Lithium Batteries: Pioneering Scalable Energy Solutions
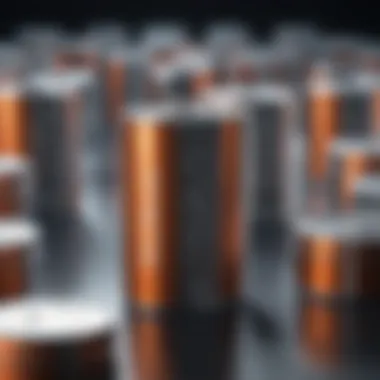
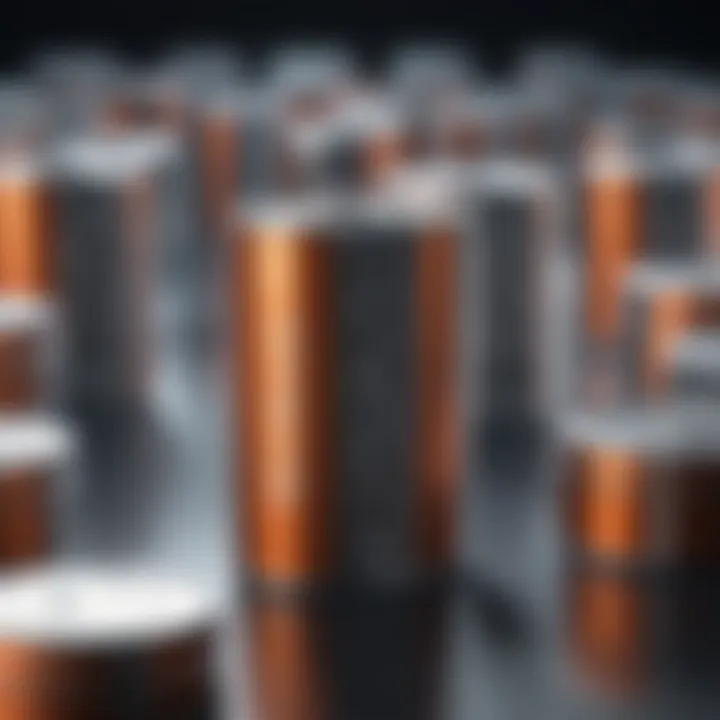
Intro
Lithium batteries have emerged as critical components in the pursuit of scalable energy solutions. Their ability to store and discharge energy efficiently makes them well-suited for various applications. This includes electric vehicles, renewable energy integrations, and consumer electronics. Understanding the nuances of lithium battery technology is essential for grasping its role in the modern energy landscape. The implications are far-reaching, influencing environmental sustainability and economic development.
Significant advancements have been made in lithium battery technology. Researchers are constantly exploring enhanced materials and technologies to improve their performance and reduce environmental impact. However, scaling production presents challenges that cannot be overlooked. From raw material sourcing to manufacturing processes, each step demands careful consideration and innovation.
Sustainability is a central theme in this exploration. As demand for lithium batteries grows, it becomes crucial to examine the lifecycle of these products, including end-of-life disposal and recycling options. Not only does this entail understanding the materials used, but it also involves assessing the ecological implications of lithium extraction and battery production.
This article aims to provide a comprehensive understanding of lithium batteries within the context of scalable energy solutions. It will unfold as a detailed narrative incorporating key findings, methodologies, and discussions relating to the interplay between technology and sustainability.
Prolusion to Lithium Batteries
The significance of lithium batteries has seen a dramatic rise in recent years. As essential components in modern technology, they play a pivotal role in various sectors such as consumer electronics, electric vehicles, and renewable energy solutions. Understanding lithium batteries is crucial for students, researchers, educators, and professionals, as the demand for scalable, efficient energy storage continues to grow.
Definition and Composition
Lithium batteries are a type of rechargeable battery that use lithium ions as a primary component of their electrochemistry. They consist mainly of a lithium compound for the cathode, usually lithium cobalt oxide or lithium iron phosphate, and a graphitic carbon material for the anode.
The electrolyte in lithium batteries is typically a lithium salt dissolved in an organic solvent. This design allows for a high energy density, making lithium batteries favorable compared to alternatives like nickel-cadmium or lead-acid batteries. The ability to charge quickly, combined with a significant cycle life, also adds to their appeal in applications requiring substantial energy management.
Historical Context
The journey of lithium batteries began in the mid-20th century, with the first practical lithium battery introduced in 1991 by Sony. Before this, the use of lithium was limited due to safety concerns linked to its reactivity. Early advancements focused primarily on improving battery safety and efficiency, paving the way for the technology we know today.
Since their inception, lithium batteries have experienced rapid advancements. The mobile revolution, characterized by the surge in smartphone usage, significantly increased demand for more efficient power sources. This demand further propelled innovations in lithium battery technology. Over the years, various chemistries and architectures have emerged, contributing to the multifaceted applications of lithium batteries in the modern world.
"Lithium-ion batteries were a breakthrough technology that enabled the compact design and functionality of portable electronic devices."
The growing focus on electric vehicles and renewable energy systems marks the next chapter in the evolution of lithium batteries. Their adaptability and efficient energy storage capabilities make them ideal choices for supporting a sustainable future in transportation and grid management. As researchers continue to develop better materials and manufacturing techniques, we can expect lithium batteries to dominate the energy landscape for years to come.
The Chemistry Behind Lithium Batteries
The topic of chemistry in lithium batteries is fundamental. Understanding the chemical processes and materials involved enables deeper insights into the performance and efficiency of these batteries. Chemistry governs energy density, charging rates, and cycle life, impacting various applications like electric vehicles and renewable energy storage. Knowledge of the core components initiates a dialogue about innovations and enhancements that can improve lithium battery performance and sustainability.
Basic Chemical Reactions
The basic chemical reactions in lithium batteries take place during the charge and discharge cycles. At the heart of these reactions is the movement of lithium ions between the anode and cathode.
When charging, lithium ions move from the cathode (often made of lithium cobalt oxide) through the electrolyte into the anode (typically graphite). The equation for the reaction is as follows:
This reaction shows that lithium ions are intercalated into the anode material. During discharge, the opposite occurs. Lithium ions travel back to the cathode, delivering energy that powers devices.
The efficiency of these reactions directly relates to the conductivity of the materials used and the design of the battery itself. Higher conductivity in the electrolyte often translates to better performance and faster charging times.
Electrode Materials
The electrode materials play a critical role in determining the battery's overall efficiency, capacity, and lifespan. Different materials offer unique benefits and considerations, directly affecting how the batteries perform under different conditions.
Anode Materials
Anode materials are primarily responsible for storing lithium ions during charging. Graphite is the traditional choice for anodes due to its high capacity and stability. One key characteristic of graphite is its layered structure, allowing efficient lithium ion intercalation. This makes graphite a popular choice as it provides:
- High energy density
- Good cycle stability
However, graphite does have limitations. It has a theoretical capacity of about 372 mAh/g, which might not suffice for applications needing higher energy density. Thus, researchers are exploring silicon and lithium metal as alternatives, which can significantly increase capacity. But these options come with their challenges, such as expansion during cycles, which can lead to reduced lifespan.
Cathode Materials
Cathode materials determine much of the battery's voltage and overall energy capacity. Lithium cobalt oxide is a dominant choice for many consumer electronics. Its key characteristic is a high specific energy, which allows devices to run longer on a single charge. Benefits include:
- High energy density
- Relatively stable structure during cycling
On the downside, lithium cobalt oxide presents an issue with thermal stability and resource scarcity, which raises environmental concerns. Alternatives like lithium iron phosphate may offer lower energy density, but they deliver greater thermal stability and longer cycle life. Thus, the choice of cathode material is a balance between energy capacity and safety concerns.
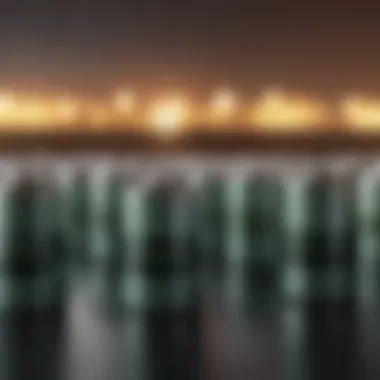
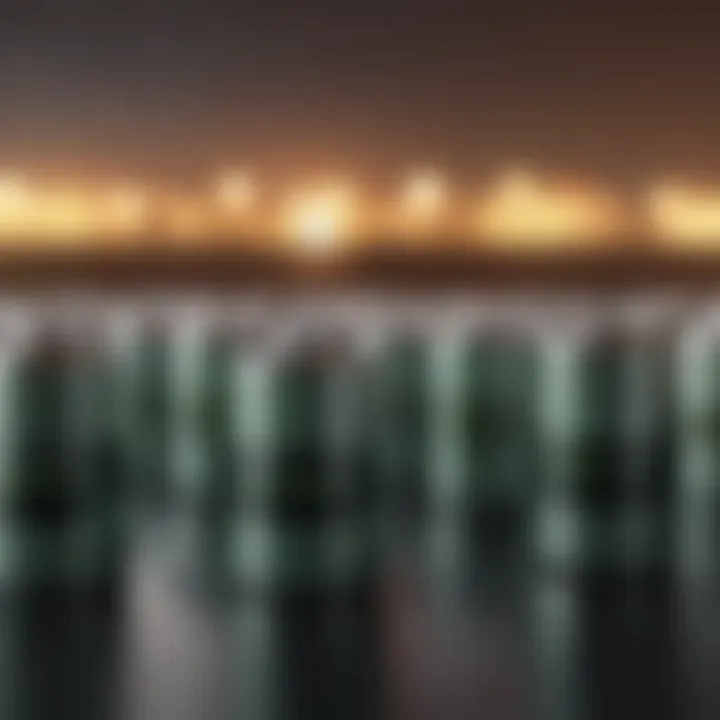
Electrolyte Technologies
Electrolytes are vital as they facilitate the movement of lithium ions between the electrodes. Traditionally, liquid organic electrolytes have been used, providing good ionic conductivity. However, they also come with risks like flammability. Innovations such as solid-state electrolytes are gaining traction due to their potential benefits:
- Enhanced safety and stability
- Higher energy densities
This area remains active in research and development. New materials are continuously being evaluated for possible integration into commercial lithium-based battery systems, focusing on enhancing performance while minimizing safety risks.
Effective chemistry in lithium batteries is crucial for advancing technology and meeting growing energy demands.
Applications of Lithium Batteries
The application of lithium batteries is crucial to understanding their role in modern technology and energy systems. Lithium batteries are central to numerous fields, ranging from consumer electronics to transportation and renewable energy storage. Each application presents unique benefits and challenges that can influence the overall effectiveness and sustainability of lithium battery technology in addressing contemporary energy demands.
Consumer Electronics
Smartphones
Smartphones represent one of the most significant sectors for lithium battery application. These devices depend on high-energy density batteries that maintain lightweight properties while offering long-lasting performance. A key characteristic of lithium-ion batteries in smartphones is their ability to recharge quickly, which aligns well with consumer expectations for rapid functionality.
Moreover, lithium batteries have a high cycle life, meaning they can endure numerous charge and discharge cycles without substantial degradation. However, one challenge is heat generation during charging, which can affect battery lifespan. Despite this, the mobility and compact design of smartphones powered by lithium batteries continue to make them a popular choice for users and manufacturers alike.
Laptops
Laptops are another critical arena for lithium battery use. As portable computing devices, laptops require batteries that can deliver substantial power while remaining efficient. The major advantage of lithium batteries in laptops is their capacity to provide power over extended periods without the need for frequent recharges. This is particularly beneficial for professionals who rely on their devices for long meetings or travel.
A unique feature of lithium batteries used in laptops is their integrated management systems, which help optimize performance and safety. However, these batteries can sometimes be more expensive compared to other alternatives, which can limit access for some users. Nonetheless, the performance and reliability of lithium batteries solidify their importance in this sector.
Electric Vehicles
Electric vehicles (EVs) are transforming the landscape of transportation. Lithium batteries are key to this evolution due to their capacity for high energy density and relatively low weight. This enables longer driving ranges and quicker acceleration, enhancing the appeal of electric vehicles in a market predominantly characterized by gasoline-powered cars.
However, there are challenges in scaling battery production to meet increasing EV demands. Issues related to sourcing lithium, cobalt, and other materials can create supply chain constraints. These challenges must be addressed to foster wider adoption of electric vehicles.
Renewable Energy Storage
Solar Energy Systems
Solar energy systems often use lithium batteries to store energy produced during the day for use at night or during cloudy periods. The capacity for quick recharging and long cycle life makes these batteries well-suited for integration in solar energy solutions. A critical characteristic here is efficiency; lithium batteries can convert and store energy with little loss.
Nevertheless, one must consider the costs associated with battery installation and maintenance, which can pose barriers to broader usage in some regions. Despite these factors, their ability to support renewable energy systems presents significant advantages for sustainable energy transitions.
Wind Energy Applications
Wind energy applications also benefit from lithium battery technology. These systems require reliable storage solutions to provide consistent power, given the variable nature of wind. Lithium batteries can support this variability effectively due to their capacity for rapid charge and discharge.
The key characteristic of lithium battery systems in wind applications is their compactness, enabling integration in various settings from large wind farms to smaller installations. However, like solar systems, they face challenges related to cost and environmental concerns tied to battery production and disposal. Still, they remain a valuable part of the renewable energy mix due to their efficiency and effectiveness.
Industrial Uses
In industrial contexts, lithium batteries are increasingly utilized for a variety of applications, including fork-lifts and robotics. The ability to provide consistent power with minimal downtime is particularly advantageous in manufacturing and logistics. Lithium batteries can enable more efficient operations by reducing maintenance needs and extending operational capacity.
Overall, the applications of lithium batteries span numerous sectors with unique considerations. Their high energy density and efficiency make them pivotal in a world striving for sustainable energy solutions, yet there are challenges requiring ongoing innovation and resource management.
Scaling Production of Lithium Batteries
The topic of scaling production of lithium batteries holds significant importance in meeting the growing demand for energy storage solutions. As global reliance on renewable energy sources and electric vehicles increases, the necessity to produce lithium batteries at a larger scale becomes crucial. The interplay between production capabilities and consumer needs shapes the future of technology in various sectors. Financial feasibility, environmental impacts, and efficiency top the list of considerations when addressing this subject.
Current Manufacturing Processes
Traditionally, producing lithium batteries involves several steps that include sourcing raw materials, electrode manufacturing, and cell assembly. The classic methods have relied heavily on manual labor and batch production techniques. This approach presents challenges, such as inconsistent quality and time-consuming processes.
In the present industry climate, companies are striving toward vertical integration of manufacturing. This means controlling every aspect, from mining lithium to assembling the final product. Companies such as Tesla and Panasonic have initiated large-scale facilities to boost output. These operations emphasize efficiency while maintaining sustainability principles. The challenge remains to reduce energy consumption and optimize material usage throughout the production line to ensure minimal waste and maximum product life.
Technological Innovations for Scaling
Emerging technologies have the potential to revolutionize the way lithium batteries are produced. Innovations focus on automation and efficient material sourcing. These advancements can significantly alter the production landscape.
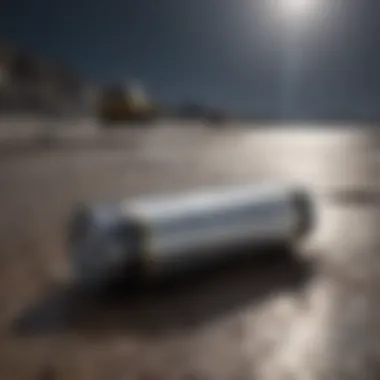
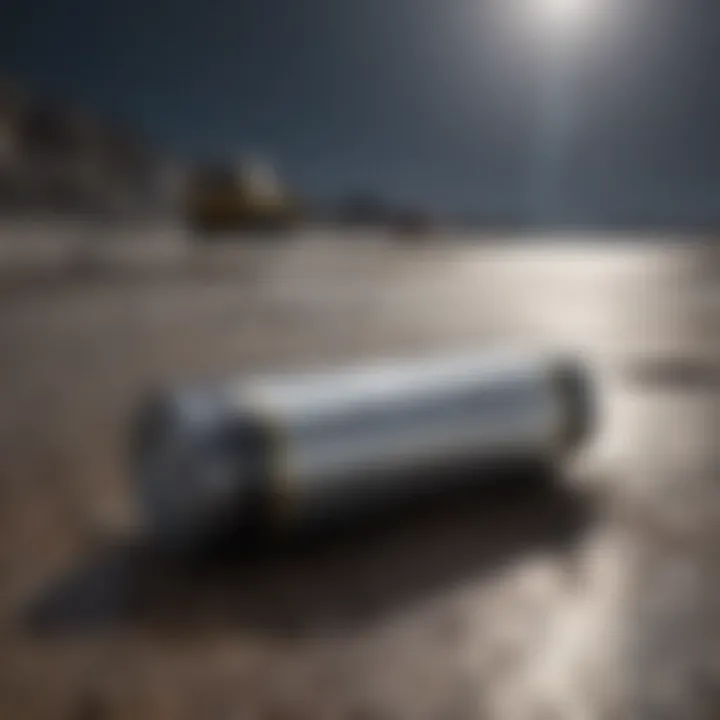
Automation
Automation plays a critical role in enhancing production capabilities for lithium batteries. By integrating robotic systems and automated processes, manufacturers can achieve higher throughput and efficiency. One of the key characteristics of automation is its ability to minimize human error during production. This quality makes it a popular choice in the battery manufacturing ecosystem. The unique feature of automation is its scalability; manufacturers can adjust production levels quickly based on market demands.
The advantages include reduced labor costs and improved consistency in product quality. However, some disadvantages exist as well. Dependence on technology can lead to concerns over job losses in the manufacturing sector, and initial setup costs can be high for fully automated systems.
Material Sourcing
Material sourcing offers essential insights into the scalability of lithium batteries. This aspect involves obtaining materials necessary for battery production, such as lithium carbonate, cobalt, and nickel. A significant characteristic of efficient material sourcing is the focus on sustainable practices. This makes it a beneficial choice for companies seeking to enhance their environmental footprint while maintaining production capabilities.
The unique feature of advanced material sourcing techniques is the development of partnerships with mining companies that prioritize ethical and sustainable practices. The advantages include more stable supply chains and reduced environmental impact. However, the downside may involve fluctuating raw material prices and potential geopolitical risks in sourcing locations.
"As we move forward, the intersection of technology and sustainable practices in lithium battery production will be critical for meeting global energy needs effectively."
Through a careful analysis of current manufacturing processes and the innovations shaping the future, lithium battery production stands at a pivotal point. By adopting automation and refining material sourcing, industries can enhance their capacity to meet increasing demands while striving for sustainability.
Challenges in Lithium Battery Production
The production of lithium batteries, while a crucial aspect of modern energy solutions, is fraught with complexities. Addressing these challenges is essential to optimize lithium battery technology and ensure its sustainable scalability across various applications. This section will explore significant impediments in the supply chain, environmental concerns, and technological limitations associated with lithium battery production.
Supply Chain Constraints
Supply chain constraints present a substantial barrier to the efficient production of lithium batteries. Various elements affect the consistency and reliability of the materials needed for battery manufacturing. Disruptions caused by geopolitical tensions or natural disasters can lead to significant delays or shortages of critical components such as lithium, cobalt, and graphite.
- Source Quality: The quality of materials directly impacts battery performance. Ensuring a steady supply of high-grade raw materials is vital for meeting rising energy demands.
- Cost Fluctuations: Price volatility in raw material sourcing can reduce profit margins for manufacturers. Companies must navigate these fluctuations effectively to maintain economic viability.
In summary, the complexities in the supply chain can hinder advancements in lithium battery production, potentially impacting the broader adoption of this technology.
Environmental Concerns
Environmental concerns associated with lithium battery production primarily stem from the extraction processes and recycling challenges. The ecological footprint of lithium mining and the need for efficient recycling methods warrant thorough examination to mitigate adverse effects on nature.
Mining Impacts
Mining for lithium can have profound ecological consequences. The extraction process often involves substantial water consumption, which can deplete local water resources and affect surrounding ecosystems.
- Water Usage: Areas rich in lithium deposits may experience significant declines in water availability, impacting agriculture and local communities.
- Landscape Disruption: The physical footprint of mining operations contributes to habitat loss and biodiversity decline.
These characteristics make mining impacts a critical consideration for any discussion about lithium battery production. Understanding these mining practices is essential for developing sustainable solutions in battery production.
Recycling Challenges
Recycling challenges further complicate the path toward sustainability in lithium battery production. The recycling process for lithium-ion batteries is not yet fully developed, resulting in underutilization of valuable materials.
- Technological Limitations in Recovery: Current methods of recovering lithium and other materials from used batteries are not efficient. Many batteries end up in landfills, leading to potential environmental issues when components leach into the soil.
- Economic Viability: Developing a comprehensive infrastructure for battery recycling involves monetary investment and technological advancement.
The unique feature of these recycling challenges lies in their impact on resource recovery and environmental stewardship. Addressing these issues can promote a circular economy in battery production, ultimately benefiting both manufacturers and consumers.
Technological Limitations
Technological limitations also play a significant role in the challenges faced by lithium battery production. The current state of battery technology often falls short in terms of energy density, longevity, and charge times.
- Innovation Stagnation: While there are ongoing research efforts aimed to enhance battery performance, breakthroughs are slow. This stagnation can limit the potential of lithium batteries in meeting future energy needs.
- Integration with Existing Technologies: There can be compatibility issues when integrating new battery technologies into existing systems and applications.
These technological limitations highlight the need for ongoing research and development, making it crucial for stakeholders to remain committed to long-term innovation in lithium battery production.
Sustainability and Recycling of Lithium Batteries
The topic of sustainability and recycling in the context of lithium batteries is vital for addressing the growing demand for scalable energy solutions. As societies increasingly rely on lithium-based technologies for applications ranging from electric vehicles to renewable energy storage, it becomes imperative to evaluate the long-term impacts of these batteries on the environment and resource management. The benefits of focusing on sustainability and recycling include not just mitigating ecological harm, but also promoting responsible consumption and ensuring that valuable materials are recovered for future use.
One key consideration is the finite nature of lithium resources. Lithium, along with cobalt and nickel, is crucial for battery production. As the global appetite for batteries expands, the pressure on these resources increases. Sustainable mining practices can reduce environmental degradation, but recycling presents an opportunity to minimize the extraction of new materials. Recycling also contributes to lowering production costs and reducing emissions associated with mining.
"Recycling lithium batteries not only conserves essential materials but also strengthens the circular economy, making future battery production more sustainable and less resource-dependent."
Life Cycle Analysis
Life cycle analysis (LCA) offers a comprehensive framework for assessing the environmental impacts of lithium batteries from production through disposal. It evaluates every phase, including raw material extraction, manufacturing, use, and end-of-life scenarios. The findings of LCA can influence decisions related to policy, innovation, and consumer behavior.
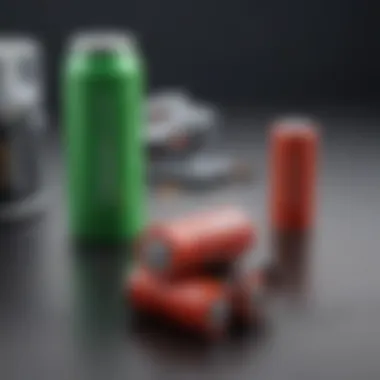
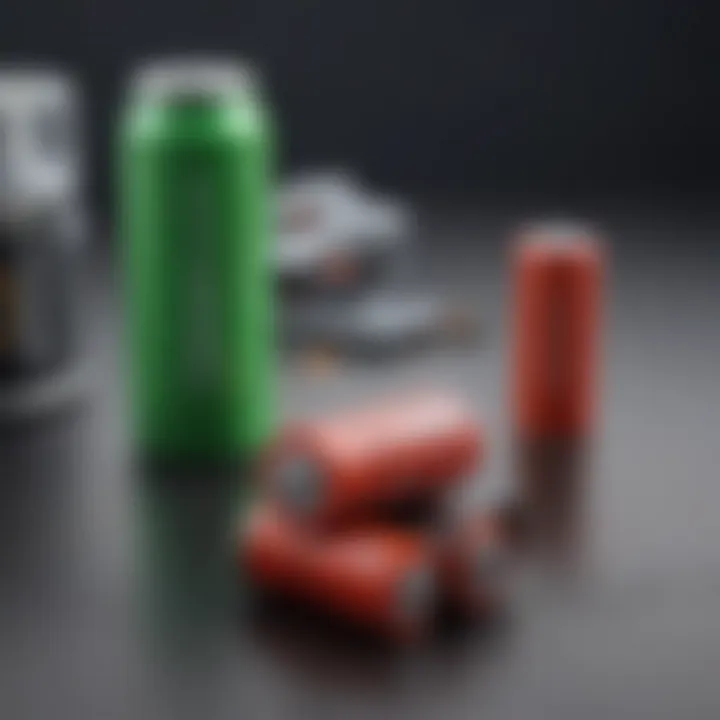
Several factors are examined in an LCA:
- Energy consumption during various manufacturing stages.
- Emissions generated at each phase, particularly during mining and production.
- Potential energy savings and emissions reductions from recycling instead of virgin material extraction.
Considering the full life cycle is essential for informing sustainable practices in both battery manufacturing and usage, ultimately leading to more informed choices by stakeholders.
Current Recycling Methods
Currently, the most common recycling methods for lithium batteries include hydrometallurgical and pyrometallurgical processes. Each method has its own strengths and weaknesses in terms of efficiency and environmental impact.
- Hydrometallurgical recycling involves using aqueous solutions to recover metals from battery materials. It allows for the separation of valuable materials like lithium, cobalt, and nickel with relatively low energy requirements.
- Pyrometallurgical recycling uses high temperatures to melt battery components and recover metals. While this method can be effective at recovering metals, it requires significant energy input which can diminish sustainability gains.
Both methods are operational but face challenges such as economic viability and the need for advanced technology to ensure safety and efficiency.
Future Developments in Recycling Technology
Future developments in recycling technology for lithium batteries hold promise for improving efficiency and reducing environmental impact. Innovations are emerging, including:
- Direct recycling techniques that preserve the batteryβs original structure, potentially enhancing recovery rates of lithium and other materials.
- Microbial processes utilizing specially designed bacteria to leach metals from battery waste, providing an environmentally friendly alternative to traditional methods.
- Improved battery designs themselves focusing on ease of disassembly. This can simplify recycling processes and allow for better material recovery.
Continuous research and investment into these technologies could lead to more effective recycling strategies, strengthening the sustainability framework for lithium batteries and ensuring that they remain a responsible choice as energy solutions evolve in future.
The Future of Lithium Batteries
The future of lithium batteries is promising yet complex. As technologies evolve, so do the demands and expectations from energy storage systems. Lithium batteries are central to numerous innovations in energy-based applications. Their role in consumer electronics, electric vehicles, and renewable energy solutions underscores their importance. Understanding future developments allows us to anticipate the shifts in technology and policy. This section explores emerging technologies, policy implications, and market trends that will shape the future of lithium batteries.
Emerging Technologies
Innovations in lithium battery technology continue to emerge. Currently, research focuses on enhancing energy density and reducing charging times. Solid-state batteries represent a significant technological shift. They promise greater safety and efficiency by replacing liquid electrolytes with solid materials. This transition could lead to batteries that last longer and pose fewer risks of leakage.
Developments in lithium-sulfur and lithium-air technologies are also underway. These alternatives could potentially offer higher energy capacities than conventional lithium-ion batteries. Companies like Sion Power and 24M are making strides in these areas. Additionally, advancements in manufacturing processes can reduce costs and improve scalability.
"Emerging technologies in lithium batteries will redefine energy storage capabilities and pave the way for new applications."
Policy Implications
As the demand for lithium batteries expands, so do regulatory challenges. Governments are increasingly recognizing the environmental impact of lithium mining and battery disposal. Policies surrounding recycling and sustainable sourcing are necessary. International cooperation becomes vital to ensure that raw materials are sourced responsibly. These policies not only guide production practices but also influence public acceptance of lithium technology.
Legislation may focus on incentivizing research into alternative battery technologies or improving the lifecycle management of existing batteries. Furthermore, establishing standards can help regulate the quality and safety of lithium battery production, ensuring reliability in end-use applications.
Market Trends
Market trends reveal an upward trajectory for lithium batteries, driven by consumer demand and technological advancements. The growth of electric vehicles is a major factor influencing this demand. Major automakers are investing significantly to electrify their fleets, increasing the need for efficient battery solutions. This rush is propelling innovation and scalability.
Moreover, the rise of renewable energy systems requires reliable energy storage solutions. Lithium batteries are crucial in linking intermittent sources like solar and wind energy to end-user demands.
Financial analysts predict a continuous decline in battery costs as production scales. Companies like Tesla and Panasonic are ramping up battery production capacity, which is expected to reduce prices even further.
In summary, the future of lithium batteries will be characterized by technological evolution, evolving regulations, and market expansion. These elements will interact to reshape the landscape of energy storage solutions, paving the way for more sustainable and efficient systems.
Finale
The conclusion of this article serves as a vital synthesis of the information discussed throughout the various sections. It emphasizes the importance of lithium batteries in facilitating advanced energy solutions across multiple sectors. Their role is not just limited to consumer electronics or electric vehicles; lithium batteries influence the broader landscape of renewable energy, industrial applications, and eventually societal norms surrounding energy consumption.
One key element raised in this exploration is the scalability of lithium battery technology. As demand increases, so does the need for streamlined production processes, and advancements in materials and manufacturing techniques can bring solutions to market effectively. This scalability is crucial for transitioning from traditional energy sources to more sustainable alternatives.
Additionally, the environmental impacts associated with lithium battery production and disposal are critical considerations. The article highlights recycling as a significant component in ensuring sustainability. Implementing efficient recycling methods can offset some of the ecological challenges faced by lithium battery production.
Summary of Findings
The exploration of lithium batteries reveals several significant findings:
- Lithium batteries are essential for energy storage, prominently in renewable sectors.
- The scaling of production is hindered by several challenges, including supply chain disruptions and resource scarcity.
- Advancements in technology, such as improved electrode materials, have the potential to enhance battery efficiency and lifespan.
- Environmental concerns require immediate attention, especially regarding mining practices and recycling methods.
- Future research must focus on sustainable practices and innovative technologies to enhance the life cycle of lithium batteries.
"The success of lithium batteries will not only depend on technology but also on responsible production and disposal practices."
Call for Further Research
The domain of lithium batteries is expanding rapidly, creating a compelling case for ongoing research. Future studies should concentrate on the following areas:
- Exploring new materials that could replace lithium in batteries, potentially unlocking higher efficiency and lower environmental impacts.
- Assessing the long-term sustainability of lithium extraction and developing alternative sourcing methods to minimize ecological disruption.
- Investigating advanced recycling technologies that can reclaim materials more effectively and reduce waste.
- Analyzing market trends to predict shifts in demand and how they will influence battery technology evolution.
The study of lithium batteries is ongoing, and as technological and environmental challenges evolve, our understanding will also need to adapt. Engaging with these topics through further research ensures that we can maximize the benefits while minimizing the trade-offs associated with lithium battery use.