metrics closely and predict maintenance needs before breakdowns occur. Consequently, smart turbines lead to reduced operational costs and enhanced longevity of the equipment.
Another advantage of digitalization is data analytics. Data collected from smart turbines can be analyzed to improve efficiency. This enables power generation plants to optimize energy output based on changing demand or environmental conditions. Predictive algorithms can significantly improve operational decisions. Being proactive rather than reactive in maintenance strategies proves helpful in maintaining high efficiency levels.
Advancements in Materials Science
Materials science is pivotal in developing more resilient and efficient turbines. Innovative materials can withstand extreme operational conditions and reduce wear and tear. For instance, advances in composite materials and coatings account for weight reduction without compromising strength. This leads to improved efficiency in turbine operation, as lighter components require less energy to function effectively.
Additionally, research into nanomaterials presents new opportunities for turbine applications. By utilizing nanostructures, it is possible to enhance thermal and mechanical properties significantly. Improved materials can withstand higher temperatures, facilitating better performance in turbines, especially in gas and steam applications. These advancements highlight the importance of ongoing research in materials science as a cornerstone for the next generation of turbines.
Global Energy Transition and Turbines
As nations grapple with the pressing challenge of climate change, the global energy transition is in full swing. Turbines are at the heart of this transition, given their role in converting various types of energy into usable forms. The shift toward renewable energy sources such as wind, solar, and hydropower underscores the need for adaptable turbine technologies.
This transition necessitates the rethinking of turbine designs that can efficiently harness renewable resources. For example, wind turbine designs are evolving to capture lower wind speeds, making energy generation more viable in various geographical locations. Similarly, developments in hydro turbines aim to allow for better fish passage, thus minimizing ecological impacts while maximizing energy capture.
Moreover, regulatory policies and international agreements call for cleaner energy production. This pushes manufacturers and engineers to innovate continually. Aligning turbine technologies with sustainability goals ensures that they remain integral to the energy landscape. The future clearly indicates that advances in turbine technologies will play a vital part in achieving global energy targets.
"Innovation in turbine design is essential for meeting future energy needs sustainably."
In summary, the trends in turbine technology are not merely about improving efficiency; they reflect a comprehensive approach to sustainable power generation. Digitalization, advancements in materials science, and the critical global energy transition collectively shape the landscape of power generation. As these elements develop, the role of turbines will become increasingly significant in achieving a cleaner and more efficient energy future.
Finale
The conclusion of this article serves as a critical reflection on the importance of power generation turbines. These machines are not merely components in the energy production cycle; they are integral to understanding energy efficiency, technological innovation, and sustainability in the modern world. Examining their role allows us to appreciate the intricate balance of design, efficiency, and environmental impact that defines contemporary power generation systems.
Reflections on the Role of Turbines
Power generation turbines have made substantial contributions to both industrial and domestic energy needs. Their evolution, from basic mechanical systems to advanced technology relying on complex digital controls, highlights humanity's pursuit of efficiency and sustainability. It is crucial to recognize that turbines are not only vital for power generation but also serve as a benchmark in energy innovation. They symbolize the progress made in engineering and energy management practices.
The evolution of turbine technology reinforces several key points:
- Efficiency: As new materials and designs emerge, turbines continue to improve in performance, affecting overall energy consumption.
- Adaptability: Turbines are being adapted to integrate with renewable energy sources, transforming their application in the current energy landscape.
- Impact Assessment: Understanding the ecological effects of turbine operation becomes fundamental in sustainable practices, considering the balance between energy production and environmental stewardship.
In essence, turbines act as a hallmark for ongoing research and development efforts aimed at improving energy infrastructure while reducing environmental footprints.
The Path Forward
Looking ahead, the path for power generation turbines is defined by technological advancements and increased focus on sustainability. The global shift towards cleaner energy sources mandates a rethinking of turbine design and functionality. Future innovations aim to blend high efficiency with minimal ecological impacts.
Key factors shaping the future include:
- Smart Turbines: The integration of IoT (Internet of Things) in turbine systems promises enhanced monitoring, predictive maintenance, and performance tracking.
- Materials Development: Innovations in materials science will lead to lighter, more durable turbines that can withstand harsher operating conditions.
- Policy and Investment: Increasing support for renewable energy technologies may drive investments in turbine development, shaping the market towards more sustainable solutions.
Ultimately, the focus on renewable integration and smart technologies will dictate how turbines evolve. As society continues to navigate the complexities of energy demands and environmental concerns, turbines will remain at the forefront of discussions about energy generation.