Exploring the Formation of Black Holes: A Deep Dive
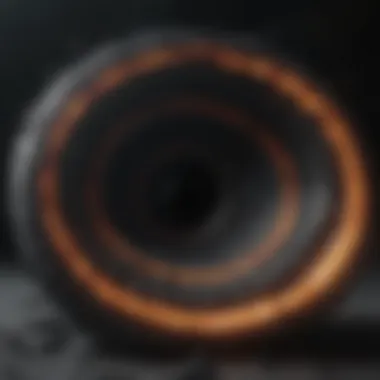
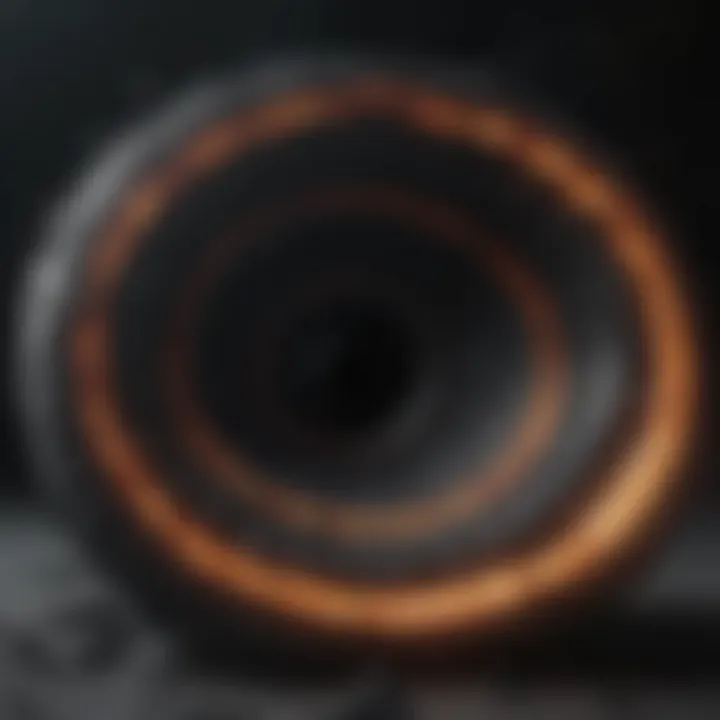
Intro
The exploration of black holes stands at the frontier of modern astrophysics. These enigmatic entities challenge our understanding of gravity and spacetime. To grasp their formation involves examining key processes in stellar evolution, gravitational interactions, and cosmic phenomena. This article delves into the mechanisms that lead to the birth of black holes, providing a thorough insight aimed at scholars and enthusiasts alike.
Research Overview
Summary of Key Findings
Recent advancements in astrophysics reveal intricate processes behind black hole formation. Several key findings emerge in this area:
- Black hole types: They can be classified as stellar black holes, supermassive black holes, and intermediate black holes, each with unique formation pathways.
- Stellar collapse: Massive stars undergo gravitational collapse at the end of their lifecycle, leading to the creation of stellar black holes.
- Supermassive black holes: Found at galactic centers, their origins are still a topic of deep research. They likely formed from the merging of smaller black holes or rapid accretion of gas and stars.
- Cosmological implications: Understanding black holes helps elucidate galaxy formation and the nature of dark matter.
Background and Context
Black holes were first theorized in the 18th century. The term itself was coined in the 1960s. Early studies were primarily theoretical, based on Einstein's general relativity. Over time, observational techniques improved, enabling researchers to detect the presence of black holes through their effects on surrounding matter. This ongoing research has vast implications on how we comprehend cosmic evolution and the overall structure of the universe.
Methodology
Experimental Design
Studying black holes involves diverse methodologies. Observational astronomy plays a crucial role in detecting their presence. Techniques include:
- Gravitational wave detection: Instruments like LIGO have confirmed black hole mergers.
- X-ray observations: Satellites such as Chandra provide insights into accretion disks around black holes.
Data Collection Techniques
Data is collected by monitoring high-energy signals in the universe. The key strategies involve:
- Telescopic surveys: Ground and space-based telescopes identify potential black hole candidates.
- Mathematical modeling: Simulations allow scientists to predict black hole behaviors and relate them to observable phenomena.
As this article progresses, we will uncover more about black holes’ formation processes, their classification, and their significance in our understanding of the cosmos. Each section reveals layers of complexity and invites further exploration into one of science's most profound mysteries.
Understanding Black Holes
The topic of understanding black holes holds critical significance in the study of astrophysics. This is primarily due to their mysterious nature and the profound implications they have on our comprehension of the universe. Black holes serve as key players in various cosmic phenomena, from galaxy formation to the dynamics of spacetime. Analyzing black holes involves considering not only their physical characteristics but also the fundamental laws of physics that govern them.
By exploring black holes, researchers gain insight into fundamental questions about gravity, matter, and energy. This understanding is increasingly relevant as we strive to unify general relativity and quantum mechanics.
Furthermore, black holes challenge our perceptions of reality, pushing the boundaries of known science. They act as natural laboratories that test theories and models, providing rich material for academic inquiry. Each discovery related to black holes, whether observational or theoretical, can significantly influence our grasp of the universe.
Definition of Black Holes
Black holes are regions in spacetime where the gravitational pull is so immense that nothing, not even light, can escape from them. This extreme gravitational force arises from a concentration of mass within a small volume, resulting in a singularity at the core where density becomes infinite and the laws of physics as we understand them cease to apply.
The boundary surrounding this region is called the event horizon. It marks the point of no return for any matter or radiation that crosses it. The concept of a black hole challenges our intuitive understanding of gravity, as they represent an anomaly in the fabric of spacetime.
Historical Context
The concept of black holes was not always accepted. Historically, the idea evolved from theoretical foundations laid by several notable scientists. In 1783, John Michell proposed the idea of "dark stars" with gravity so strong that light could not escape.
In the 20th century, Albert Einstein's general theory of relativity provided the mathematical framework for understanding black holes. The term "black hole" was coined by physicist John Archibald Wheeler in 1967.
As technological advancements occurred, observational evidence started to accumulate. This included indirect evidence from the behavior of stars near invisible mass and gravitational waves detected from merging black holes. Today, with sophisticated instruments and telescopes, researchers continue to investigate these enigmatic entities, unveiling their mysteries and enriching our perspective on the cosmos.
Types of Black Holes
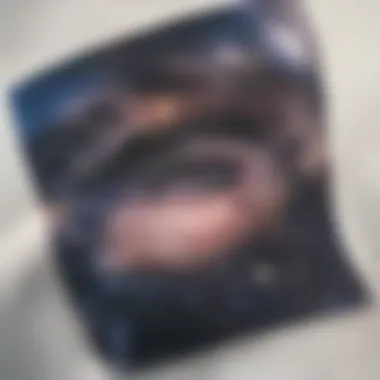
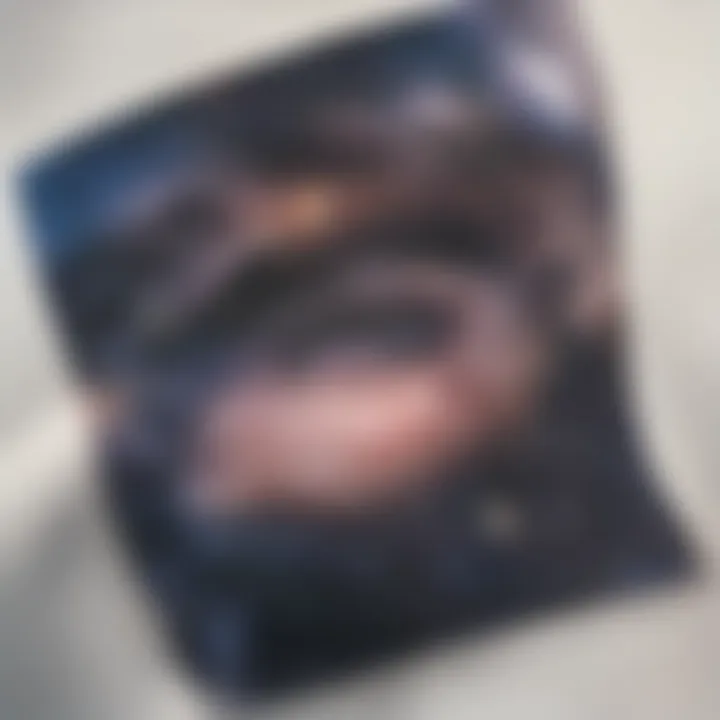
The study of black holes encompasses various classifications. Understanding these types is crucial for grasping the complexities of astrophysics and the broader universe. Each type of black hole has unique characteristics and formation processes that provide insights into cosmic evolution. This section will detail these classifications, their significance, and how they contribute to our knowledge of black holes and their roles in the universe.
Stellar Black Holes
Stellar black holes are formed from the remnants of massive stars after they undergo supernova explosions. When a star exhausts its nuclear fuel, gravity takes over, leading to the collapse of its core. As the star’s material falls inward, it creates a black hole if the remaining mass exceeds a particular threshold, known as the Tolman-Oppenheimer-Volkoff limit.
- Mass Range: Typically, stellar black holes have masses ranging from about three to several tens of solar masses.
- Formation Process: The life cycle of a star is a key aspect of stellar black hole formation. A massive star evolves through various stages, culminating in its death. The core collapse leads to the formation of a singularity, surrounded by the event horizon.
- Importance: Studying stellar black holes helps scientists understand stellar evolution, the lifecycle of stars, and the mechanics of supernovae.
Supermassive Black Holes
Supermassive black holes are found at the centers of galaxies and have masses ranging from millions to billions of solar masses. Their origins remain a subject of ongoing research, but several theories exist regarding their formation.
- Formation Theories: These black holes may form through the merging of smaller black holes, the accretion of gas and stars over time, or through the direct collapse of massive gas clouds early in the universe’s history.
- Galaxy Dynamics: Supermassive black holes play a significant role in galaxy formation and evolution. Their gravitational influence can regulate star formation and the overall dynamics of the galaxy.
- Research Significance: Investigating supermassive black holes contributes to our understanding of the nature of the universe, dark matter, and cosmic evolution.
Intermediate Black Holes
Intermediate black holes are less understood and fall between stellar and supermassive black holes, with masses ranging from hundreds to thousands of solar masses. Their existence has been supported by some observational evidence, yet many questions remain.
- Formation Mechanisms: Theories range from the merger of stellar black holes to direct collapse of massive stars. However, the exact mechanism for their formation is still unclear.
- Observational Challenges: Detecting intermediate black holes is challenging due to their mass range, which often places them in a middle ground where they are not easily spotted. Ongoing research aims to identify observational methods to confirm their presence in the universe.
- Astrophysical Implications: They serve as a bridge in our understanding of black hole evolution and may provide clues about the formation of supermassive black holes.
Primordial Black Holes
Primordial black holes are hypothesized to have formed in the very early universe, potentially from density fluctuations in the hot, dense conditions shortly after the Big Bang. Their existence is theoretical, and they differ significantly from the black holes formed from stellar processes.
- Mass Range: They could have a wide range of masses, from very small (as light as a proton) to much larger ones.
- Cosmological Importance: If primordial black holes exist, they could play a role in dark matter, providing a potential explanation for this mysterious component of the universe. They could also offer insights into the conditions present in the early universe.
- Current Research: Scientists are actively searching for evidence of primordial black holes, using various observational methods and indirect evidence through gravitational wave detections.
Understanding the classifications of black holes provides a foundation for studying their roles in the cosmos. Each type has unique characteristics that contribute to the broader narrative of cosmic evolution and the workings of the universe.
The Process of Black Hole Formation
Understanding the process of black hole formation is crucial for grasping not only the nature of these enigmatic objects but also their role in the universe. The formation of black holes encapsulates a series of astrophysical phenomena deeply embedded in stellar dynamics and evolution. This section delves into these intricate mechanisms, identifying key stages that lead to the birth of black holes. By exploring the specific elements involved, we can better appreciate the significance of black holes in the cosmos.
Stellar Evolution and Lifecycle
The lifecycle of a star is fundamental in the journey toward black hole creation. Stars undergo several phases throughout their existence, from formation to their eventual end states. Initially, they spend billions of years in a steady state, fusing hydrogen into helium. As the star exhausts its nuclear fuel, it transitions into later stages, which may include becoming a red giant or supergiant. During these phases, the core contracts while the outer layers expand. The star's mass is a crucial factor. Stars with a mass greater than about 20 times that of our Sun have the potential to become black holes after undergoing supernova explosions. This lifecycle not only highlights the transformation of material but also sets the stage for the phenomena that follow.
Supernova Explosions
Supernova events serve as pivotal moments in the birth of black holes. These colossal explosions occur when massive stars reach the end of their life cycle. During a supernova, the outer layers are expelled while the core collapses under its own gravity. This dramatic event releases enormous amounts of energy and can briefly outshine entire galaxies. The significance lies in how this explosion contributes to the formation of a black hole. If the remaining core's mass is sufficient, around three solar masses or more, it will collapse into a black hole, marking a transition from a luminous star to an invisible entity. This process invites ongoing study into stellar remnants and the mechanics that govern such powerful explosions.
Core Collapse Mechanism
The core collapse mechanism is a vital aspect of black hole formation. When a star's core can no longer support itself against gravitational forces, it begins to collapse. As the core contracts, temperatures and densities soar. The forces at play are extreme, leading to conditions where electrons and neutrons are compressed together. Eventually, this process may reach a critical point, resulting in a singularity—a point where density becomes infinite and the laws of physics as we know them break down. The core collapse catalyzes the transition from a star to a black hole, paving the way for one of the universe's most profound mysteries.
Accretion of Mass
After the formation of a black hole, it can continue to grow by accumulating surrounding material. This process, known as accretion, involves gas, dust, and even other celestial bodies being drawn into the black hole's gravitational influence. The material forms an accretion disk, spiraling inwards as it loses energy and angular momentum. As matter gets closer to the event horizon, it heats up, emitting x-rays and other forms of radiation. This phase is not merely passive; it is dynamic and produces observable phenomena, contributing to our understanding of black hole behavior. Observing these accretion processes provides insights into the feeding mechanisms of black holes and their impact on galactic evolution.
Understanding these processes illustrates the fundamental relationship between black holes and their host stars, establishing a link that significantly enriches our comprehension of cosmic evolution.
As we continue exploring black hole formation, it is essential to keep in mind these intricate processes. The journey from stellar life to the majestic yet daunting black hole presents a unique unfolding narrative of cosmic events that shapes the structure of the universe. Each stage provides valuable data, enhancing our grasp of the universe's complex tapestry.
Gravitational Collapse
Gravitational collapse plays a crucial role in the formation of black holes. It describes the process by which an astronomical object collapses under its own gravity. This process is essential to understand how massive stars can end their lifecycle as black holes. The dynamics of gravitational forces dictate the behavior of matter in the universe, influencing stellar development and ultimate fate.
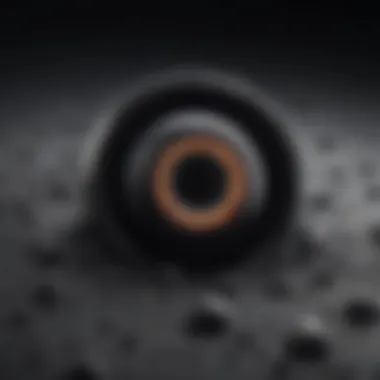
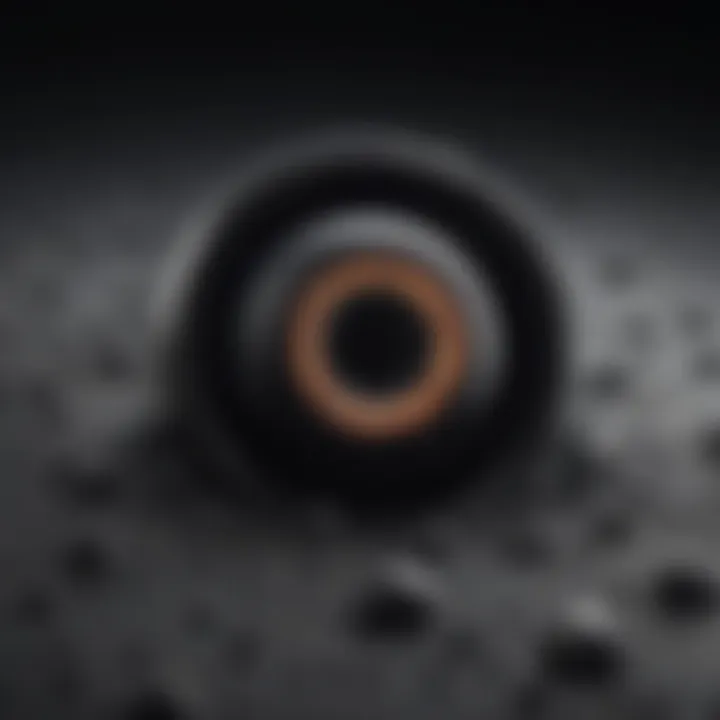
This section examines the specific elements that underlie gravitational collapse, which include the mass of an object, the distribution of matter within it, and external forces that may impact its stability. The implications of these factors can help reveal the transitional events that occur during the formation of a black hole. Knowing how gravity interacts with a star's mass allows astrophysicists to predict the conditions necessary for black hole creation.
Role of Gravity in Black Hole Creation
Gravity serves as the driving force behind black hole creation. It pulls matter together, leading to the compression of stellar material. As a massive star exhausts its nuclear fuel, it can no longer counterbalance the inward pull of gravity. At this juncture, the outer layers of the star may be expelled through a supernova explosion, while the core continues to collapse.
Throughout this process, gravity competes against the outward pressure created by nuclear reactions. Once the core loses this support, nothing can stop the gravity from pulling the remaining mass into an increasingly smaller volume. Eventually, this results in the emergence of an event horizon, marking the boundary beyond which no light or information can escape. Thus, gravity is not only a catalyst but also the defining characteristic of black holes, fundamentally influencing their formation and properties.
Singularity Concept
The concept of singularity is central to understanding black holes. A singularity refers to a point in space where the gravitational field becomes infinite, and the laws of physics as we know them cease to function predictably. In a black hole, the singularity is theorized to lie at the center, a point where all the mass of the collapsed star is concentrated. The structure of the surrounding space is fundamentally altered.
Singularities challenge our understanding of physics, particularly general relativity. As objects approach the singularity, they experience extreme gravitational effects, leading to phenomena such as time dilation. These effects reveal the limitations of our current models and theories when addressing conditions in black holes.
"The concept of singularity forces scientists to rethink the foundational principles of space and time."
Theories surrounding singularities pose significant questions regarding the ultimate fate of matter and information. This ambiguity has led to concepts such as the information paradox, prompting further studies. Understanding singularities requires a nuanced approach, blending both theoretical physics and observational astronomy.
By grasping the role of gravitational collapse and the implications of singularities, we can deepen our awareness of the universe and the enigmatic nature of black holes.
Evidence and Observations
The study of black holes is fundamentally rooted in the evidence collected through advanced observational techniques. Observations help scientists confirm the existence of black holes and understand their properties. While theoretical frameworks provide a base, it is through empirical evidence that these concepts gain credibility. This section delves into crucial elements of evidence supporting our understanding of black holes, including the innovative methods employed to visualize and detect these enigmatic objects.
Event Horizon Telescope and Imaging
The Event Horizon Telescope (EHT) represents a ground-breaking advancement in the realm of astrophysics. This global network of radio telescopes works in unison to create incredibly high-resolution images of black holes. In 2019, the EHT produced the first-ever image of a black hole, located in the center of the galaxy M87. This image provided visual confirmation of theoretical predictions regarding black holes.
The significance of the EHT extends beyond just one image. It marks a paradigm shift in observational astronomy. Data collected by this telescope array allows scientists to test aspects of General Relativity and understand gravitational effects near the event horizon. The implications of these observations have a profound effect on the astrophysical community.
- Key outcomes from the EHT include:
- Verification of the existence of black holes.
- A deeper understanding of accretion processes.
- Insight into the role of black holes in galaxy formation.
Gravitational Waves Detection
The advent of gravitational wave detectors like LIGO has opened new avenues in black hole research. These detectors observe ripples in spacetime created by merging black holes. The first detection occurred in 2015, confirming that binary black hole systems exist and can coalesce, resulting in gravitational waves detectable from Earth.
This capability has led to valuable insights into black hole populations, mass distributions, and even the behavior of neutron stars. Observations through gravitational waves provide a complementary perspective to traditional electromagnetic observations, enhancing our grasp of cosmic phenomena. Such discoveries prompt further research into the life cycles of stars and their ultimate fate as black holes.
- Key insights gained from gravitational wave detections:
- The mass range and properties of merging black holes.
- The frequency and distribution of black hole merger events.
- Correlations between merging black holes and their respective host galaxies.
Observational Challenges
Despite significant advancements in both imaging and gravitational wave detection, researchers face numerous observational challenges. The detection of black holes involves high levels of complexity. For instance, the inherent darkness of black holes hinders their direct observation. Instead, scientists rely on indirect evidence, such as the effects they have on nearby stars or gas.
The immense distances of these colossal objects also pose a challenge, as light from them takes millions of years to reach our telescopes. Additionally, distinguishing between signals from black holes and other cosmic phenomena requires advanced analytical techniques, which can sometimes lead to misinterpretations.
- Main challenges in observing black holes include:
- Limited direct visibility of the black holes themselves.
- Difficulty in measuring vast cosmic distances.
- The necessity of sophisticated technology for accurate data collection.
To conclude, gathering evidence and making observations about black holes is indispensable in black hole studies. Techniques such as the Event Horizon Telescope and gravitational wave detection have revolutionized our understanding while also highlighting the challenges that remain in the field.
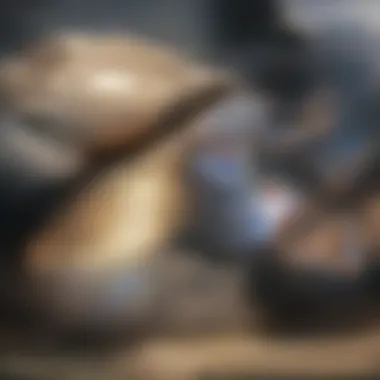
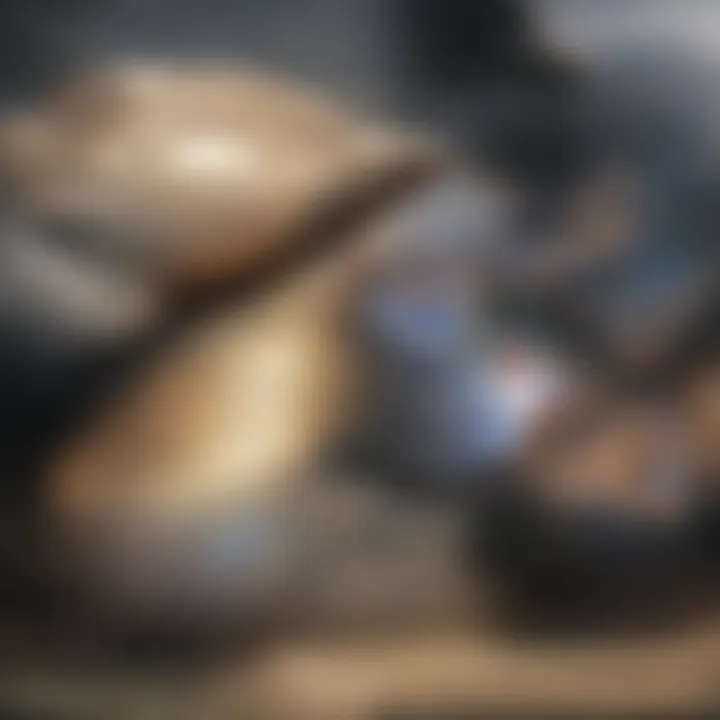
Theoretical Implications
The theoretical implications of black hole formation encompass several core concepts that challenge our understanding of physics and the universe. These implications have profound effects not only on astrophysics but also on the fundamental laws of nature. By examining how black holes interact with general relativity, the nature of information, and the role of Hawking radiation, we delve into a realm that raises critical questions about reality itself.
Black Holes and General Relativity
General relativity, proposed by Albert Einstein, describes how mass can warp spacetime. This warping creates gravitational wells, where massive objects like black holes exist. A black hole's event horizon marks the boundary beyond which nothing, not even light, can escape. The formation of a black hole, therefore, is a testament to the predictions made by this theory.
In black hole formation, the core collapse of a massive star leads to a concentration of mass at a point called a singularity. Here, the laws of physics as we presently understand them break down. General relativity predicts these singularities exist, yet their true nature remains ambiguous. This discordance underlines the importance of studying black holes, as they may hold clues to a more complete theory of quantum gravity.
Information Paradox
The information paradox presents a significant dilemma in theoretical physics. According to quantum mechanics, information is never lost. However, when an object crosses the event horizon of a black hole, it appears to vanish completely, suggesting that its information is lost. This contradiction poses challenges for scientists.
Two prevailing theories attempt to resolve this paradox. One suggests that information is stored on the event horizon itself, while another proposes that it escapes the black hole in some form. Understanding which theory holds true could radically alter our view of the universe, leading to advances in quantum theory.
Hawking Radiation
Stephen Hawking introduced the concept of Hawking radiation in the 1970s. This process occurs when virtual particles near the event horizon result in one particle escaping while the other is absorbed by the black hole. Consequently, black holes can slowly lose mass over time, leading to the possibility of their evaporation. This discovery was groundbreaking as it suggested that black holes are not entirely black and can emit radiation.
Hawking radiation challenges the conventional view of black holes as ultimate sinks for matter and information. It opens avenues of thought regarding the fate of black holes and their eventual role in the cosmos.
Ultimately, the exploration of theoretical implications surrounding black holes serves to advance our knowledge of the universe and the laws that govern it.
Understanding these concepts helps to frame future research directions. It reveals a complex interplay between established physics and new theories, insisting upon a broader perspective of our cosmic reality.
As we continue to study the implications of black holes, we might find pathways toward further innovations in theoretical astrophysics.
Future Research Directions
The exploration of black hole formation is a continually evolving field. Therefore, the future research directions in this domain are crucial, as they can lead to significant advancements in our understanding. As new technologies emerge and theories develop, researchers must identify and capitalize on these opportunities for deeper inquiry.
Technological Advancements in Astrophysics
Technological innovation plays a critical role in advancing astrophysical research. Over the years, tools like the Event Horizon Telescope have made groundbreaking discoveries, including the first direct image of a black hole.
The push for more sensitive detectors and larger, more coordinated observational platforms can lead to even clearer images and data collection. New instrumentation, such as more powerful telescopes capable of multi-wavelength observations, can provide a better understanding of the environments surrounding black holes.
Additionally, advancements in computational methods allow scientists to model complex interactions in greater detail. High-performance computing enables the simulation of various scenarios of black hole formation and their subsequent evolution. Improved algorithms could offer insights that were previously unattainable.
The Role of Black Holes in Cosmology
Black holes are fundamental to the fabric of the universe. They influence galactic formation and evolution. Understanding their role in cosmology can unlock secrets about the origins and destiny of the universe.
Research indicates that supermassive black holes are present at the centers of most galaxies, including our Milky Way. Their gravitational influence may dictate the structure and dynamics of galaxies. Thus, studying black holes can help clarify how galaxies form and evolve over time.
Moreover, exploring the relationship between dark matter and black holes remains a key area of interest. Insights into these connections may further elucidate the fundamental nature of the cosmos. Understanding how black holes interact with surrounding matter can shed light on the high-energy processes occurring in the universe.
"The study of black holes not only enhances our knowledge of these celestial phenomena but also greatly informs our understanding of the universe as a whole."
End
The study of black holes is not just a scientific curiosity; it has profound implications for our understanding of the universe. Black holes, as regions of spacetime exhibiting such strong gravitational effects that nothing can escape from them, challenge our conventional notions of physics. This article's exploration details various black hole types and the intricate processes leading to their formation.
Summary of Key Points
In summation, several critical features about black holes emerge through comprehensive analysis:
- Formation Mechanisms: Black holes can form from the remnants of massive stars after supernova explosions or through the gravitational collapse of gas clouds in the early universe.
- Types of Black Holes: The classification ranges from stellar black holes created from single stars to supermassive black holes found at galactic centers.
- Gravitational Dynamics: The role of gravitational collapse and the significance of singularity presents fundamental challenges for physicists.
- Observational Evidence: Telescopes and gravitational wave detectors provide essential evidence of black holes’ existence, forming a bridge between theoretical physics and empirical observation.
- Future Research: As technology advances, astrophysics stands to gain significantly from further research on black holes, impacting cosmology and our overall perception of the universe.
The Continuing Mystery of Black Holes
Despite numerous discoveries, black holes remain enigmatic. Several key questions persist:
- Nature of Singularity: What exactly occurs at the center of a black hole? The singularity remains one of the biggest mysteries in modern physics.
- Information Paradox: Can information that crosses the event horizon ever be retrieved? This paradox poses a challenge to quantum mechanics and general relativity.
- Hawking Radiation: The theoretical prediction by Stephen Hawking that black holes emit radiation raises questions about black hole evaporation and the ultimate fate of these cosmic entities.
As we deepen our exploration of black holes, the implications extend far beyond just theoretical understanding; they touch upon the very fabric of reality itself. The mysteries of black holes prompt us to reconsider fundamental principles of physics and ultimately encourage an ongoing quest for knowledge in the field of astrophysics.