Ion Pairs: Exploring Their Role in Chemistry and Physics
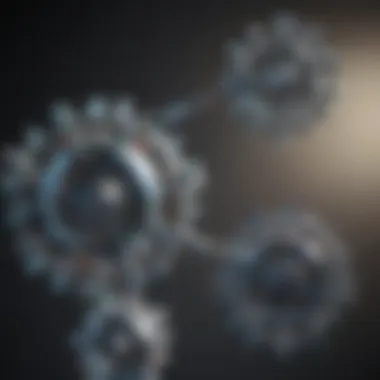
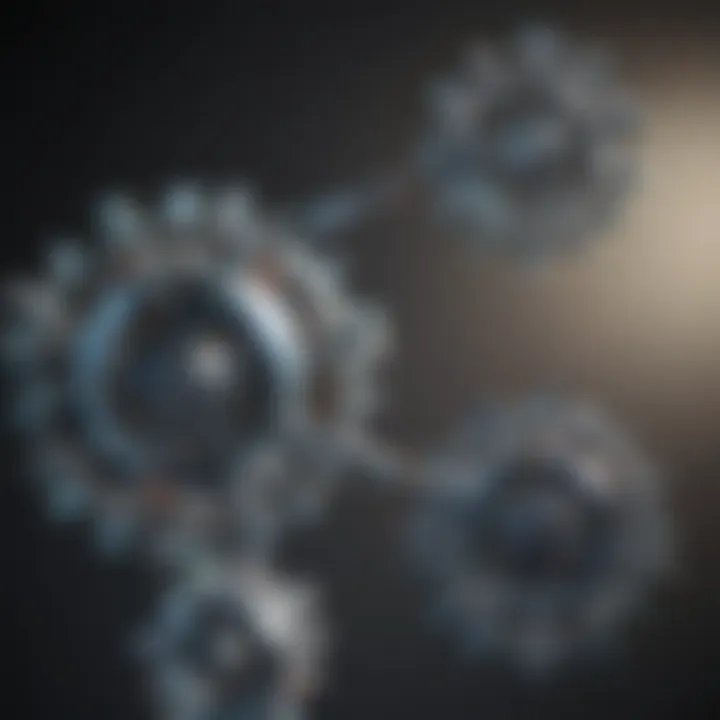
Intro
Ion pairs are fundamental entities in both chemistry and physics, transcending the boundaries of basic concepts to influence complex interactions within various systems. At their core, ion pairs consist of a cation and an anion that are juxtaposed yet engaging with each other through electrostatic forces. Their study reveals significant insights into chemical bonding, reaction mechanisms, and even biological processes.
Understanding ion pairs is essential for academics, researchers, and professionals in the field. The formation of these pairs can alter the properties of solutions, impacting solubility and reactivity. Therefore, a deep dive into ion pairs not only makes theoretical sense but has practical implications across multiple disciplines.
Research Overview
Summary of Key Findings
As the discussion unfolds, we will explore key findings around the behavior and implications of ion pairs, including:
- The classification of ion pairs into contact pairs and solvent-separated pairs.
- Their critical role in solubility and dissociation processes.
- The influence of ion pairs in biological systems, such as enzyme activities and metabolic pathways.
Background and Context
Ion pairing has a substantial historical context. Early theories of chemical bonding began acknowledging the importance of electrostatic interactions in atomic and molecular structures. Over the decades, modern advancements in spectroscopy and computational chemistry have propelled our understanding forward. Ion pairs are now recognized not just as simple interactions, but as pivotal to the dynamics of various chemical and physical processes.
Methodology
Experimental Design
The study of ion pairs prominently employs different methodologies to analyze their formation and behavior.
- Solution Chemistry Approaches: Often, researchers prepare dilute solutions containing both cations and anions, altering concentrations to observe ion pair formation.
- Spectroscopy Techniques: These methods, including NMR and infrared spectroscopy, facilitate the investigation of ion pairs at a molecular level.
Data Collection Techniques
Data collection is essential for understanding ion pairs. Common approaches include:
- Conductivity Measurements: Used to ascertain changes in ion mobility and interactions.
- Viscosity Studies: These can reveal the extent of ion pair formation by measuring the flow of the solution under various conditions.
The exploration of ion pairs aids in decoding several molecular phenomena, bridging theory and real-world applications.
This comprehensive overview sets the stage for a deeper exploration of ion pairs in subsequent sections, outlining the mechanisms of their formation, their diverse implications, and the continuing advancements in this field of study.
Prelims to Ion Pairs
In the field of chemistry and physics, the concept of ion pairs plays a pivotal role. Understanding ion pairs is essential for grasping various chemical processes and reactions that dictate the behavior of molecules in different environments. Ion pairs are particularly significant in areas like electrochemistry, biological systems, and material science. This section explores the definition and historical context leading to the current understanding of ion pairs.
Definition of Ion Pairs
Ion pairs are defined as two oppositely charged ions that are closely associated with each other. They can exist in a solution, typically formed when an ionic compound dissolves in a solvent. The positive ion, or cation, and the negative ion, or anion, interact through electrostatic forces, creating a stable unit.
Ion pairs can be categorized into distinct types based on their proximity and interaction in the solvent environment. Understanding these interactions is fundamental as they influence a wide range of properties, such as conductivity, solubility, and chemical reactivity. Moreover, ion pairs can significantly affect the kinetics of chemical reactions, making their study crucial for both theoretical and applied chemistry.
Historical Context
The study of ion pairs began in the early 20th century, when researchers first recognized the influence of ionic interactions in solutions. Early experiments focused on salt solutions, which highlighted how ions remain associated even in a dissolved state. This work was foundational, leading to a better understanding of ion behavior in various media.
As scientific techniques advanced, particularly in spectroscopy and electrochemistry, the understanding of ion pairs deepened. Researchers began to classify ion pairs more rigorously and explore their roles in reaction mechanisms and solvation dynamics. With the development of computational chemistry, the theoretical exploration of ion pairs gained momentum, allowing scientists to simulate conditions that could not be readily replicated in experimental setups.
"The interplay between theory and experiment has significantly advanced the comprehension of ion pairs, shedding light on their essential roles in numerous chemical phenomena."
In recent decades, interest in ion pairs has surged due to their implications in biological systems and materials science, making them a focal point of modern research. This background sets the stage for exploring how ion pairs form, their types, and their applications across various scientific disciplines.
Formation of Ion Pairs
The formation of ion pairs is a crucial aspect of understanding their role in both chemistry and physics. As fundamental entities, ion pairs provide insights into various chemical processes, environmental interactions, and biological functions. Identifying how ion pairs form helps in comprehending their implications on reaction mechanisms and kinetics, which is vital for both theoretical studies and practical applications.
Electrostatic Attraction
Electrostatic attraction is the first driving force behind the creation of ion pairs. This force occurs between ions of opposite charges. For example, a cation, which carries a positive charge, attracts an anion, which carries a negative charge. The strength of this attraction depends on several factors:
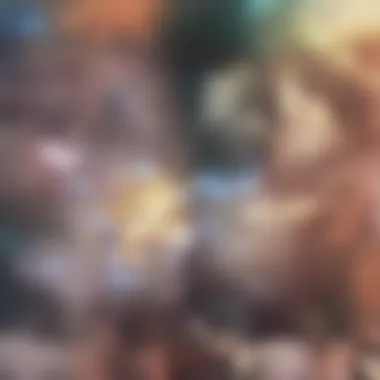
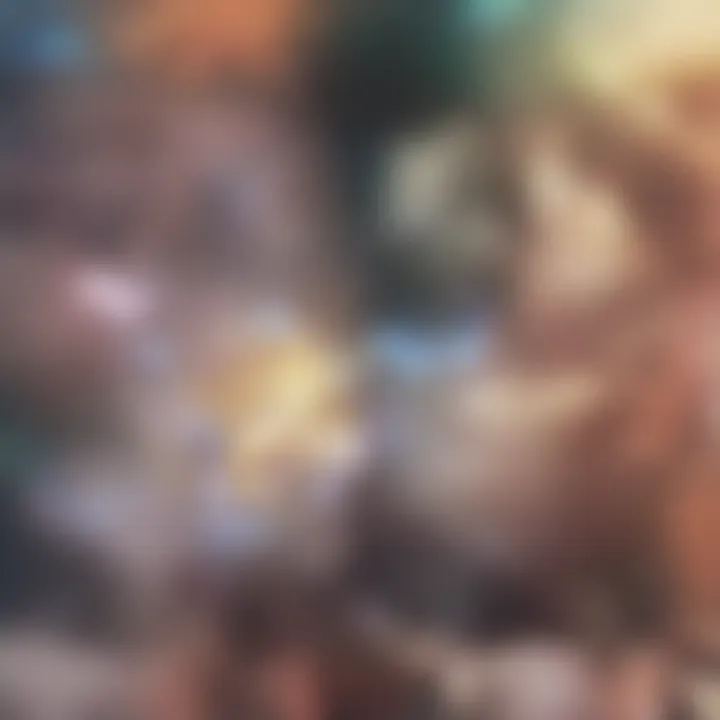
- The magnitude of the charges.
- The distance between the ions.
- The dielectric constant of the surrounding medium.
These factors combined determine the stability of the ion pair. In many cases, the electrostatic interaction is strong enough to overcome the thermal motion of the ions, allowing them to exist as a pair in solutions.
Solvation Effects
Solvation, or the interaction of solvent molecules with solute ions, plays a significant role in the formation and stabilization of ion pairs. When an ion enters a solvent, it is surrounded by solvent molecules. This process can either promote or inhibit ion pair formation depending on the solvent's characteristics. Hydration for example can stabilize ion pairs through the formation of a solvation shell surrounding each ion. This transformation changes the effective interaction radius of the ions, allowing for greater stability within the solvent.
Additionally, the nature of the solvent affects the balance between ion pairing and free ions. For polar solvents like water, strong solvation can diminish the formation of ion pairs, whereas in less polar solvents, ion pairs can exist more freely. Understanding solvation effects is essential for predicting the behavior of ions in various chemical environments.
Temperature Dependence
Temperature significantly influences ion pair formation. As temperature increases, thermal energy also increases, which impacts the movement of ions and their capacity to overcome electrostatic attractions. Higher temperatures often lead to increased ion mobility, which can reduce the likelihood of ion pairing due to greater distances between charged entities. Conversely, lowering the temperature can enhance ion pairing under conducive conditions as the kinetic energy of the ions decreases, allowing electrostatic forces to dominate.
In summary, the formation of ion pairs is influenced by electrostatic attraction, solvation effects, and temperature dependence. Each of these factors plays an integral role in the dynamics of ion interactions. Understanding these elements provides a more holistic view of ion pairs' presence in chemical and physical systems, establishing a groundwork for further exploration and research.
Classification of Ion Pairs
Understanding the classification of ion pairs is essential for several reasons. Firstly, it allows chemists and physicists to predict how ions behave in various environments. Different types of ion pairs can have significant variations in their interaction strengths, stability, and reactivity. This classification enables researchers to tailor their approaches in both experimental setups and theoretical models.
Moreover, the classification aids in the interpretation of experimental data. By knowing the characteristics of contact ion pairs, solvent-separated ion pairs, and triple ion pairs, scientists can better analyze their findings in terms of ion behavior in reactions and solutions. Each type of ion pair offers insights into the fundamental interactions that occur at the molecular level.
Furthermore, when dealing with applications in fields like material science and biological systems, recognizing the type of ion pair present can inform choices for materials and methods. In this section, we will discuss three key types of ion pairs: contact ion pairs, solvent-separated ion pairs, and triple ion pairs.
Contact Ion Pairs
Contact ion pairs form when oppositely charged ions are very close to each other, often within a distance that allows them to affect each other's charge properties directly. This close proximity can lead to strong electrostatic interactions, resulting in a tightly bound structure that behaves almost like a neutral molecule. The study of contact ion pairs is crucial because they often play a significant role in reaction mechanisms.
For example, in many organic reactions, contact ion pairs can facilitate the transfer of electrons between reactants. Their presence can influence the energy landscape of a reaction, thereby impacting its kinetics. Understanding contact ion pairs is also valuable for designing new catalysts and optimizing reaction conditions.
Solvent-separated Ion Pairs
Solvent-separated ion pairs are characterized by a noticeable distance between the ions, separated by one or more solvent molecules. This configuration reduces the intensity of direct electrostatic interactions compared to contact ion pairs. The presence of solvent mediates interactions and alters the stability of these ion pairs. They are important in many electrochemical processes where solvation effects determine ion mobility and conductivity.
In biochemical systems, solvent-separated ion pairs can influence the binding affinities of substrates and enzymes, thereby affecting biochemical pathways. Understanding these dynamics can lead to innovations in drug design and enzyme engineering.
Triple Ion Pairs
Triple ion pairs consist of two ions of opposite charges and a third ion that can either be charged or neutral. This configuration often arises under high ionic strength conditions where interactions are significantly altered due to the presence of additional ions in the vicinity. The dynamics of triple ion pairs offer unique insights into complex systems, such as those observed in ionic liquids and highly concentrated electrolytes.
The role of triple ion pairs becomes relevant in processes like ion transport across membranes or conductance in electrolytic cells. By understanding their behavior, chemists can enhance the efficiencies of various applications, from energy storage to separation technologies.
In summary, the classification of ion pairs provides essential insights into the behavior of ions in different environments and contexts. Each type of ion pair has distinct characteristics that can greatly influence chemical and physical phenomena. This understanding is vital for both academia and industry, paving the way for advancements in various scientific fields.
Ion Pairs in Chemical Reactions
Ion pairs are fundamental to the mechanistic understanding of chemical reactions. Their presence can alter reaction pathways and influence the speed at which reactions occur. Recognizing the significance of ion pairs in chemical processes provides insights on how reactions are orchestrated at molecular levels. Several aspects merit attention in this context, focusing on their roles in reaction mechanisms and their impact on reaction kinetics.
Role in Reaction Mechanisms
Ion pairs often act as intermediates within reaction mechanisms. In many cases, the interaction between cations and anions can effectively stabilize transition states, which are crucial for determining whether a reaction proceeds or not. This stabilization can occur through various means, such as electrostatic interactions or specific solvation effects.
Different ionic combinations can result in different reaction pathways. For instance, consider the nucleophilic substitution reactions occurring in organic chemistry. The presence of ion pairs can lead to either a bimolecular mechanism or a unimolecular mechanism depending on the concentration and nature of the reactants. This variability is significant since it allows chemists to predict and control outcomes in synthetic pathways.
Moreover, ion pairs can be crucial in redox reactions where charge transfer occurs. The reformation of ion pairs may facilitate electron transfer, enhancing reaction efficiency. This interaction can impact the formation of products and increase the formation rate. Ultimately, understanding their role assists in the prediction of reaction mechanisms.
Influence on Reaction Kinetics
The influence of ion pairs on reaction kinetics cannot be understated. They can affect the effective rate of reactions, altering both the activation energies and overall reaction rates. When an ion pair forms, it can lead to reduced collision frequency of reactants, impacting how quickly a chemical change occurs.
For example, in ionic solutions, the formation of ion pairs may increase the viscosity of the solution. This change can impede reactant mobility, resulting in a slower kinetic response in comparison to reactions where ion pairs are not significant.
Additionally, temperature plays a crucial role in ion pair interactions. As temperature rises, the kinetic energy of particles also increases, often diminishing the stability of these pairs. In turn, this dynamics can lead to a more rapid dissociation of ion pairs, allowing for faster reaction kinetics under certain conditions. Consequently, understanding how ion pairs interact and their influence on reaction kinetics offers profound insights into numerous chemical processes, from organic syntheses to biological mechanisms.
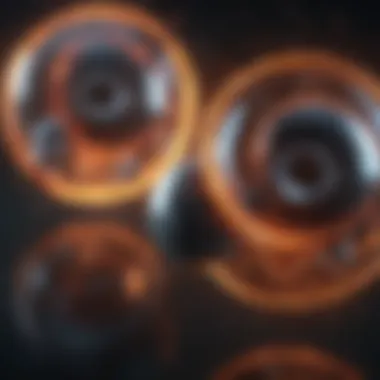
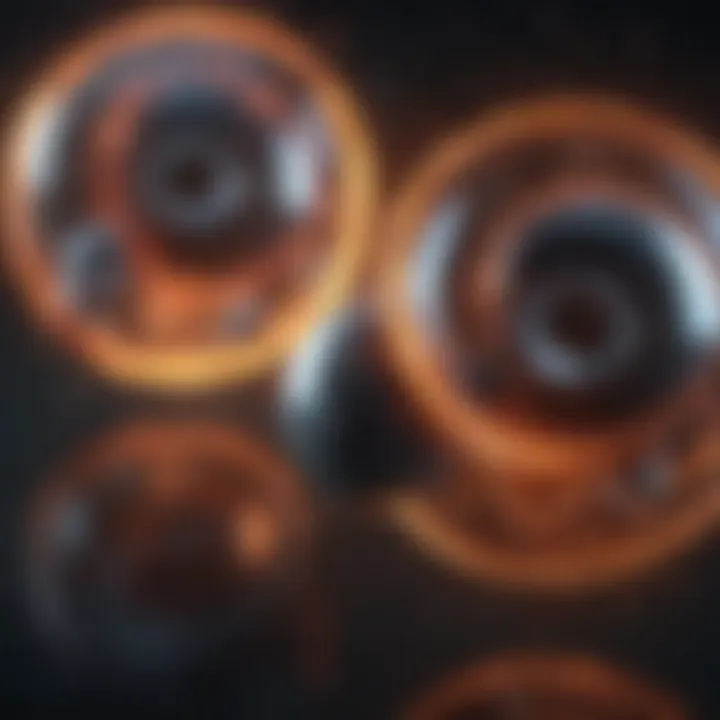
"Ion pairs are not just spectators in chemical reactions; they actively shape the entire process, influencing everything from reaction rates to product formation."
This emphasizes a need for a nuanced understanding of ion pairs when studying chemical reactions.
Applications of Ion Pairs
Ion pairs play a pivotal role in various fields, bridging theoretical concepts with practical applications. Their significance spans across biological systems, analytical chemistry, and material science. Understanding how ion pairs operate enhances our grasp of both natural processes and engineered systems. This section explores specific applications, elucidating the intrinsic benefits and considerations that arise from the interplay between ion pairs and their environments.
In Biological Systems
In biological contexts, ion pairs are crucial for processes such as enzyme function and protein stability. For instance, many proteins rely on ionizable groups to maintain their structure in aqueous environments. The formation of ion pairs affects the overall charge and stability of these macromolecules, influencing how they interact with other biomolecules.
The binding interactions between charged amino acids in proteins can lead to the formation of ion pairs, which help stabilize the protein's conformation. Additionally, ion pairs influence the transport of ions and molecules across cell membranes. Specifically, ion channels utilize ion pairs to facilitate selective ion movement. The precise control of these movements is essential for cellular communication and homeostasis.
In Analytical Chemistry
Ion pairs have significant relevance in analytical chemistry, primarily in methods such as ion chromatography and mass spectrometry. In ion chromatography, the interaction between ion pairs enhances the separation of different ions in solution, making it feasible to analyze complex mixtures. The use of ion-pairing agents, such as tetrabutylammonium ions, helps to improve detection limits and resolution.
Furthermore, ion pairs can influence the ionization efficiency in mass spectrometry. The presence of specific ion pairs affects the fragmentation patterns of the analytes. This characteristic is utilized to enhance the sensitivity and specificity of mass spectrometric analyses. Understanding the behavior of ion pairs in these methods allows for improved methodologies and more accurate results.
In Material Science
Within material science, ion pairs contribute to developing new materials with tailored properties. For example, the design of conductive polymers often involves the formation of ion pairs to enhance conductivity. Polymers with ion-conducting capabilities are utilized in energy storage devices, such as lithium batteries. Here, ion pairs facilitate the movement of lithium ions, enhancing the overall performance of the battery.
Moreover, ion pairs are instrumental in the formulation of nanomaterials. By controlling the ionic interactions, researchers can fine-tune the physical and chemical properties of nanoparticles. This manipulation allows for the creation of responsive materials that can adapt to external stimuli, such as changes in pH or temperature.
In summary, the applications of ion pairs extend across diverse disciplines, underscoring their importance in advancing both theoretical knowledge and practical technologies. Their roles in facilitating biological functions, enhancing analytical techniques, and driving innovations in materials underscore the potency of this concept.
Ion Pairs and Electrochemistry
Ion pairs play a crucial role in electrochemistry, significantly influencing the properties and behaviors of electrolytes. Understanding the dynamics of ion pairs in this context provides valuable insights into various processes, from conductivity to energy storage. In electrochemical systems, the interaction of ions is fundamental for performance and efficiency.
Conductivity Studies
Conductivity studies investigate how well a solution can conduct electric current. This property depends largely on the presence of charged particles, such as ions in a solution. Ion pairs influence conductivity by affecting the overall mobility of ions. When ion pairs form, they can either enhance or reduce conductivity depending on their nature and concentrations.
In research, studies often utilize specialized equipment to measure conductivity across different concentrations and temperatures. Key findings typically indicate that a higher degree of ion pairing can lead to a decrease in conductivity. This decrease happens because ion pairs create neutral entities that do not contribute to current flow. Consequently, understanding ion pairing is vital for optimizing solutions, especially in batteries and supercapacitors.
Additionally, ion concentrations play an integral role. The critical balance between free ions and ion pairs determines the conductivity level within a specific electrochemical system. Adjustments in temperature can also alter the extent of ion pairing, impacting the conductivity.
The relationship between ion pairing and conductivity is a central focus in electrochemical studies, revealing underlying mechanisms that govern performance in various applications.
Ion Mobility
Ion mobility refers to the speed at which an ion can move under an electric field. This concept is closely related to ion pairs since the formation of these pairs affects how freely individual ions can migrate. When ion pairs form, their effective size increases, subsequently reducing their mobility. In cases where ion pairs are tightly bound, the overall mobility can be significantly hindered.
In many electrochemical applications, such as fuel cells, high ion mobility is desirable for optimal performance. Researchers explore various strategies to enhance ion mobility, including the use of additives that can disrupt ion pairing and increase the number of free ions. Moreover, knowing the mobility of different ion species can assist in tailoring materials for specific electrochemical applications, facilitating better design of batteries and sensors.
Ion mobility studies often employ techniques such as time-of-flight mass spectrometry or conductance measurements to evaluate how ions behave in different environments. The methodologies provide valuable data that can inform the development of advanced materials designed to improve overall electrochemical efficiency.
In summary, ion pairs play an essential role in electrochemistry. Conductivity studies and ion mobility measurements contribute significantly to understanding the impact of ion pairing on various electrochemical processes. This knowledge is crucial for enhancing the performance of electrochemical devices and refining strategies in energy storage and conversion.
Experimental Techniques for Studying Ion Pairs
Understanding ion pairs requires robust experimental techniques. Such methods are crucial to identify, characterize, and analyze the behavior of these entities in different environments. Ion pairs influence various chemical and physical processes, making their study vital in both research and applied science. In this section, we delve into three primary experimental techniques used for studying ion pairs: spectroscopic methods, electrochemical methods, and computational approaches. Each technique offers unique benefits and considerations that contribute to a comprehensive understanding of ion pairs.
Spectroscopic Methods
Spectroscopic methods provide insights into the structural and electronic properties of ion pairs. Techniques such as UV-Vis spectroscopy and NMR spectroscopy are commonly utilized.
- UV-Vis Spectroscopy: This method analyzes the absorption of light by ions at different wavelengths. It helps in identifying ion pairs based on their absorbance peaks. Changes in peak intensities can indicate the formation or dissociation of ion pairs.
- NMR Spectroscopy: Nuclear Magnetic Resonance is invaluable for observing the environment of specific nuclei in ion pairs. By analyzing the chemical shifts, researchers can deduce interactions between ions and hence infer the presence of ion pairs.
These spectroscopic approaches allow scientists to determine ion pair dynamics in solution, giving crucial insights into the mechanisms of chemical reactions that involve these species. The data obtained can be critical when assessing how ion pairs behave in various solvents.
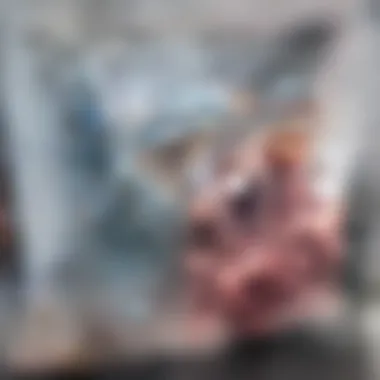
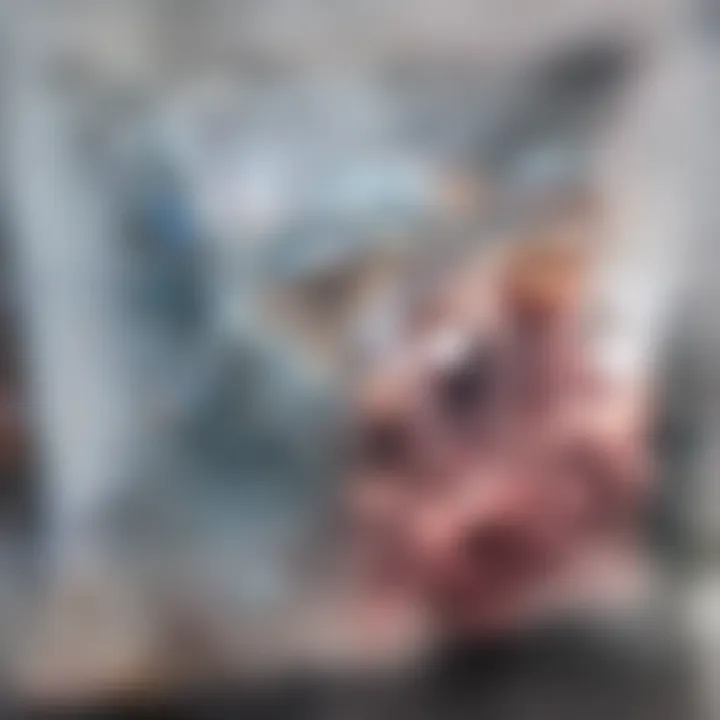
Electrochemical Methods
Electrochemical methods are essential for studying the behavior of ion pairs at the electrode-solution interface. Techniques like cyclic voltammetry and impedance spectroscopy are often employed.
- Cyclic Voltammetry: This technique measures the current response of electrochemical systems as the potential is varied. It can reveal information about the kinetics of ion pair formation and their stability in solutions. Peaks in the current response suggest either the formation or disruption of ion pairs during the redox processes.
- Impedance Spectroscopy: This method provides information on the charge transfer and diffusion properties of ions and ion pairs in solutions. By analyzing the impedance spectra, researchers can infer details about the ion pairing phenomenon, including relaxation times and equilibrium constants.
Electrochemical techniques are particularly potent in understanding ion pairs' roles in various electrochemical applications, such as batteries and fuel cells.
Computational Approaches
Computational approaches have become increasingly significant in studying ion pairs due to their ability to simulate complex molecular behaviors. Techniques often used include molecular dynamics simulations and quantum mechanical calculations.
- Molecular Dynamics Simulations: These simulations allow researchers to visualize the motion and interaction of ion pairs over time. Adjustments in temperature and concentration can be manipulated to observe how these factors affect ion pair stability and dynamics.
- Quantum Mechanical Calculations: These calculations can predict interaction energies and geometries of ion pairs. They are particularly useful in examining ion pair stability under various conditions, including different solvent environments.
Computational methods complement experimental data, providing insights that might not be attainable through experimental techniques alone. They enhance the understanding of ion pair phenomena at a fundamental level.
The combination of spectroscopic, electrochemical, and computational methods creates a holistic approach to studying ion pairs, enabling researchers to explore their roles in both fundamental science and practical applications.
Challenges in Ion Pair Research
Research on ion pairs is crucial for understanding many fundamental chemical and physical processes. However, this area presents several challenges that need careful consideration. As the study of ion pairs evolves, scientists encounter barriers related to detection sensitivity and modeling complexity. Addressing these issues not only improves our understanding of ion pairs but also enhances their application in various fields.
Detection Sensitivity
One of the primary hurdles in ion pair research is the challenge of detection sensitivity. Detecting ion pairs requires advanced methods capable of discriminating between various species present in a sample. Standard detection methods, like mass spectrometry, may lack the sensitivity required for identifying weak interactions associated with transient ion pairs.
Improving detection methods can involve refining instrumentation or employing more sensitive techniques. For instance, specialized NMR techniques enable the observation of dilute ion pairs in complex mixtures. By enhancing detection sensitivity, researchers can gain deeper insights into the dynamics and behavior of ion pairs in a solution.
Modeling Complexity
The complexity of modeling ion pairs also poses a considerable challenge. Ion pair behavior arises from intricate interactions between charged ions and surrounding solvent molecules. These interactions can differ significantly based on the specific conditions, such as temperature, pressure, and concentration. Consequently, creating accurate models can be difficult.
Researchers must balance computational efficiency with accuracy. Some modeling approaches may oversimplify the interactions, leading to misleading results. Others may provide detailed insight, but at the cost of computational resources and time. Striking the right balance is essential to developing reliable models that can predict the behavior of ion pairs under varying conditions.
In summary, the challenges in ion pair research, particularly detection sensitivity and modeling complexity, necessitate ongoing innovation in methods and approaches. Addressing these challenges is essential for advancing our knowledge of ion pairs and their implications in chemistry and physics.
Future Directions in Ion Pair Studies
The exploration of ion pairs is framing the future of not only theoretical chemistry but also practical applications across various fields. As understanding improves, ion pairs are becoming central to advancements in material science, biochemistry, and even environmental chemistry. Increased interest in this area signals significant implications for research methodologies and application processes. This section highlights upcoming trends and focuses on key areas that may shape future investigations into ion pairs.
Advancements in Research Technology
Technological innovations are paving new pathways in ion pair research. The development of more sensitive and precise measurement techniques allows for the exploration of ion pair dynamics under various conditions. Methods like high-resolution mass spectrometry and advanced spectroscopic techniques are proving invaluable. They enable researchers to observe ion pair behaviors at an unprecedented scale and detail. Moreover, imaging technologies provide real-time observations of ion interactions in complex systems.
Emerging technologies like cryo-electron microscopy can observe ion pairs in native environments, elevating our understanding significantly.
Furthermore, machine learning algorithms are now being applied to analyze large datasets generated from these experiments. These approaches uncover patterns and correlations that may have been previously overlooked. As a result, the implementation of artificial intelligence facilitates the predictive modeling of ion pair behavior in diverse environments, enhancing experimental efficiency and accuracy.
Interdisciplinary Approaches
The investigation of ion pairs frequently requires contributions from multiple scientific disciplines. By combining insights from chemistry, physics, biology, and materials science, researchers can gain a more holistic view of ion pair behavior.
For instance, collaborations between chemists and biologists can reveal how ion pairs function within biological systems, including their roles in enzyme catalysis and cellular communication. Similarly, physicists contribute their expertise to understand the fundamental forces driving ion pair interactions.
Moreover, interdisciplinary education programs are emerging, encouraging students to engage in multidisciplinary studies. These programs are essential to producing a new generation of scientists adept in various fields, which is crucial in tackling complex challenges associated with ion pairs.
As researchers adopt a more integrated approach, they enhance their capacity to solve intricate problems, ultimately driving innovation. Understanding ion pairs demands not only a firm grasp of individual disciplines but also the ability to synthesize this knowledge effectively. Thus, embracing these interdisciplinary strategies will be vital for advancing ion pair studies in the future.
Ending
In examining the role of ion pairs, it becomes clear that they are fundamental to a nuanced understanding of both chemistry and physics. Their significance extends beyond theoretical interest; they have practical implications in various areas including biological systems, analytical chemistry, and electrochemistry.
Summary of Key Points
- Definition and Formation: Ion pairs consist of oppositely charged ions that associate due to electrostatic forces. Factors such as temperature and solvation effects play important roles in their formation.
- Classification: Ion pairs can be categorized into contact, solvent-separated, and triple ion pairs, each exhibiting unique characteristics that influence their behavior in different environments.
- Chemical Reactions: Their presence can greatly impact reaction mechanisms and kinetics, thus altering the pathways and rates at which chemical reactions occur.
- Research Techniques: Innovations in spectroscopy, electrochemistry, and computational modeling have enhanced our ability to study ion pairs more effectively.
Implications for Future Research
The exploration of ion pairs holds promise for numerous scientific advancements. As researchers develop more refined techniques for detection and analysis, the understanding of ion pair dynamics will deepen. For instance, the interplay of ion pairs within biological systems could provide insights into cellular processes and the design of novel therapeutics. Furthermore, interdisciplinary collaborations, merging insights from chemistry, biology, and materials science, are poised to unveil new applications of ion pairs.
In summary, continuous investigation into ion pairs will not only enrich the foundational knowledge of chemical interactions but also catalyze innovations across multiple domains. A thorough understanding of these entities may pave the way for transformative applications and technologies.