Gamma Radiation Shielding: Principles and Applications
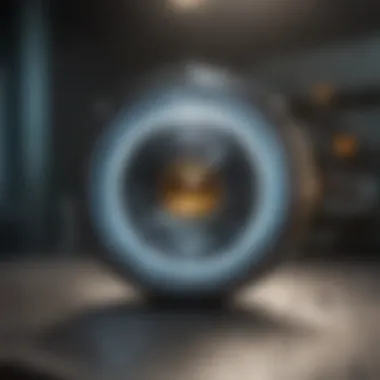
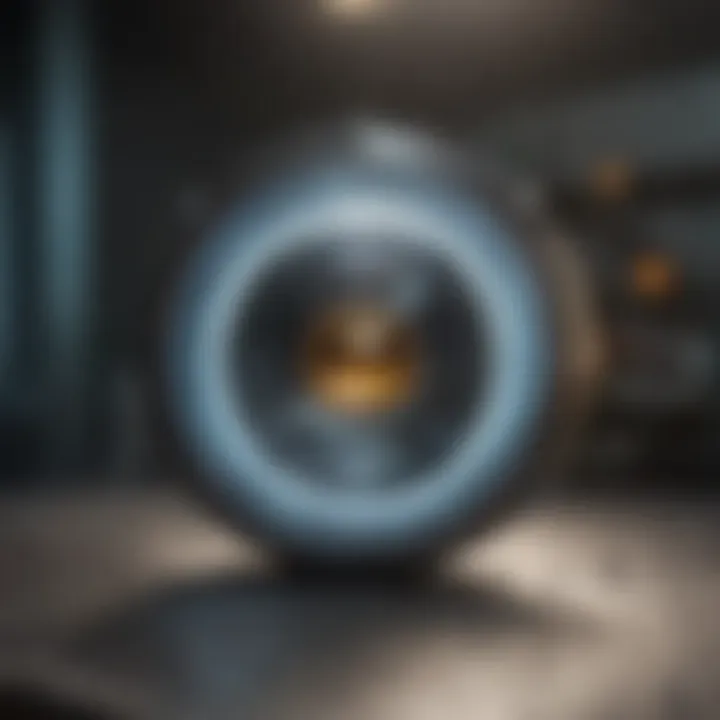
Research Overview
Gamma radiation shielding is a critical subject in the fields of radiation protection, nuclear energy, and medical imaging. Understanding how to effectively shield against gamma rays can have a significant impact on safety protocols and health standards across various industries. This section delves into key findings and the background that contextualizes the importance of gamma radiation shielding.
Summary of Key Findings
Research indicates that gamma rays are highly penetrating electromagnetic waves, which makes them particularly challenging to shield against. Effective shielding typically requires dense materials that can absorb or deflect these rays. Lead and concrete are commonly used but are not without flaws, such as weight and cost. Recent advancements in materials science have introduced alternatives, such as composite materials and advanced polymers, which can offer new benefits in shielding efficiency.
The advancements in computational modeling also enhance our understanding of gamma radiation interactions. These models help in predicting the performance of various materials, allowing for optimized design of shielding structures.
Background and Context
Historically, the need for gamma radiation shielding arose with the advent of nuclear technology and medical imaging techniques like X-rays and gamma cameras. As gamma rays pose a risk to human health and the environment, governments and institutions worldwide developed guidelines and regulations to manage and mitigate these risks. Organizations such as the International Atomic Energy Agency (IAEA) have played a pivotal role in setting safety standards.
In recent years, the emergence of newer technologies in nuclear medicine and security has spurred research into more effective shielding materials and strategies. Understanding the interplay between gamma radiation and various shielding materials requires comprehensive studies that can translate theoretical principles into effective applications.
Methodology
The methodology for researching gamma radiation shielding involves experimental designs that can measure the effectiveness of different shielding materials under controlled conditions. Here, we explore typical approaches used in this area of research.
Experimental Design
Standardized tests often involve exposing specific shielding materials to gamma radiation sources and measuring the resultant radiation levels on the opposing side. This typically utilizes gamma spectrometry to accurately assess the intensity of radiation passing through the material. The experiments may incorporate varying thicknesses of materials and different energy levels of gamma radiation to analyze their shielding capacities.
Data Collection Techniques
Data collection in gamma radiation shielding studies often relies on advanced instrumentation to gather precise measurements. Techniques used include:
- Spectrometric Analysis: Using gamma spectrometers to quantify energy and intensity of radiation.
- Geometric Configuration: Varying the arrangement of materials to observe differences in shielding efficiency.
- Statistical Methods: Applying statistical models to evaluate the significance of results and guide future research.
Researchers often share their findings through databases and publications, contributing to a collective understanding that informs safety protocols and materials development.
Understanding Gamma Radiation
Gamma radiation plays a crucial role in various scientific and industrial fields. Understanding its nature and sources helps in developing effective shielding methods to protect individuals and the environment from its adverse effects. Gamma rays are a form of electromagnetic radiation, characterized by their high energy and penetration ability. This section delves into the fundamental attributes of gamma radiation, highlighting the importance of grasping such nuances for both practical application and safety considerations.
Nature of Gamma Rays
Gamma rays are at the high-energy end of the electromagnetic spectrum. They are distinct from other forms of radiation, such as alpha and beta radiation, due to their lack of mass and electrical charge. This unique property enables gamma rays to penetrate various materials more thoroughly, making them a significant concern in radiation safety.
Some key characteristics of gamma rays include:
- High Penetration: They can pass through most materials, requiring dense materials for effective shielding.
- No Charge: As uncharged particles, they are less likely to interact with matter, which contributes to their penetrating ability.
- Origin: Gamma rays often originate from nuclear reactions, radioactive decay, or certain astrophysical events.
The understanding of gamma ray properties is vital in fields that use radiation, such as nuclear medicine and radiology.
Sources of Gamma Radiation
Gamma radiation can emerge from several natural and artificial sources. Recognizing these sources is essential for implementing appropriate safety measures. Major sources of gamma radiation include:
- Natural Radioactive Materials: Elements like uranium and radium emit gamma rays through their decay processes.
- Cosmic Rays: Space debris and solar emissions also produce gamma radiation, which enters the Earth's atmosphere.
- Nuclear Reactions: In reactors, gamma radiation is produced owing to fission processes and neutron activation.
- Medical Applications: In medical treatments, isotopes such as cobalt-60 and cesium-137 are used in radiotherapy, contributing to localized gamma radiation in clinical settings.
Awareness of these sources aids in the design of containment strategies, minimizing exposure to hazardous levels.
Biological Effects of Gamma Radiation
The biological impact of gamma radiation is particularly concerning due to its ability to penetrate body tissues. Exposure can lead to various health issues, depending on the radiation doses and duration of exposure. Immediate effects might include skin damage, while long-term exposure can lead to serious conditions such as cancer.
Some potential biological effects are:
- Cellular Damage: High-energy gamma rays can ionize atoms, leading to cellular and DNA damage.
- Radiation Sickness: Acute exposure can cause symptoms like nausea, fatigue, and in severe cases, death.
- Carcinogenesis: Long-term exposure increases the risk of cancer development, necessitating strict safety protocols in radiation-related environments.
Understanding the biological effects of gamma radiation is critical for safety in medicine and industry. Knowledge in this area helps in crafting effective shielding strategies to mitigate health risks.
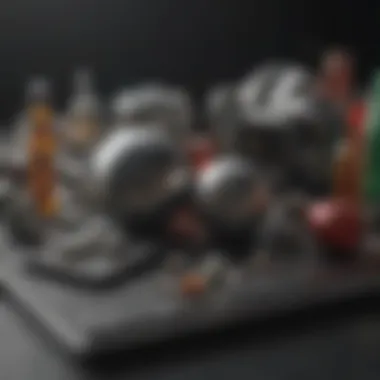
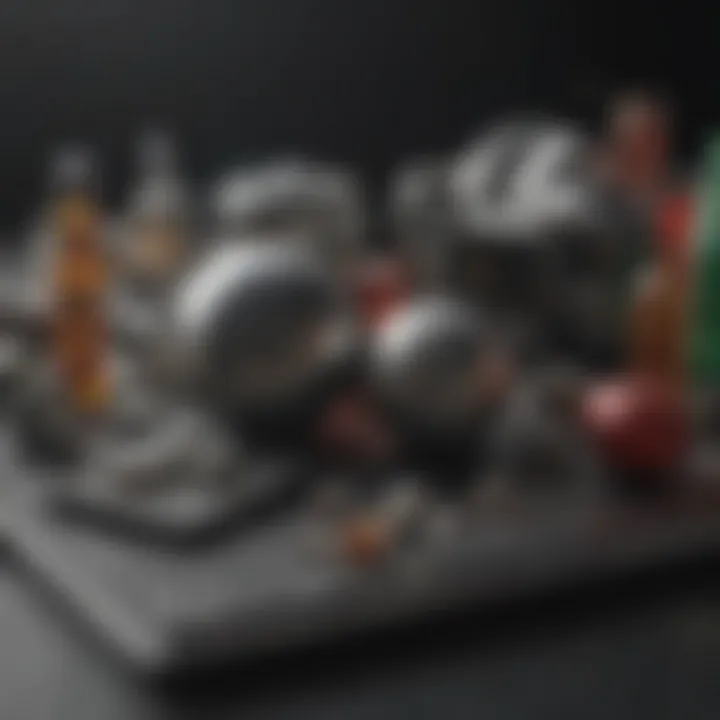
This section lays the groundwork for discussing shielding principles and applications later in this article. Understanding advanced concepts in gamma radiation will empower professionals to create safer environments across various domains.
Principles of Radiation Shielding
Understanding the principles of radiation shielding is pivotal to ensure safe handling and exposure to gamma radiation. These principles are rooted in the need to protect individuals and the environment from the adverse effects of gamma rays. Gamma radiation is unique due to its high energy and penetration ability, making effective shielding a necessity in various applications, from medical settings to nuclear facilities. This section explores the foundational concepts that underpin radiation protection, the mechanisms involved in the attenuation of gamma rays, and the significance of the half-value layer in shielding design.
Key Concepts in Radiation Protection
Effective radiation protection is based on several core concepts that help to minimize exposure to harmful radiation. Key elements include:
- Distance: Increasing distance from the source of radiation reduces exposure significantly. It is often the simplest and most effective measure employed in radiation protection.
- Time: Reducing the time spent near a radiation source is a fundamental principle. By minimizing exposure duration, one can decrease the total dose received.
- Shielding: The application of material barriers, such as lead or concrete, is essential in blocking or attenuating gamma radiation. The choice of shielding material depends on its density and atomic number, as these characteristics influence its effectiveness.
Adhering to these principles not only protects human health but also ensures compliance with safety regulations and guidelines. Each of these concepts plays a critical role in the design of shielding solutions tailored to specific environments and applications.
Attenuation of Gamma Rays
Attenuation is the process through which gamma rays lose intensity as they travel through a material. This reduction in intensity occurs due to absorption and scattering events, which are influenced by the properties of the shielding material. The rate of attenuation is governed by several factors, which include:
- The type of material: Denser materials with higher atomic numbers tend to absorb more gamma radiation. Lead, for example, is widely utilized due to its high density and effective shielding properties.
- The energy of the gamma rays: Higher energy gamma rays penetrate more deeply into materials. Therefore, materials must be selected based on the energy levels of the gamma radiation present.
- The thickness of the material: Increasing the thickness increases the likelihood of interactions between gamma rays and the shield material. Thus, thicker shields generally provide better attenuation.
Overall, understanding these factors is vital in the selection and design of appropriate shielding materials to effectively reduce radiation exposure.
Half-Value Layer and Its Importance
The half-value layer (HVL) is a crucial concept in radiation shielding, representing the thickness of a specific material required to reduce the intensity of gamma radiation by half. Understanding HVL is necessary for several reasons:
- Material Selection: By knowing the HVL for a given material, engineers can decide on the thickness required to achieve desired levels of protection. This can lead to cost-effective and efficient use of materials.
- Design Calculations: In practical applications, the HVL assists in calculating the necessary shielding configurations for various radiation sources. This is particularly important in environments where compliance with safety standards is critical.
- Comparative Analysis: Assessing the HVLs of different materials allows for the comparison of their efficacy in shielding gamma radiation. Understanding which material provides superior protection is vital in optimizing design solutions.
Knowing the half-value layer not only aids in the scientific understanding of radiation shielding but also facilitates the implementation of practical shielding solutions tailored for specific safety requirements.
"Effective shielding is a blend of physical principles and material science, aiming to protect health and safety in environments exposed to high radiation levels."
In essence, the principles of radiation shielding form the foundation of effective safety measures that protect both humans and the environment from the penetrating effects of gamma radiation.
Materials for Gamma Radiation Shielding
The selection of materials for gamma radiation shielding is a critical aspect of radiation protection. Different materials have unique properties that contribute to their effectiveness against gamma rays. The effectiveness of shielding materials is measured by their ability to attenuate gamma radiation, ensuring that exposure levels remain within safe limits. Understanding the properties and applications of various shielding materials helps in making informed decisions in both medical and industrial settings.
Common Shielding Materials
Lead
Lead is one of the most commonly used materials for gamma radiation shielding. Its high density and atomic number make it particularly effective in attenuating gamma rays. One key characteristic of lead is its ability to reduce radiation by a significant percentage with relatively thin layers. For example, a mere 1 inch of lead can absorb a substantial amount of gamma radiation.
However, the use of lead comes with some considerations. While it is effective, lead is also heavy, which can complicate transportation and installation. Additionally, its toxicity poses health risks, especially in environments where it could be ingested or inhaled. Therefore, while lead is a popular choice for shielding, careful handling and planning are necessary.
Concrete
Concrete is another widely used material for gamma radiation shielding. Its primary advantage is its abundance and low cost compared to other materials. Concrete's density can be adjusted by mixing it with heavy aggregates, enhancing its shielding capabilities. A notable feature of concrete is its ability to be molded into various shapes, making it versatile for construction in shielding applications.
Despite these advantages, concrete has a limitation. Its effectiveness is generally lower than that of lead. Therefore, it may require thicker walls to achieve the same level of radiation protection. Thus, while concrete is beneficial for large structures, its use may be less effective in compact settings where space is at a premium.
Water
Water serves as an efficient material for gamma radiation shielding in certain applications, particularly in nuclear reactors. Its primary attraction lies in its ability to absorb radiation, making it invaluable in cooling systems where heat dissipation is necessary. Water is also relatively inexpensive and widely available.
However, water presents its own challenges. Its shielding effectiveness can be less than that of more dense materials. In addition, water can be a logistical challenge in some environments, particularly in areas with limited access to resources for managing and storing large volumes. Nevertheless, it remains a crucial component in many shielding systems, especially in temporary setups.
Gypsum
Gypsum board is increasingly recognized for its effectiveness in shielding against gamma radiation. Its unique property is its ability to provide a barrier to radiation while also acting as a fire retardant. Gypsum is relatively lightweight and easy to handle, making it a favorable choice for various construction applications.
However, gypsum has its drawbacks. The shielding potential is generally lower than that of lead and concrete, and its application may be limited in high-radiation environments. Therefore, while gypsum can be beneficial in specific applications, it often needs to be used in conjunction with other shielding materials to ensure adequate protection.
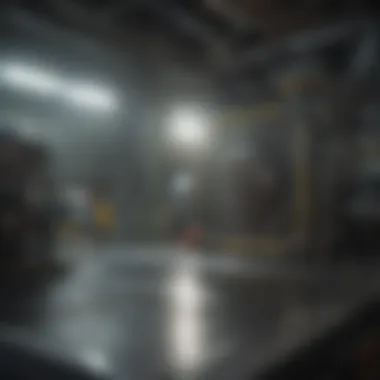
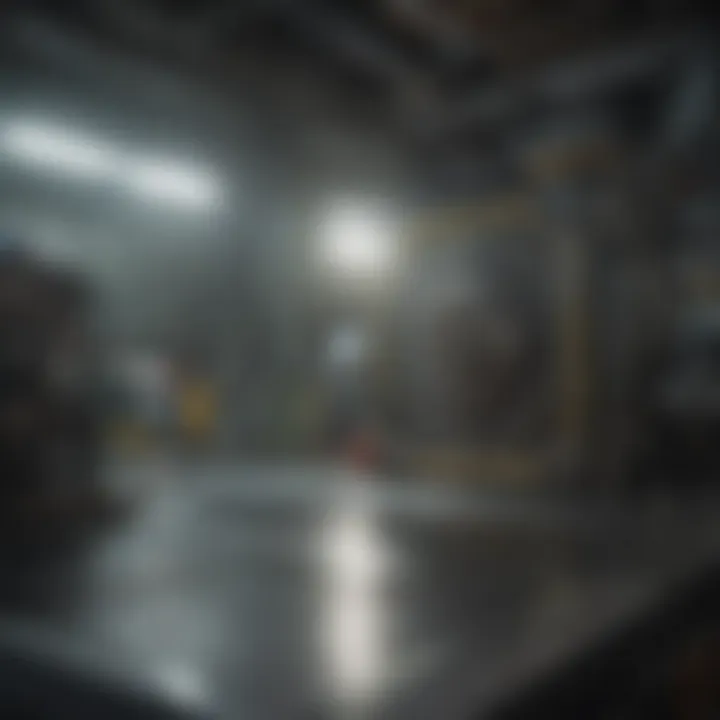
Innovative Shielding Materials
Polymer Composites
Polymer composites are gaining attention for their use in radiation shielding. They combine polymers with other materials to improve performance. A key advantage of these composites is their lightweight nature compared to traditional materials like lead or concrete. This can facilitate easier application and handling.
Furthermore, polymer composites can be engineered for specific radiation protection requirements, offering flexibility in design. However, their long-term effectiveness and durability under extreme conditions require further investigation to fully understand their potential in broad applications.
Nanomaterials
Nanomaterials have emerged as promising candidates for gamma radiation shielding. Due to their extremely small size, they offer an increased surface area, which enhances their interaction with gamma rays. This characteristic can lead to improved protection levels using lesser material.
Despite their potential, the production and cost of nanomaterials can be significant barriers. Ongoing research aims to alleviate these challenges, demonstrating that while nanomaterials offer exciting possibilities, practical implementation still requires careful consideration.
Metallic Glasses
Metallic glasses are an interesting approach in gamma radiation shielding. These materials exhibit high strength and a unique atomic structure that provides enhanced protection properties. They can be thinner while maintaining effective shielding performance, which is a significant advantage in space-sensitive environments.
However, metallic glasses are still under research for their broad application. While they promise high effectiveness, their cost and production methods can limit their immediate use as shielding materials in some settings. Thus, while they show promise, more exploration is necessary to realize their full potential in real-world applications.
Design Considerations for Shielding
Design considerations for shielding against gamma radiation play a crucial role in ensuring effective protection and safety in environments where exposure risk exists. Understanding these design elements enhances the efficiency of shielding setups, providing more robust protection against gamma rays. Key factors include shielding configuration, thickness, geometry, and safety factor analyses. Each of these elements not only influences design choices but also impacts overall efficacy in applications.
Shielding Configuration
The configuration of the shielding material is significant. Depending on the source of radiation and the intended protection level, the arrangement can vary considerably. For instance, a combination of layers may be used, where various materials are stacked to utilize their distinct attenuation properties. When designing the configuration, it is important to assess the layout in relation to potential exposure scenarios. This involves understanding the radiation source angle and the distances involved. Proper configuration minimizes unnecessary exposure and maximizes the shield's effectiveness.
Thickness and Geometry of Shielding
The thickness of shielding material directly correlates with its ability to attenuate gamma radiation. To achieve desired protection levels, designers must calculate the required thickness based on the radiation type and intensity. Employing the half-value layer concept provides insight into how thick a given material needs to be to reduce gamma radiation to half its intensity. Geometry also contributes to how effective the shielding is. Flat panels, large containers, or cylindrical designs can all have distinct effects on radiation attenuation. Ultimately, adequately assessing both thickness and geometry ensures a customized approach tailored to specific needs.
Safety Factor Analysis
In any shielding design, safety factors are essential. These factors consider the uncertainties inherent in calculations, such as variations in radiation levels, material properties, and potential exposure durations. By incorporating a safety margin in the shielding design, professionals can account for risk and enhance reliability. This proactive approach reassures stakeholders that the shielding design can withstand unexpected conditions while still providing ample protection.
Safeguarding against gamma radiation is not just about following protocols; it's about creating thoughtful designs that prioritize people's safety and environmental health.
Applications of Gamma Radiation Shielding
The applications of gamma radiation shielding are critical to safeguarding health and the environment in various fields. Understanding the importance of these applications allows us to appreciate how effective shielding can mitigate exposure to harmful rays. The significance lies not only in the protection that these applications offer but also in the advancing methods and technologies that are continually being developed to enhance safety and efficacy.
Medical Applications
Radiotherapy
Radiotherapy is a key application of gamma radiation shielding in medicine. It uses high doses of radiation to kill cancer cells or shrink tumors. The shielding involved is essential for protecting healthy tissues surrounding the targeted areas. One key characteristic of radiotherapy is its targeted delivery system, which aims to minimize exposure to surrounding cells.
The beneficial aspect of radiotherapy is its effectiveness in treating a wide range of cancers. Also, advancements in techniques like IMRT (Intensity-Modulated Radiation Therapy) have improved precision. However, the unique feature lies in the careful planning required to determine the appropriate shielding materials and configurations to ensure maximum efficacy while minimizing risks. The disadvantage includes the potential for side effects, which underscores the need for effective shielding.
Diagnostic Imaging
In the realm of diagnostic imaging, gamma radiation plays a significant role, especially in procedures like PET scans. These scans help in detecting diseases, assessing organ function, and planning treatments. The key characteristic of this application is its non-invasive nature, allowing for detailed internal examinations without the need for surgery.
Diagnostic imaging is a popular choice because it provides crucial information for diagnosis. Moreover, the technology has advanced rapidly, leading to improved image quality and reduced radiation doses. The unique feature in this field involves the shielding required to protect healthcare workers and patients from unnecessary exposure. However, a disadvantage is the cost of implementing advanced shielding solutions, which can be substantial but ultimately necessary for safety.
Industrial Applications
Nuclear Facilities
Gamma radiation shielding is vital in nuclear facilities, where radioactive materials are handled regularly. The role of shielding is to protect workers and the environment from excessive exposure. A key characteristic of these facilities is the high-level containment of radioactive substances. This containment must be coupled with effective shielding strategies to ensure safety.
The beneficial aspect of shielding in nuclear facilities is the control it affords over radiation exposure, which is critical in maintaining compliance with safety regulations. A unique feature of this application is the extensive use of heavy shielding materials like lead and concrete. However, disadvantages may include the complexities and costs involved in maintaining shielding integrity over time, especially in older facilities.
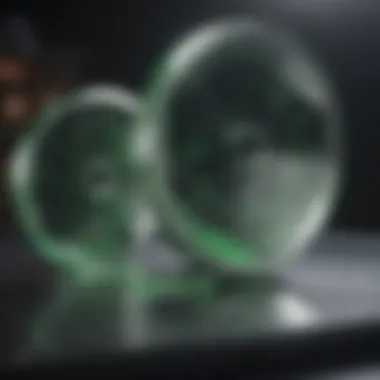
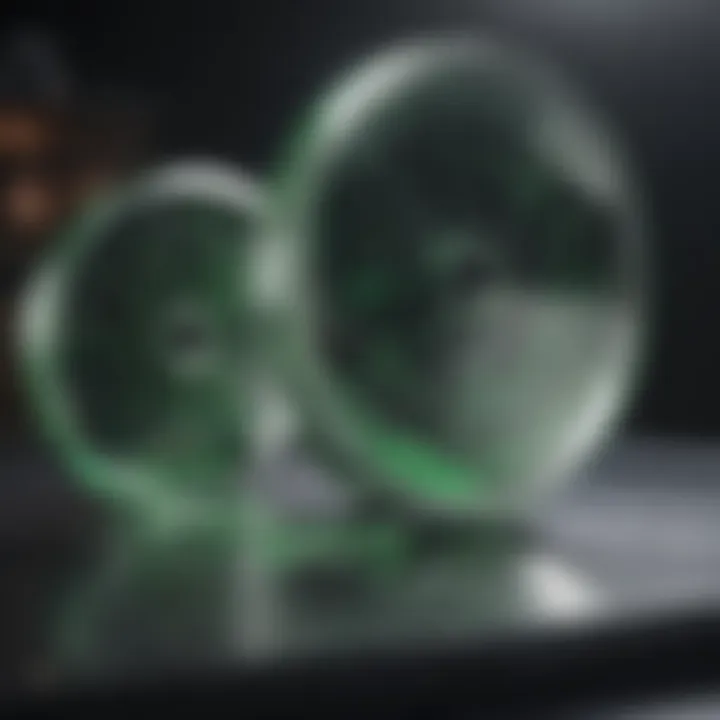
Research Laboratories
Research laboratories often engage in experiments involving radioactive substances. Here, gamma radiation shielding is necessary to safeguard personnel and visitors. The primary focus in this setting is to establish protocols that minimize exposure while allowing for groundbreaking research to continue.
The key characteristic is flexibility, as the shielding must adapt to various experiments. This adaptability makes it a beneficial choice for research. A unique feature of laboratory settings is the implementation of modular shielding that can be adjusted as per experimental needs. While this approach enhances functionality, a disadvantage might be the time and resources required for constant assessments of shielding efficacy.
Environmental Protection
Radioactive Waste Management
In radioactive waste management, gamma radiation shielding is indispensable for protecting both people and the environment. The focus here is on containment and safe disposal of radioactive waste, which poses long-term risks. The key characteristic involves the use of various materials to create barriers that prevent radiation from leaking into the environment.
The beneficial aspect of applying shielding in waste management is the reduced health risk to communities living near disposal sites. A unique feature involves the long-term plans for monitoring and maintaining containment systems. The disadvantage arises from the ongoing costs and complexities involved in ensuring the integrity of these systems over time.
Decommissioning of Nuclear Plants
Decommissioning nuclear plants involves carefully dismantling facilities and managing residual radioactive materials. Effective gamma radiation shielding is crucial during this process to protect workers and the surrounding environment. One key characteristic is the stringent safety measures employed throughout the decommissioning phases.
This rigorous approach makes it a beneficial choice for ensuring safety standards are met. The shielding must effectively contain radiation during both active dismantling and the long-term storage of radioactive materials. A unique feature is the detailed planning and execution strategies employed, which require substantial investment. However, a disadvantage is the potential environmental impact if shielding measures fail.
By understanding these applications, one grasps the true value of gamma radiation shielding across multiple fields. The need for advanced materials, innovative designs, and constant regulatory compliance fosters an environment ripe for ongoing research and development.
Regulatory Framework and Standards
The regulatory framework and standards surrounding gamma radiation shielding play a vital role in ensuring the safety of individuals and the environment. These guidelines are not arbitrary; they are derived from numerous studies and experiences that reflect the need to mitigate risks associated with exposure to gamma radiation. The importance of these regulations extends beyond mere compliance; they foster a culture of safety and accountability in industries that deal with radioactive materials.
The development of a robust regulatory framework involves both national and international standards, which together provide clear directives for safety practices, monitoring procedures, and material usage. Such frameworks aid in establishing best practices, complying with legislation, and addressing public concerns about radiation exposure.
International Guidelines
International guidelines are established by organizations such as the United Nations Scientific Committee on the Effects of Atomic Radiation (UNSCEAR) and the International Atomic Energy Agency (IAEA). These bodies provide comprehensive recommendations based on scientific evidence for the protection of people and the environment from harmful radiation.
- Safety Principles: These guidelines generally emphasize the principles of justification, optimization, and dose limitation. This means all radiation exposure must be justified, kept as low as reasonably achievable, and within acceptable limits.
- Radiation Protection Recommendations: The IAEAβs Safety Standards are essential for regulatory frameworks worldwide, outlining the obligations and rights of workers in industries that involve radiation exposure.
- Training and Competence: International guidelines also stress the importance of adequate training for professionals involved in radiation protection, ensuring they are competent to manage and mitigate risks effectively.
These principles aim to unify global efforts towards radiation safety and protection, fostering collaboration among countries to address the complexities of gamma radiation shielding.
National Regulations
National regulations further refine these international guidelines by tailoring them to the specific contexts and needs of individual countries. Each nation formulates its set of legal requirements concerning radiation protection, typically administered by governmental agencies.
- Regulatory Bodies: In the United States, for instance, the Nuclear Regulatory Commission (NRC) oversees and enforces regulations related to nuclear materials, ensuring compliance with both federal and state laws.
- Permits and Licensing: Many countries require strict licensing for facilities that utilize gamma radiation materials. This licensing process often includes thorough inspections and assessments to guarantee adherence to safety standards.
- Monitoring and Reporting: National regulations typically stipulate regular monitoring of radiation levels and require facilities to report any incidents or elevated exposure levels immediately. This transparency is crucial for public safety and trust.
The significance of adhering to these national regulations cannot be overstated. They serve not only as a shield against potential hazards but also as a framework for continuous improvement in safety practices, innovations in shielding technology, and comprehensive training for individuals involved in various sectors.
Ensuring compliance with regulatory frameworks allows organizations to cultivate a culture of safety and responsibility while advancing in gamma radiation research and technology.
Future Directions in Gamma Radiation Shielding
Gamma radiation shielding is a vital area of study that continuously evolves. With the growth of nuclear technology and environmental protection efforts, it is crucial to explore future directions in shielding methods. This section highlights the importance of ongoing research and advancements within the sector. Addressing advancements in shielding could greatly improve the safety of workers and residents in radiation-prone areas.
Research Trends
Research is a dynamic aspect of gamma radiation shielding. As new findings emerge, they often lead to significant transformations in shielding materials and strategies. Current research trends emphasize the development of advanced materials that offer enhanced shielding effectiveness while being lighter and more cost-effective.
Some relevant trends include:
- Nanomaterials: These materials, characterized by their ultra-small particle size, provide significantly improved attenuation properties compared to conventional materials.
- Composite Materials: Combining different materials allows for customized solutions tailored to specific shielding requirements. For instance, the use of lead-polymer composites may optimize weight and functionality.
- Bio-inspired Designs: Researchers are starting to look at biological systems for innovative solutions. Nature often has its ways of optimizing material use and effectiveness, which can inspire new shielding approaches.
The shift toward environmentally friendly materials is also notable. There is growing awareness about the environmental impact of traditional shielding materials, prompting focus on sustainable alternatives.
Technological Innovations
Technological innovations play a significant role in enhancing gamma radiation shielding. Integrating new technologies into the design and application of shielding can provide powerful solutions to existing problems. Some notable innovations include:
- Advanced Imaging Techniques: Improved imaging technologies, such as computed tomography (CT) scans, enable better assessment of shielding effectiveness. This helps in fine-tuning materials and configurations for specific environments.
- Simulation Software: Software tools that simulate gamma radiation environments can aid researchers in understanding interactions between radiation and shielding materials. This can lead to more efficient designs before physical prototypes are created.
- Smart Shielding Solutions: The integration of sensors into shielding materials allows for real-time monitoring of radiation levels. This advancement provides critical information that can help adapt shielding strategies dynamically.
"The future of gamma radiation shielding is intertwined with technological growth and environmental responsibility."
These points illustrate how the future of gamma radiation shielding will likely focus on materials that are not only effective but also align with contemporary concerns regarding sustainability. As the need for adequate protection against gamma radiation continues to rise, these future directions will shape the landscape of radiation safety measures.