Exploring the Multifaceted Role of Plasma in Science

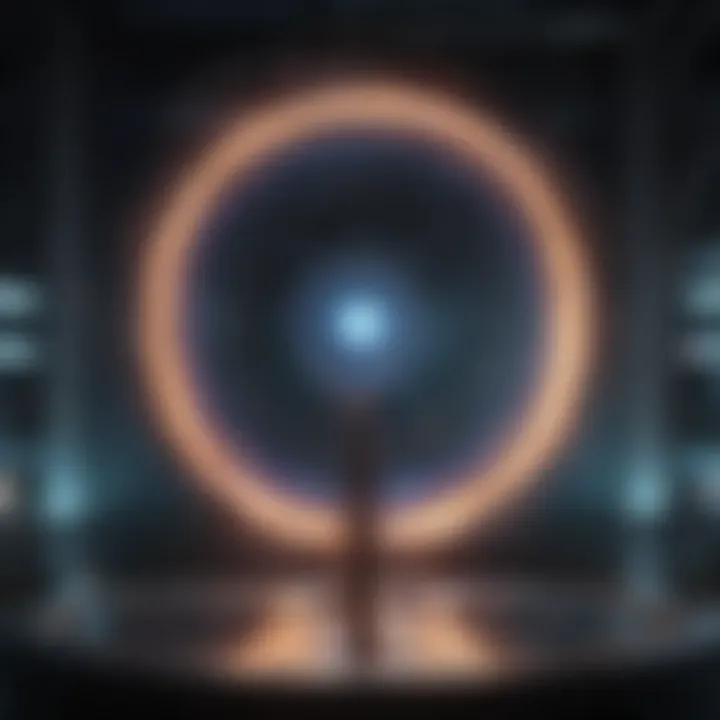
Intro
Plasma, often labeled as the fourth state of matter, goes beyond a mere scientific curiosity; it serves profound purposes across varied fields from astrophysics to healthcare. While many are familiar with solids, liquids, and gases, plasma presents a compelling narrative in how matter behaves under extreme conditions. Understanding plasma isn't just an academic pursuit; it impacts technologies that shape daily life, from the screens of our devices to the very stars twinkling above.
In this exploration, readers may find themselves drawn into a world where scientific principles intermingle with practical applications. The reason plasma holds such significance lies in its unique properties and behaviors, which become especially apparent when it interacts with electromagnetic fields.
Through detailed analysis, this article will unpack the essence of plasma, shedding light on its multifarious roles. The objectives here are straightforward: to clarify plasma's functional relevance in nature and to delve into its applications that highlight human innovation.
Research Overview
Summary of Key Findings
Plasma’s versatility facilitates numerous applications in science and technology:
- Astrophysics: Plasma is the primary constituent of stars, including the sun. Its behavior explains various astronomical phenomena.
- Healthcare: The use of plasma technology in sterilization and medical treatments, such as plasma-induced cancer therapies, has shown promising results.
- Industrial Applications: Plasma contributes to semiconductor manufacturing, enhancing the quality and efficiency of electronic components.
By dissecting these crucial aspects, we will build a refined understanding of the underlying principles governing plasma's myriad roles.
Background and Context
Historically, the concept of plasma was not always clear. It surfaced in the early 20th century when scientists observed highly energized gases exhibiting unique behaviors. Fast forward to today, plasma technology permeates various sectors, uncovering its worth in both academic research and practical solutions.
To grasp how plasma fits into contemporary science, it's essential to trace its roots through prevailing theories and applications. The historical context underpins the relevance and interest surrounding plasma, ensuring a deeper engagement with the material.
Methodology
Experimental Design
The exploration of plasma involves a careful design of experiments that measure its properties under controlled conditions. This includes:
- Observing plasma behavior in different environments.
- Adjusting variables like temperature and electromagnetic fields to study their influence.
Data Collection Techniques
Accurate data is crucial for understanding plasma's interactions. Typical techniques include:
- Spectroscopy: Analyzing light emitted from plasma enables insights into the energy states of particles.
- Digital Imaging: High-resolution cameras can capture plasma formations, providing visual evidence for theoretical models.
Through these methods, researchers compile a wealth of data that elucidates plasma's behavior and potential.
"Plasma is not just a state of matter; it represents the bridge between various scientific domains, linking cosmic events to healthcare advancements."
In the sections to follow, we will continue to build on this narrative, looking closely at the intricate functions of plasma and what they mean for various walks of life, all while maintaining a lens focused on scientific rigor and real-world implications.
Understanding Plasma
Grasping the concept of plasma is crucial for anyone delving into the intricate web of physics, engineering, medicine, and even the cosmos. Plasma, distinguished as the fourth state of matter, captures the essence of ionized gases, showcasing unique characteristics that set it apart from solids, liquids, and gases. It’s not just about the term itself; it's also about the profound implications of plasma in various scientific domains and practical applications.
This section will address several key points:
- What plasma fundamentally is: We’ll look at the essential definition and its distinct attributes that define it.
- Why it matters: From its role in energy production to its influence in space phenomena, we'll cover the significance.
- How it relates to the broader picture: An understanding of plasma opens up discussion regarding its comparison with other states of matter, enriching our comprehension of physics.
Definition and Characteristics of Plasma
Plasma is often described as an ionized gas, where free electrons and ions coexist. This unique composition leads to several defining characteristics:
- High-energy particles: Unlike neutral gases, plasma consists of charged particles that respond to electromagnetic fields. This property enables practical applications such as plasma televisions and nuclear fusion reactors.
- Luminous state: When excited, these particles emit light, which is why we observe glowing arcs in neon signs.
- Collective behaviors: Plasma behaves collectively rather than as individual particles, leading to various phenomena like waves and instabilities.
The combination of these traits not only invites curiosity but also prompts in-depth study across multiple fields.
Comparison with Other States of Matter
When comparing plasma with other states of matter—solid, liquid, gas, and Bose-Einstein condensate—it’s evident that each state presents unique properties, yet plasma stands out.
Solid
Solid materials hold their shape, characterized by tight molecular bonds. This structural integrity is highly beneficial in engineering and architecture. For instance, metals like steel are robust for building tall skyscrapers against strong winds. However, when considering energy transfer, solids possess limited capabilities compared to plasma.
Liquid
Liquids, on the other hand, like water or mercury, offer fluidity and adaptability. They mold to their containers, making them essential in various industrial applications such as hydraulics and cooling systems. Their capacity to conduct heat is noteworthy, yet they lack the vast energy transfer potential found in plasma.
Gas
Gases are vibrant states, filled with potential energy, moving freely to occupy any space offered. Air, for instance, is essential for life; however, gases' energy is dispersed, making them less effective in certain applications like energy storage compared to plasma. Gas can readily transition into plasma under specific conditions, linking the two states.
Bose-Einstein Condensate
Bose-Einstein condensate represents a rare state occurring at temperatures near absolute zero, where atoms behave as a single quantum entity. While it reveals fascinating quantum phenomena, its practical applications are limited compared to plasma. Plasma’s capacity for high energy and adaptability makes it a powerful tool in technology and research, illustrating the stark contrast in functionality across these states.
As we dissect these states, we gain not only a clear distinction between plasma and its gaseous neighbors but also a greater appreciation for plasma's intricate role in technology and the natural world. This comparison lays the groundwork for further exploration into plasma's formation, behaviors, and applications.
"Understanding plasma is crucial, as it affects everything from medical innovation to astrophysical phenomena."
With this knowledge in our toolkit, we can now move on to the nuances of plasma formation, giving us a better grasp of how to harness its potential.
Formation of Plasma
In discussing plasma, its formation provides a fundamental understanding of this fourth state of matter. Plasma is not a substance found merely as is but instead emerges from specific conditions that allow matter to transition from solid, liquid, or gas states into an ionized state. This transformation is pivotal as it lays the groundwork for the roles plasma plays in a myriad of applications, spanning from astrophysics to medical technology. Recognizing the intricacies of how plasma is formed helps us understand not only its properties but also the potential benefits derived from its use in various fields.
Ionization Processes
Thermal Ionization
Thermal ionization occurs when atoms absorb enough energy, usually through heat, to lose electrons and form ions. The intriguing aspect here is how temperature plays a crucial role in facilitating this process. High thermal energy leads to increased movement of particles, granting them the ability to overcome electrostatic forces holding electrons in the atom. Thus, thermal ionization stands out as a highly efficient method for producing plasma, especially in environments where heat is abundant, such as stellar interiors. The primary appeal lies in its relative simplicity when conditions are favorably aligned, though it’s not without its drawbacks; high temperatures might lead to unwanted materials being ionized as well.
Photonionization
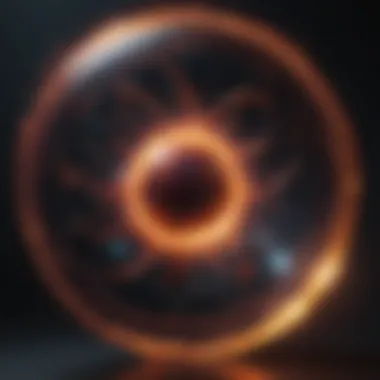
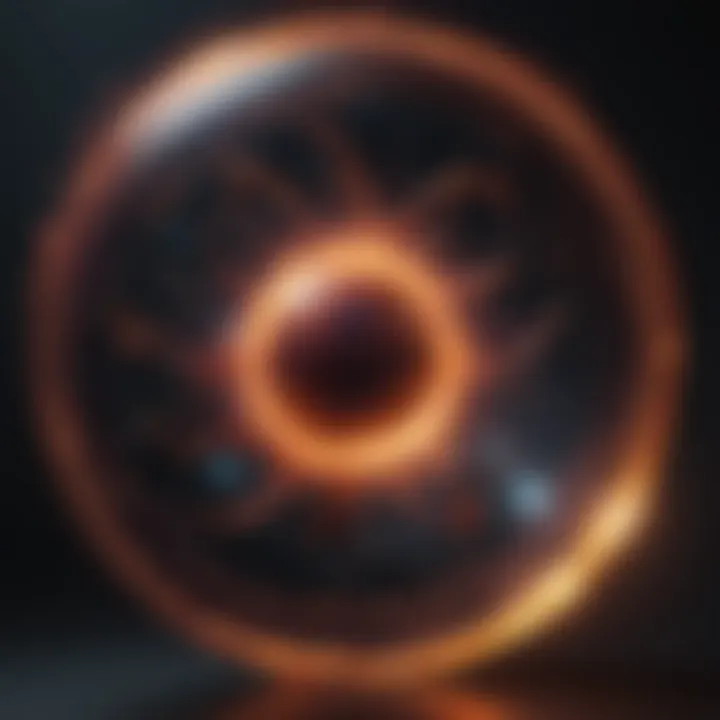
Photonionization entails the absorption of photons—light particles—which bestows enough energy onto atoms to displace their electrons. One of the key characteristics of photonionization is its versatility, as it can occur at various energy levels of photons, making it adaptable to different environments. This method is often favored in laboratory settings, where precise control over light inputs allows for tailored plasma generation. A notable advantage is the capacity for selective ionization, which is invaluable in research and technological contexts. However, it might require complex equipment to generate appropriate resonance conditions, potentially scaling up costs and complicating processes.
Electroionization
Electroionization relies on electric fields to strip electrons from atoms, effectively turning them into ions. The standout feature of this mechanism is its rapid response, making it a preferred technique in various applications like plasma displays and industrial processes. Here, low energy input can yield swift ionization, which amplifies productivity in practical scenarios. The benefits are clear, especially in applications needing precise control over ionization rates; however, the challenges include ensuring uniformity in plasma formation, as localized spikes in electric fields might lead to inconsistent results.
Conditions for Plasma Creation
High Temperatures
High temperatures are a primary condition for plasma creation, as they supply the necessary energy to ionize gases. The tremendous heat can strip electrons from atoms, thereby forming plasma. This characteristic is particularly essential in astrophysical phenomena, like the sun, where extreme temperatures facilitate nuclear fusion processes, creating vast amounts of plasma. But it’s worth noting that while high temperatures are beneficial for creating plasma, controlling these conditions is critical. Excessively high temperatures without proper management can lead to significant energy losses.
Low Pressures
Low pressures are another important condition for plasma formation. Under less atmospheric pressure, particles can move more freely, allowing for easier collisions that lead to ionization. The importance of low pressures is evident in applications like plasma processing in semiconductor fabrication, where it is vital to maintain a controlled environment. However, the challenge here lies in maintaining stability; too low of a pressure could lead to the unpredictability of the plasma state, complicating its practical use.
Electric or Magnetic Fields
Electric or magnetic fields are paramount in the creation and manipulation of plasma. These fields can steer charged particles within the plasma, allowing for the containment of this state of matter and enhancing its applicability. For instance, fusion reactors rely heavily on magnetic fields to confine plasma at high densities without allowing it to touch the reactor walls. The unique feature of these fields is their ability to manipulate plasma flow and stability, making them a beneficial choice for innovation in energy production. However, generating and maintaining these fields can be technically complex and may require significant resources, posing challenges for widespread implementation.
"Understanding the formation of plasma lays a foundation for technological advancements that can follow in various fields. The pathways through which plasma can be created and sustained—be it through ionization processes or environmental conditions—highlight the intricacies involved in harnessing this captivating state of matter."
In assessing the formation of plasma, it becomes clear that the underlying processes and conditions involved present both a challenge and an opportunity. Each method and condition for creating plasma brings its own advantages and disadvantages, underscoring the need for thoughtful consideration in both research and applications.
Physical Behavior of Plasma
Plasma is not just a soup of charged particles; it has a distinct behavior that sets it apart from other states of matter. Understanding how plasma interacts dynamically is crucial because it affects everything from stellar activity to technological applications. This physical behavior includes how waves propagate through plasma, how it reacts to magnetic fields, and why these interactions matter deeply in both natural and engineered systems.
Plasma Waves and Oscillations
Langmuir Waves
Langmuir waves are a fascinating manifestation of plasma behavior. These high-frequency oscillations occur in the electron density of the plasma, triggered by the presence of electric fields. Their primary contribution lies in their ability to transport energy and momentum within the plasma. This makes them particularly key to understanding processes such as plasma heating in devices like tokamaks.
One standout characteristic of Langmuir waves is their frequency, which is directly related to the electron density in the plasma. This relationship is crucial since it allows scientists to infer properties about the plasma based on wave behavior. In this context, they serve as an invaluable tool for diagnostics in both astrophysical and laboratory plasmas.
A unique feature of Langmuir waves is their ability to couple with other types of waves, creating a complex interplay that can lead to various phenomena, both beneficial and potentially disruptive. For example, while they can enhance performance in plasma confinement systems, excessive oscillations might introduce instabilities. Thus, understanding Langmuir waves helps to optimize plasma performance while avoiding pitfalls.
Alfven Waves
On the other hand, Alfven waves are another important type of plasma oscillation characterized by their interaction with magnetic fields. These low-frequency waves propagate along the magnetic field lines and are instrumental in transferring energy across different regions of plasma. Their contribution is vital to understanding space weather phenomena and the dynamics of solar winds, making them particularly relevant for researchers in astrophysics and fusion energy.
One prominent feature of Alfven waves is their relationship with magnetic field strength and plasma density. This characteristic allows for intriguing applications in understanding how energy is transferred from the sun to the Earth, which has implications for satellite operations and telecommunications.
The unique advantage of studying Alfven waves is their ability to inform us about the stability of plasma configurations. However, they also bear some disadvantages, as they can lead to turbulence when they interact with other waves in certain conditions. Therefore, grasping Alfven wave dynamics provides critical insights into both natural and engineered plasma systems.
Interaction with Magnetic Fields
Magnetohydrodynamics
Magnetohydrodynamics (MHD) represents the study of how magnetic fields interact with electrically conducting fluids, including plasma. This area of research is significant as it underpins the understanding of various astrophysical phenomena like solar flares and the dynamics within stars. By unraveling the complexities of MHD, we can learn how magnetic fields can stabilize or destabilize plasma flows.
One notable characteristic of MHD is its foundational role in fusion research. MHD principles are key to designing containment systems, like magnetic confinement fusion devices. Therefore, any advances in MHD can yield substantial returns in the quest for sustainable fusion energy.
A unique feature of MHD applications is its capacity for predictive modeling. It allows scientists to predict behaviors under different conditions, which can be indispensable in anticipating environmental effects on plasma systems during energy production or in natural settings.
However, MHD can present challenges, particularly in accurately modeling turbulence and instabilities, which could drastically influence plasma confinement or energy output. Thus, while MHD shines a light on many plasma interactions, it also reveals complexities that necessitate further exploration.
Cyclotron Motion
Cyclotron motion describes the trajectory of charged particles as they spiral around magnetic field lines due to Lorentz force. This motion is particularly significant because it plays a crucial role in how plasma behaves in magnetic confinement systems and astrophysical environments. Understanding this aspect of plasma physics is key to insights into energy transfer mechanisms and particle confinement.
A defining characteristic of cyclotron motion is its dependence on both particle charge and the strength of the magnetic field. This relationship is useful in numerous applications, especially in designing particle accelerators and diagnosing behaviors in fusion reactors.
The unique feature of cyclotron motion lies in its energy aspects—particles accelerate under this motion, which can be harnessed for specific applications in energy generation or medical technologies, like proton therapy for cancer treatment.
Despite these advantages, cyclotron motion can lead to adverse phenomena, such as resonances that may destabilize plasma dynamics. Thus, while it provides immense benefits to the field, it also introduces challenges that need to be effectively managed. Understanding these interactions is crucial for exploiting plasma's vast potential in technology and research.
"By examining the physical behavior of plasma, we unlock the door to various technological advancements and cosmic phenomena, enhancing our grasp of both earthly and celestial events."
Plasma in Astrophysics
Plasma plays an indispensable role in the realm of astrophysics, impacting our understanding of celestial phenomena and the mechanics of the universe. Recognized as the predominant form of visible matter in the cosmos, plasma is essential for studying the life cycles of stars, the composition of interstellar mediums, and the structure of galaxies. In the following sections, we will delve deeper into the role of plasma in stars and the broader universe, examining its intricate behaviors and contributions.
Role of Plasma in Stars
Nuclear Fusion
Nuclear fusion is at the heart of stellar energy production, underpinning the life of stars from their birth until their demise. This is a process where hydrogen nuclei combine under immense temperatures and pressures to form helium, releasing significant amounts of energy. The key characteristic of nuclear fusion is its ability to generate vast amounts of energy, which powers stars, making them visible across great distances in the universe.
One of the appealing aspects of nuclear fusion is its fuel source—hydrogen, abundant in the cosmos. This aspect positions fusion as a clean and sustainable energy option not only in stellar processes but also holds potential for controlled energy production on Earth. The unique feature of fusion lies in its efficiency; for every gram of hydrogen converted into helium, a substantial release of energy occurs, far exceeding that of chemical reactions.
However, despite its advantages, nuclear fusion poses numerous challenges, primarily in terms of achieving and maintaining the extreme conditions needed for reaction. Additionally, the technology for harnessing fusion on Earth is still in experimental stages, making it a subject of ongoing research.
Stellar Formation and Evolution
Stellar formation and evolution are intrinsically linked to plasma dynamics. Stars form from clouds of gas and dust, primarily composed of hydrogen, that collapse under gravity. The densification of these clouds leads to the formation of protostars, where plasma becomes crucial as temperatures rise and nuclear fusion ignites.
The key characteristic of stellar formation is the transformative journey from gas to a full-fledged star. This evolution is a vital concept throughout astrophysics as it dictates the lifecycle of stars and their eventual impact on the universe. Whether it’s the creation of elements within a star or their dispersal after a supernova, plasma dynamics are at play.
The unique feature of stellar evolution is its iterative cycles, as younger stars create heavier elements through fusion, eventually enriching the surrounding interstellar medium when they die. One disadvantage here is that the life cycle of a star can take millions or even billions of years, making direct observation of these processes challenging for astronomers and astrophysicists.
Cosmic Plasma and the Universe
As we broaden our perspective beyond individual stars, the concept of cosmic plasma emerges, highlighting its presence in vast structures like galaxies and the interstellar medium.
Interstellar Medium
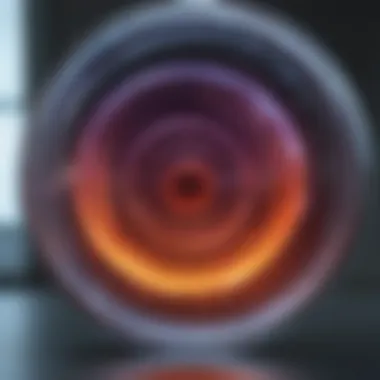
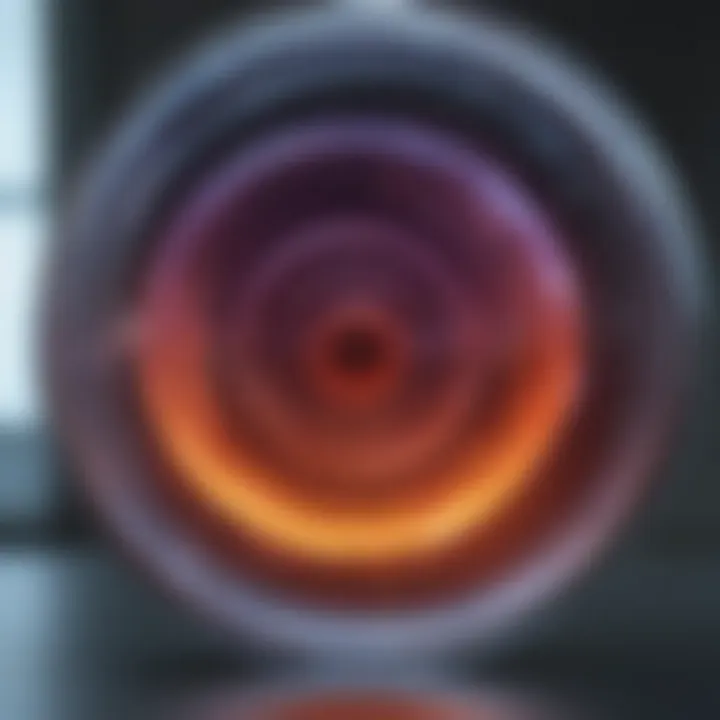
The interstellar medium, the matter that exists in the space between stars, is predominantly composed of plasma. This plasma serves as the fuel from which new stars arise and affects the dynamics of galaxies. The key characteristic here is that the interstellar medium acts as a medium of exchange; it carries stellar materials and influences the gravitational forces at play.
A significant aspect of the interstellar medium's makeup is its magnetic fields, which can impact star formation by exerting pressure that can either inhibit or promote the collapse of clouds to form new stars. This quality makes it a fascinating subject of study, as understanding its dynamics could reveal insights into the formation of stellar and galactic structures.
However, the study of the interstellar medium is not without its challenges. The diffuse nature of the medium makes it difficult to observe directly, requiring indirect methods to piece together the behaviors and compositions of these regions.
Galactic Magnetic Fields
Galactic magnetic fields represent another exciting frontier for the study of cosmic plasma. These magnetic fields arise from the movement of charged particles within plasma, unveiling a compelling relationship between plasma physics and celestial dynamics. The key characteristic here is that these magnetic fields are not static; instead, they change and evolve, influencing how matter and energy interact on a galactic scale.
This dynamic nature of galactic magnetic fields can shape cosmic structures, regulating star formation rates and contributing to the overall behavior of galaxies. The unique feature of these fields is their capacity to channel energies across vast distances, essentially guiding the pathways of cosmic rays and other forms of energy.
Despite their intriguing roles, deciphering the complexity of galactic magnetic fields adds layers of difficulty to astrophysical studies, necessitating advanced observational techniques and theoretical modeling to fully understand their influence.
"Understanding plasma in astrophysics deepens our comprehension of not just the stars, but the universe itself."
Plasma Applications in Technology
Plasma technology serves as a cornerstone in many modern applications, underscoring its versatility across multiple fields. From electronics to industrial processing, plasma harnesses unique properties that enable groundbreaking developments. This section explores its significant contributions, emphasizing why plasma technology stands out in today’s ever-evolving tech landscape.
Plasma in Electronics
Plasma Displays
Plasma displays are a prominent application of plasma technology, primarily utilized in television screens and digital signage. One notable aspect of plasma displays is their ability to produce rich colors with deep blacks. This characteristic results from using tiny cells filled with noble gases, which, when excited by electrical energy, emit light. The vibrant output can make films and gaming experiences extraordinarily immersive.
A unique feature of plasma displays is their wide viewing angle. Unlike LCDs, which can exhibit color distortion at sharp angles, plasma technology maintains its color integrity from nearly any perspective. While these screens can offer superior image quality, they also pose some disadvantages, like higher energy consumption compared to LED technologies. However, many enthusiasts appreciate the performance of plasma displays, viewing them as a worthy investment for high-quality visual experiences.
Semiconductor Fabrication
Plasma technology also plays a crucial role in semiconductor fabrication, vital for modern electronic devices. One main aspect of this application is the use of plasma etching, which precisely removes layers from semiconductor material. This process is essential in defining circuit patterns on chips, making it indispensable for the electronics industry.
A key characteristic of plasma fabrication is its ability to achieve nanoscale precision. As devices become smaller and more complex, this precision is paramount. However, there are some considerable challenges that one must reckon with, such as potential damage to sensitive materials during processing. Still, the efficiency and precision offered by plasma technologies, particularly in creating high-performance and small-scale components, underscore their pervasive use in the tech arena.
Industrial Uses of Plasma
Plasma Cutting
Plasma cutting is an innovative application that has revolutionized metalworking industries. This technique employs a focused stream of ionized gas, or plasma, to cut through electrically conductive materials, such as steel and aluminum. One of the standout features of plasma cutting is its speed; it can slice through thick materials much faster than traditional cutting methods.
This technique's advantage is minimal heat-affected zones, leading to cleaner and more precise cuts. This reduces the likelihood of warping or cracking in the materials, making it a popular choice in manufacturing and construction. However, plasma cutting also comes with challenges such as the need for proper ventilation due to the generation of fumes and heat, which can be a health concern.
Surface Modification
Surface modification is another fascinating application of plasma technology in industry. This process involves treating materials with plasma to improve their surface properties, such as adhesion, wettability, and resistance to wear. An important aspect of surface modification using plasma is adjusting surface chemistry without altering the bulk material.
A key characteristic of this method is its ability to improve bonding capabilities in coatings or adhesives. Through plasma treatment, surfaces become more receptive, enabling better adherence of paints or adhesives. Nonetheless, it is vital to consider that not every material responds well to plasma treatment; some may require specific conditions for effective modification. Still, the benefits often outweigh the drawbacks, as enhanced surface characteristics can lead to longer-lifespan products and improved performance.
Plasma technology continues to push the envelope in both electronics and industrial applications. Its adaptability makes it a technology to watch for future advancements and innovations.
Plasma Medicine: Innovations in Healthcare
Plasma medicine represents a fascinating confluence of science and practical application, ushering in novel methods of diagnosis and treatment. This field explores how plasma, often engineered under controlled conditions, can serve as a powerful tool in healthcare. As the understanding of plasma’s capabilities grows, so does its potential influence in promoting health and treating various conditions.
Plasma's Role in Sterilization
One of the hallmark applications of plasma in medicine is its ability to sterilize surfaces and instruments. Plasma's ionized particles exhibit antimicrobial properties, making it highly effective against a broad range of pathogens, including bacteria, viruses, and fungi. The process involves exposing medical devices and surgical instruments to cold plasma, which disrupts the cellular structure of microorganisms, effectively rendering them harmless.
The benefits of this method, compared to traditional sterilization techniques like autoclaving or chemical methods, include:
- Efficiency: Plasma sterilization typically requires shorter exposure times.
- Environmentally Friendly: It doesn’t rely on harsh chemicals that may leave residues or pollute the environment.
- Material Compatibility: Ideal for sensitive instruments, as the low temperatures used prevent heat damage.
Plasma sterilization exemplifies how innovations in plasma technology can significantly bolster healthcare practices, addressing critical issues like infection control, particularly in surgical settings.
Therapeutic Uses of Cold Plasma
Cold plasma therapy is another compelling area where plasma shows promise. It involves generating low-temperature plasma that can interact with biological tissues without causing harm. The nuances in its therapeutic applications are noteworthy, particularly in the context of wound healing and cancer treatment.
Wound Healing
Wound healing often challenges medical professionals. Cold plasma has emerged as a beneficial approach, accelerating the healing process for chronic wounds. The key aspect of this therapy lies in its capability to enhance cellular proliferation and promote angiogenesis, which is the formation of new blood vessels.
This treatment stands out for several reasons:
- Speed: Research indicates improved healing times in patients treated with cold plasma compared to conventional treatments.
- Non-Invasive: Unlike some surgical interventions, plasma therapy is non-invasive and generally well-tolerated.
However, as with any new technique, there are considerations to keep in mind, primarily surrounding the standardization of treatment protocols and deeper understanding of long-term effects.
Cancer Treatment
Cold plasma also extends its therapeutic portfolio into oncology, offering potential as a novel cancer treatment option. Its unique characteristic of inducing apoptosis—or programmed cell death—in cancer cells sets it apart. By applying plasma directly onto tumors, researchers have discovered that it can selectively target cancerous cells while sparing surrounding healthy tissue.
The advantages of this approach include:
- Precision: Targeting only cancer cells reduces damage to healthy cells, which is a frequent drawback of traditional therapies like radiation and chemotherapy.
- Minimally Invasive: Cold plasma can be applied with minimal discomfort to the patient.
Nevertheless, this field is still in its infancy, necessitating additional research to optimize treatment regimens and evaluate efficacy comprehensively.
In summary, plasma medicine holds immense potential to transform healthcare. Its applications in sterilization and therapeutic modalities exemplify how science can innovate to improve patient outcomes and advance medical practices. As researchers continue to explore plasma's capabilities, its multifaceted role in medicine is likely to expand further, paving the way for future breakthroughs.
"The ability of plasma to interact with biological systems opens doors to new treatments that could reshape conventional medical practices."
For further insights into the applications of plasma technology, you can check resources like Wikipedia or Britannica.
Environmental Impacts of Plasma Technology
Understanding the environmental implications of plasma technology is essential, and offers a lens through which one can appreciate its multifaceted nature. Plasma, beyond its physical wonder, is making strides towards addressing some pressing global challenges, specifically waste management and energy production. As industries search for greener solutions, plasma technology emerges as a pivotal player in reshaping how we handle waste and harness energy, leaving an observable mark on both ecology and industry.
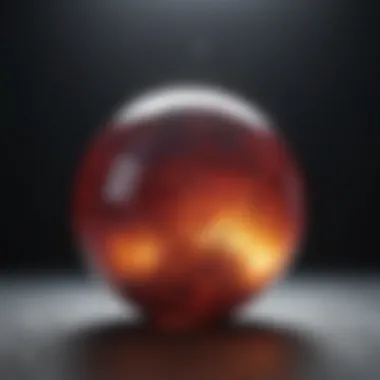
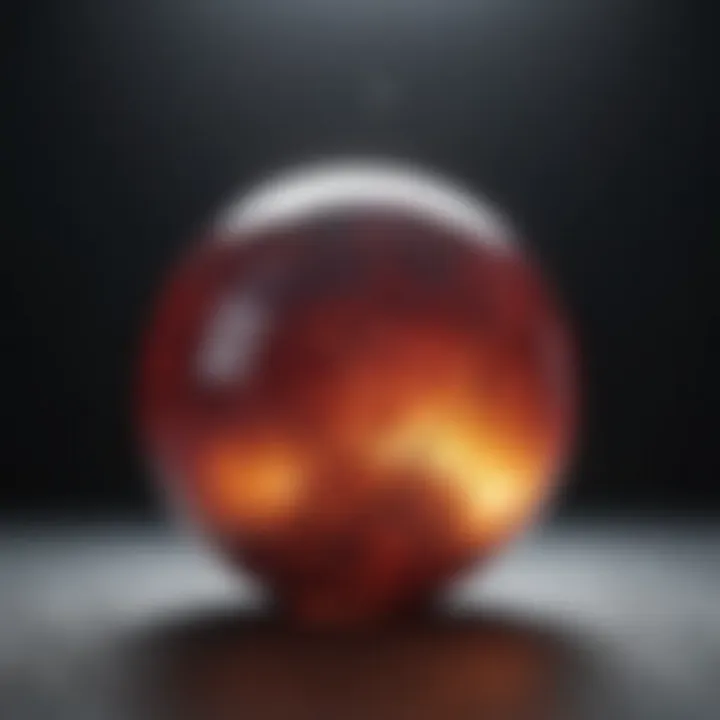
Plasma and Waste Management
Plasma Gasification
Plasma gasification represents a groundbreaking approach to waste management that turns garbage into energy. The core idea is to use high temperatures—usually above 1,000 degrees Celsius—to break down organic material. This process doesn’t merely burn waste; rather, it transforms it at a molecular level into syngas, a mix of hydrogen and carbon monoxide. What catches the eye about plasma gasification is its capability to minimize landfilling of waste.
- Key Characteristic: The efficiency of converting waste into usable gas is unmatched. Compared to traditional incineration, this method produces fewer toxins, making it a cleaner alternative.
- Unique Features: One standout benefit of plasma gasification is that it can handle diverse waste types, including plastics, which are often problematic for other disposal methods.
However, it’s worth noting some limitations. The initial investment for plasma gasification plants can be steep. Additionally, if not managed properly, the gaseous byproducts could still present some environmental hazards.
Pollution Control
The role of plasma technology in pollution control cannot be overstated. It offers innovative solutions for reducing harmful emissions from industrial processes. This technology allows for the decomposition of pollutants into harmless constituents, effectively acting like a sieve for noxious gases.
- Key Characteristic: Plasma systems are capable of treating volatile organic compounds (VOCs) and other serious pollutants efficiently, capturing what many older technologies fail to.
- Unique Features: The high energy created in plasma reactors enables real-time treatment, which means pollutants can be neutralized as they are emitted. This leads to cleaner air quality in surrounding environments.
On the flip side, setting up these systems also incurs significant operational costs, which could deter smaller companies from adopting them. Nevertheless, the long-term environmental benefits present a strong case for their consideration—a compelling argument for their investment.
Energy Production using Plasma
Nuclear Fusion Research
Nuclear fusion research often rides the wave of excitement and speculation, showing promise in providing a sustainable energy solution that can potentially shift our reliance from fossil fuels. This process involves fusing hydrogen atoms to create helium, a reaction that produces a lot of energy, similar to what happens in the sun. The excitement around nuclear fusion is attributed to its potential to generate vast energy from relatively small quantities of fuel without the long-term radioactive waste associated with traditional nuclear power.
- Key Characteristic: A major advantage is the abundance of hydrogen isotopes, which can be harvested from water, providing a virtually limitless fuel supply.
- Unique Feature: The ability to achieve high energy outputs with minimal environmental footprint underscores why fusion is seen as the holy grail of energy production.
However, the science of sustaining a controlled fusion reaction has proven to be complex. High costs and technical challenges present hurdles for wider implementation. Still, every step forward in research brings us closer to realizing this dream.
Sustainable Energy Solutions
In the quest for sustainable energy, plasma technology offers innovative engineering solutions. Various platforms are exploring the process of plasma conversion of natural materials into fuels such as biofuels or even hydrogen fuel, which may lead to cleaner alternatives.
- Key Characteristic: Plasma technology's efficiency in converting waste feedstock into valuable energy carriers makes it appealing, especially in efforts to reduce landfill waste.
- Unique Features: Plasma systems often support a circular economy approach, transforming what would be waste into a resource.
Nonetheless, transitioning to these technologies requires careful planning and integration into existing infrastructures. The upfront costs and potential operational complexities may challenge some stakeholders. Yet, as research progresses and efficiencies improve, the appeal of utilizing plasma for sustainable energy solutions continues to grow.
"Plasma technology not only provides solutions but also ignites a transformative wave of innovation in how we manage waste and produce energy, setting the stage for a cleaner future."
Plasma Physics: Theoretical Insights
Understanding plasma physics is like peeling back the layers of a complex onion; with each layer, new insights into the behavior and dynamics of this unique state of matter are revealed. Theoretically, plasma physics serves as the backbone that supports numerous applications and phenomena, from cosmic events to advanced technologies. This section entwines concepts and models that help us grasp the fundamental aspects of plasma, providing a framework to explore its vast implications.
Mathematical Models of Plasma Behavior
Mathematical modeling acts as the compass for navigating the intricate world of plasma behavior. These models are essential tools for physicists, allowing them to simulate and predict how plasma interacts under various conditions. For instance, one might employ the Vlasov equation or the Maxwell equations to describe plasma dynamics and electromagnetic fields.
Models like these help illuminate the relationships between density, pressure, and temperature in plasma systems. Additionally, they can predict phenomena such as wave propagation, particle collisions, and instabilities. Without these mathematical frameworks, our understanding of plasma phenomena would remain obscure and untested. The benefit of having robust mathematical models is that they can extract significant insights from complex plasma behaviors, guiding experiments and practical applications alike.
Challenges in Plasma Physics Research
Research in plasma physics does not come without its hurdles. One major area of difficulty lies in instability issues, where the plasma behaves unpredictably under certain conditions. This unpredictability can lead to disruptions in containment systems or unexpected outcomes in experiments.
Instability Issues
Instability within plasma systems poses a recurring theme in research. In a nutshell, it refers to the tendency of plasma to deviate from a stable state, often caused by external factors like magnetic field fluctuations or temperature variations. This aspect is crucial because it directly affects the understanding of nuclear fusion processes and astronomical phenomena. Such issues can result in energy loss or even damage to experimental devices.
The unique feature of instability is how significantly it can impact the outcome of plasma experiments. Understanding these instabilities is advantageous since they can lead to discoveries about plasma confinement and stabilization methods. By proactively addressing these challenges, researchers can refine their approaches to plasma manipulation, ultimately enhancing the reliability of further applications.
Containment Difficulties
Another notable aspect is containment difficulties that researchers encounter when trying to hold plasma within a confined space. Containing plasma typically involves using magnetic fields, which can sometimes falter, leading to loss of containment and subsequent plasma escape.
The key characteristic of containment difficulties lies in the challenge of maintaining equilibrium between plasma pressure and magnetic confinement. This makes it a crucial consideration in the fields of fusion research and space physics. One of its unique features is that containment systems must adapt to varying conditions, making their design and implementation an ongoing area of research.
While there are high stakes involved, addressing these containment issues also presents opportunities for breakthroughs in technology. For instance, innovative approaches to magnetic confinement can lead to effective solutions for sustainable energy production.
"The study of plasma physics not only illuminates the universe's secrets but also acts as a crucible for technological innovation."
In summary, the theoretical insights gained from plasma physics not only enhance our understanding of this fascinating state of matter but also pave the way for practical advancements in multiple disciplines.
Future Perspectives on Plasma Research
Plasma research is blooming like spring flowers after a long winter. As the fourth state of matter, its applications and implications stretch from the far reaches of the cosmos to the cellular structures of our bodies. Understanding future perspectives of plasma research holds great significance as it can unlock doors to innovative solutions in various domains, spanning healthcare, energy, and environmental sustainability. This exploration not only highlights how plasma interacts with different terrains of science but also casts light on potential avenues that can be pursued in the years to come.
Advancements in Plasma Technology
Emerging Applications
The field of emerging applications for plasma technology is buzzing. One noteworthy aspect is its use in plasma-enhanced chemical vapor deposition (PECVD), a technique that is shaping the future of the semiconductor and photovoltaic industries. The key characteristic of this method is its ability to produce thin films at lower temperatures compared to traditional methods, making it a timesaving and energy-efficient choice. A unique feature here is the ability of plasma to promote chemical reactions that would otherwise require high temperatures, presenting a substantial advantage in terms of materials integrity.
However, it’s not all smooth sailing. While the technology holds immense promise, scaling up these processes for large-scale industrial use can pose challenges. More research might be needed to establish consistent results across different settings. Overall, the blend of energy efficiency and functional versatility makes emerging applications of plasma a vital talking point in contemporary discussions.
Innovative Research Areas
When discussing innovative research areas, one cannot ignore the potential of plasma in fusion energy. The concept of harnessing plasma for sustainable energy production is like piecing together a puzzle that could change our energy landscape. A significant characteristic of this area is its aim to replicate the processes that power our sun, potentially offering an almost limitless supply of clean energy. The unique feature of this research is its emphasis on developing containment systems through magnetic fields to keep plasma stable and manageable.
Nevertheless, challenges remain. Achieving the needed temperatures and pressures for fusion is daunting, and progressing from experimental to practical implementation requires rigorous testing and ironing out operational inefficiencies. Yet, it is precisely this bold ambition that makes innovative research areas so appealing and essential for future energy solutions.
Interdisciplinary Approaches in Plasma Science
Collaboration Across Fields
In the realm of plasma science, collaboration across various disciplines is key. Bringing together physicists, chemists, biologists, and engineers can foster a rich environment for generating ideas and breakthroughs. This approach is especially relevant in studying the effects of plasma in medical applications, where insights from biology can lead to improved therapeutic solutions. The key characteristic of such interdisciplinary collaborations is the exchange of knowledge, enabling researchers to tackle complex problems from multiple fronts.
A notable unique feature of these collaborations is the diverse skill sets they bring together, often leading to unexpected innovations. However, one must consider potential drawbacks, such as communication gaps between experts of different fields. Nevertheless, these integrations often result in a more robust understanding of plasma's applications, making it a beneficial strategy for advancing the science.
Integration of Physics and Biology
Lastly, the integration of physics and biology in plasma research holds great promise. This synergy can be seen most vividly in the therapeutic use of plasma in medicine, which capitalizes on its antimicrobial properties. The key aspect here is the customization of plasma parameters for specific biological applications, allowing for targeted treatments. One unique feature of this integration is the ability to apply non-thermal plasma to living tissues without harming them, thus offering a new frontier in medical treatments.
However, while the promise is bright, there are some hurdles to overcome, including understanding the interactions between plasma and biological systems at a molecular level. If researchers can navigate these challenges, the integration could lead to significant advancements in healthcare, offering solutions that were once only dreamed of.
"The future of plasma science is not just about finding answers; it's about asking the right questions together."
In sum, the future of plasma research is bright and multifaceted, woven intricately with possibilities that are begging to be explored and realized.