Metals Effective in Blocking Radiation: An Analysis
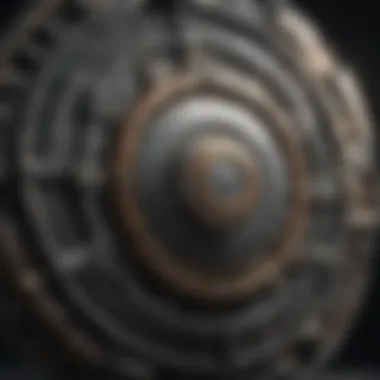
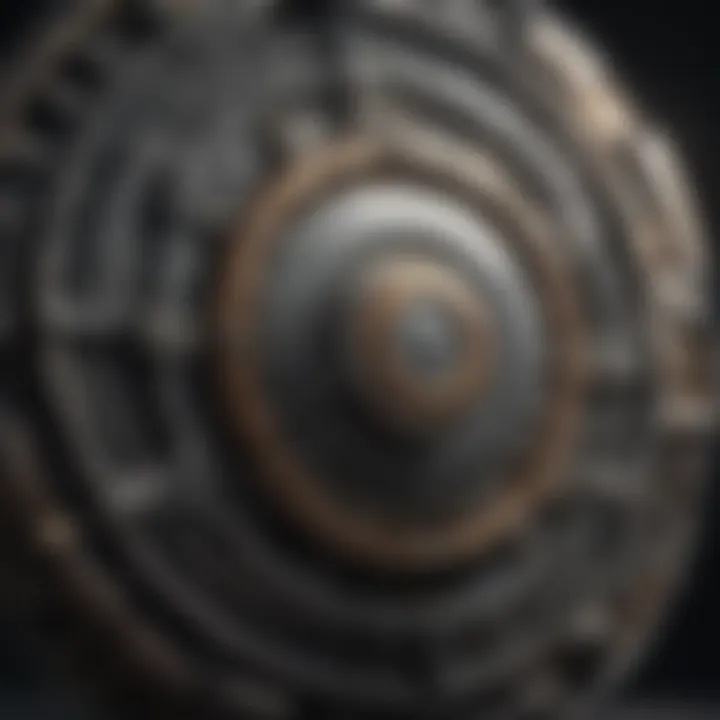
Intro
Radiation is pervasive in our world. From the cosmic rays that reach us from outer space to man-made sources like X-rays and nuclear plants, it’s an omnipresent element of modern life. As intriguing as this fact is, it also raises significant concerns about safety and health. Metal shielding has emerged as a primary line of defense against various types of radiation, offering protection across different industries. This article will explore specific metals known for their effectiveness in blocking radiation, examining their properties, applications, and the physics behind their performance.
Understanding these metals is not just academic; it has real-world implications in fields such as medicine, aerospace, and nuclear energy. The need for effective radiation shielding has become critical, driven by increasing exposure levels in our daily lives. So, what makes specific metals capable of blocking radiation, and why do some work better than others? We will traverse through empirical data and the theoretical framework that supports these findings.
As we dive into this conversation, we’ll dissect the metals that take center stage in radiation protection and gain insights into their relevance in ensuring safety.
Research Overview
Summary of Key Findings
- Lead is one of the most effective materials due to its high density and atomic number, making it a go-to choice for X-ray shielding.
- Tungsten, although heavier,is often preferred in aerospace for its ability to withstand high temperatures while providing robust radiation protection.
- Steel provides a practical balance between cost and effectiveness, commonly used in nuclear facilities.
- Copper also contributes significantly to radiation shielding, notably in electrical applications where attenuating electromagnetic radiation is vital.
Through rigorous analysis, it becomes clear that each metal's unique property plays a pivotal role in its shielding capabilities.
Background and Context
The study of metals and their interaction with radiation is not a recent phenomenon. Long before the age of modern medicine and nuclear technology, researchers recognized the need to shield sensitive populations from harmful radiation. Historical milestones include the development of lead aprons for X-ray patients in the early 20th century and the engineering of steel barricades in nuclear power plants to mitigate exposure to workers.
As technology continues to evolve, the quest for better materials persists. Recent advancements in metallurgy have introduced new alloys and composites that offer enhanced protection without the weight or bulk traditionally associated with radiation shielding.
"The journey of using metals for radiation shielding has seen innovation inspired by both necessity and technological progress."
While the significance of these metals is evident, the science behind how they function remains a wondrous pursuit that connects physics to practical applications.
Methodology
Experimental Design
In exploring the effectiveness of different metals at blocking radiation, structured laboratory methods are employed. For instance, controlled environments mimic real-world conditions, allowing observers to measure how various metals interact with different radiation types -- whether it be ionizing or non-ionizing radiation.
Data Collection Techniques
- Radiation detectors are employed to quantify the levels of radiation before and after passing through metal samples.
- Statistical analysis of collected data helps highlight the efficiency rates of each metal, offering a clear comparison of their shielding capacities.
- Longitudinal studies track the performance of materials over time, ensuring that results remain consistent even in fluctuating conditions.
Prelims to Radiation and Metals
The intersection of radiation and metals forms an interesting and critical field of study, particularly when it comes to protecting human health and the environment. Understanding how different types of metals can effectively block radiation has implications in a variety of settings—from hospitals dealing with diagnostic imaging to aerospace applications where exposure to cosmic radiation can be a concern. This section aims to unravel why this topic is not just for scientists or engineers, but for anyone who is interested in the intersection of technology, health, and safety.
Radiation is a natural phenomenon that permeates our world. Whether it's the radiation we get from the sun or from other man-made sources, it's ever-present. If we were to ignore radiation altogether, we might be taking a gamble with our lives. Metals serve as a first line of defense—acting as shields that can absorb or deflect harmful radiation. Spotlighting the role of metals in radiation shielding is pertinent, as it not only explains the mechanics behind these materials but also addresses considerations of effectiveness, cost, and practical applications.
Understanding Radiation
Radiation comes in various forms, and understanding its nuances is crucial for determining how to block it effectively. Essentially, radiation can be classified into two categories: ionizing and non-ionizing. Ionizing radiation, such as gamma rays and X-rays, has enough energy to knock electrons off atoms, leading to harmful biological effects. On the other hand, non-ionizing radiation, which includes visible light and radio waves, is generally less harmful but can still have certain effects over prolonged exposure.
It's notable that the materials used to shield against these two types of radiation can differ significantly. For example, lead is notably effective against ionizing radiation but is much less useful against non-ionizing forms. The choice of metal for shielding purposes must take into account factors such as the type of radiation encountered, the thickness of the material, and its density.
Understanding this basic concepts sets the stage for a deeper exploration of how specific metals can be used as shields, enabling us to practically apply this knowledge in the aforementioned areas, among others.
The Role of Metals in Radiation Shielding
Metals play a pivotal role in shielding against radiation, primarily due to their density and atomic structure. The denser the material, the more effective it tends to be at blocking radiation. This is why metals such as lead, tungsten, and copper frequently come into play in various shielding applications.
Several factors influence the effectiveness of a metal as a radiation shield:
- Density: Heavier metals are generally better at blocking radiation. For instance, lead is widely used in medical settings because it has a high atomic number and density, effectively attenuating gamma rays.
- Atomic Number: Higher atomic number elements tend to have a greater capacity to absorb photons, making them excellent candidates for radiation shielding.
- Material Composition: The purity and structure of the metal can also greatly influence its effectiveness. Even slight impurities may weaken its ability to block radiation.
In summary, the importance of metals in radiation shielding cannot be overstated. As we continue to explore more about their properties and applications, we not only gain insights into the best practices for protection but also raise awareness of the hidden dangers that radiation can pose.
Types of Radiation
Understanding the types of radiation is fundamental when it comes to discussing how metals can block them effectively. Radiation can be broadly categorized into two main types: ionizing and non-ionizing radiation. Each type has distinct characteristics and interacts with matter in different ways, which can significantly influence how metals are employed for shielding purposes.
Before we dive into each category, it is important to consider why this distinction matters. Ionizing radiation has enough energy to remove tightly bound electrons, thus creating ions. This process can lead to significant biological damage, making it particularly hazardous in environments like nuclear power plants or medical imaging facilities. On the other hand, non-ionizing radiation lacks the energy to displace electrons, thus it is often perceived as less dangerous, although prolonged exposure can still pose health risks.
Ionizing Radiation
Ionizing radiation includes high-energy particles such as alpha particles, beta particles, and gamma rays, as well as X-rays. These forms of radiation are notorious for their ability to penetrate different materials, depending largely on their energies and the atomic structure of the materials encountered.
For instance, gamma rays can penetrating deep into substances. In stark contrast, alpha particles can be stopped by just a sheet of paper, but they can cause serious damage if ingested or inhaled.
Key Points About Ionizing Radiation:
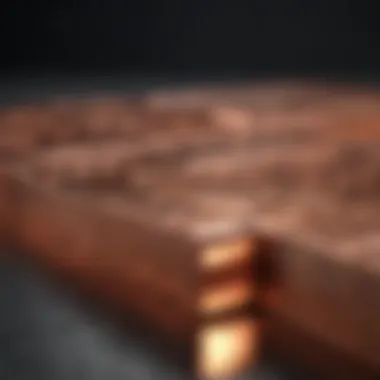
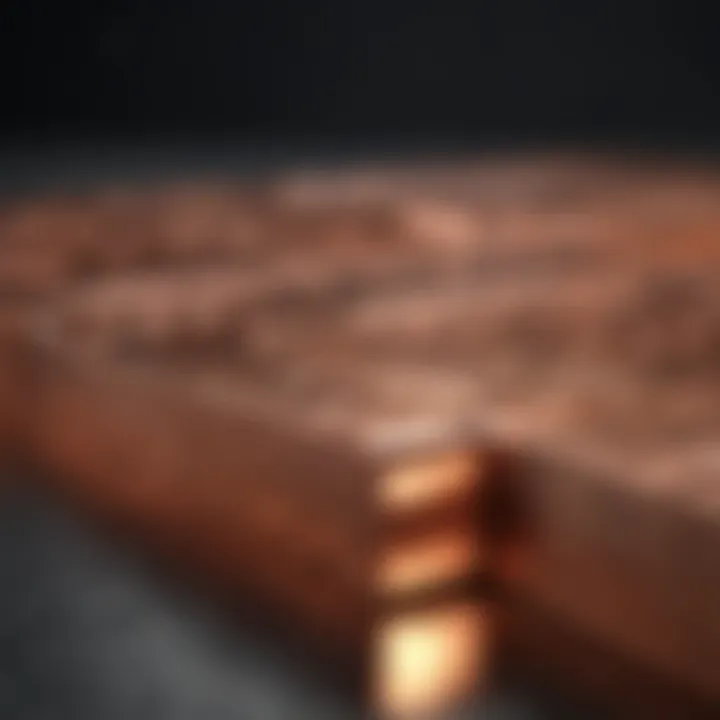
- High Energy: These types can break molecular structures, causing cellular damage.
- Materials Required for Shielding: Dense materials like lead, tungsten, and concrete are particularly effective against these radiations due to their atomic structures.
- Biological Impacts: Prolonged exposure can lead to an increased risk of cancer and other health conditions. That’s why areas using such technologies have strict safety protocols.
Non-Ionizing Radiation
In contrast, we have non-ionizing radiation, which encompasses forms like ultraviolet light, visible light, infrared radiation, and radio waves. These types don’t carry enough energy to ionize atoms but can still have biological effects through thermal and other mechanisms.
For example, UV radiation can cause skin damage, leading to conditions such as sunburn and even skin cancer over time. The shielding against non-ionizing radiation usually involves less dense materials, as there isn’t a need for heavy metals like lead or tungsten.
Considerations for Non-Ionizing Radiation Shielding:
- Common Sources: Everyday sources include sunlight, heat, and radio communication technologies.
- Materials Used: Ordinary glass can filter out some UV radiation, while simple barriers may suffice against microwaves.
- Health and Environment: While generally less dangerous, certain forms of prolonged exposure can still affect health, making adequate shielding important in specific scenarios.
"Understanding the nature of the radiation allows for more effective shielding strategies while ensuring the safety of personnel and the environment."
In summation, recognizing the distinctions between ionizing and non-ionizing radiation provides essential context for selecting the appropriate metals for shielding applications. This foundational knowledge sets the stage for a more focused discussion on the selection of specific metals and their capacities to mitigate these varied radiation threats.
Properties of Metals in Radiation Absorption
Understanding the properties of metals in radiation absorption is crucial for designing effective shielding materials. Metals serve as barriers, absorbing or deflecting different types of radiation, thus minimizing exposure to harmful effects. The effectiveness of these metals hinges on several physical properties, notably density and atomic composition. This section delves into two primary factors: density and atomic number, alongside material composition and structure, providing insight into how these characteristics influence a metal's ability to block radiation.
Density and Atomic Number
When it comes to blocking radiation, density and atomic number play pivotal roles. Density essentially refers to how much mass is contained in a given volume. A denser material is better equipped to absorb energy from radiation, thus reducing the intensity that passes through. For instance, lead, with its high density, has long been the go-to metal in radiation protection. Its compact atomic structure allows it to interact effectively with radiation photons, mitigating their energy.
On the other hand, the atomic number is equally significant. It reflects the number of protons in a metal's nucleus and influences the interaction between the metal and incoming radiation. A higher atomic number generally equates to a greater probability of interaction with energetic photons. For example, tungsten, with a higher atomic number than aluminum, displays superior radiation-blocking capabilities around gamma rays. This combination of density and atomic number forms a foundational understanding of why certain metals are favored in applications requiring radiation shielding.
"The thicker and denser the shield, the better it serves its purpose. Choices like lead and tungsten are no accident—they're chosen based on these very physical properties."
Material Composition and Structure
The material composition and internal structure of metals also merit close examination. Metal alloys, which combine two or more types of metals, can exhibit enhanced properties not found in a single metal. For instance, while pure lead is excellent for blocking x-rays, certain lead-based alloys, when mixed with other metals like tin or antimony, can offer improved mechanical properties while still maintaining effective radiation shielding capabilities.
Moreover, the microstructural characteristics—the arrangement of atoms and defects within the metal—can also influence its radiation-absorbing performance. Crystalline structures that maximize atomic packing will typically offer better shielding mechanisms than those with more disordered arrangements. Specific treatments and heat processing can enhance a metal’s grain structure, further optimizing its ability to absorb radiation.
In summary, the intrinsic properties of metals, especially density, atomic number, material composition, and structure, are essential in determining their efficacy as radiation shields. A thorough grasp of these principles is pivotal not only for selecting appropriate shielding materials but also for innovating new solutions to enhance radiation protection in various industries.
Metals Commonly Used for Radiation Shielding
When it comes to the realm of radiation protection, the right materials can make a world of difference. Metals frequently serve as the frontline defense against various forms of radiation. Their density and atomic structure contribute to their ability to absorb or reflect harmful radiation particles, making them an essential focus in this field. Each metal has its own unique set of characteristics that influence its effectiveness, cost, and applicability in real-world scenarios.
Lead: The Traditional Shield
Lead stands as the time-honored champion of radiation shielding. Its high atomic number means it has a substantial capacity to attenuate radiation. While lead is a heavy metal, this density contributes positively to its shielding effectiveness. For instance, in medical settings, lead aprons are commonly used to protect patients and healthcare professionals from X-ray exposure. The ease of fabrication into sheets or barriers adds to lead's practicality. However, one must consider the health risks associated with lead exposure, especially over prolonged periods. It's a classic case where the benefits must always be weighed against the potential for harm.
Copper: Versatile and Effective
Copper emerges not merely as a conductor of electricity but also as a formidable metal in the realm of radiation shielding. With good malleability, it can be fashioned into a range of useful forms, such as tubes and plates utilized in different scenarios. Its effectiveness in blocking low-energy radiation makes it well-suited for certain medical and industrial applications. Additionally, copper has the benefit of possessing antimicrobial properties, adding another layer of functionality in environments where hygiene is a top priority. Yet, compared to lead, its radiation absorption capacity is lower, which means it often finds itself complementing other materials rather than standing alone.
Tungsten: Robust and Efficient
Tungsten is lauded for its incredible density, almost a match for lead, but with even greater resilience. It’s often used in scenarios requiring durability, such as in aerospace and military applications. For instance, tungsten-based alloys are increasingly popular for radiation shielding in specialized environments. The strength of tungsten allows for thinner shields compared to lead, thereby saving space and weight. One drawback is its high cost and the difficulty in fabrication, but for situations demanding robust performance, tungsten proves its mettle.
Aluminum: Lightweight Shielding
Aluminum often struts its stuff in applications where weight is a critical factor. It’s frequently employed in aerospace applications due to its lightweight nature combined with reasonable shielding efficiency for certain types of radiation. In environments where mobility is key, aluminum can provide a more practical solution. For example, aluminum foil is utilized in some shielding devices, providing a degree of protection without the burden of weight. However, while effective against certain radiations, it may not stand up as well against more energetic particles, necessitating careful selection based on the specific radiation types encountered.
Steel: A Practical Solution
Steel, with its formidable strength and overall versatility, offers a practical approach to radiation shielding. Commonly used in the construction of radiation containment structures, it provides a good balance between cost, weight, and effectiveness against various radiation types. Although its density is lower than lead or tungsten, when applied in sufficient thickness, it can be an effective barrier. Additionally, steel is more readily available and cost-effective, making it a sensible option for many industries. However, its effectiveness can vary based on the radiation energy levels, and engineers often take this into account during the design phase.
Important Note: The selection of metals for radiation shielding depends on factors like the type of radiation, desired weight, and cost considerations. Each metal brings something unique to the table, and understanding these nuances is key to making informed choices.
In summary, the choice of metal for radiation shielding relies on a balancing act between efficiency, risk, and application. By understanding each metal’s properties and appropriate use cases, professionals can ensure better protection against radiation hazards, paving the way for safer medical, industrial, and aerospace environments.
Mechanisms of Radiation Shielding
The mechanisms that enable radiation shielding involve complex interactions between radiation and matter. Understanding these principles is crucial for effectively utilizing metals to block harmful radiation. Different types of radiation engage with materials in distinct ways, each driven by fundamental physics. By exploring these mechanisms, we can better comprehend how certain metals provide superior shielding. Additionally, this knowledge informs safety standards and design choices across various applications, essential in industries like healthcare, aerospace, and nuclear energy.
Photoelectric Effect
The photoelectric effect is a phenomenon where radiation interacts with a metal surface, resulting in the ejection of electrons from that surface. This effect is particularly significant for low-energy X-rays and gamma rays. When radiation hits a metal atom, the energy from the incident photon is absorbed. If this energy exceeds the binding energy of the electrons in the metal, those electrons are released.
Metals with a high atomic number, like lead or tungsten, are especially effective at blocking radiation through this mechanism. The higher the atomic number, the greater the probability the photon will be absorbed rather than pass through. This effect is a critical factor in determining how thick or dense a shielding material needs to be to provide adequate protection against low-energy radiation.
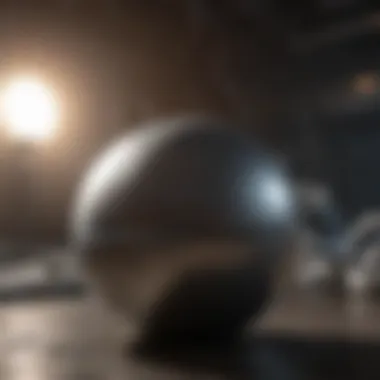
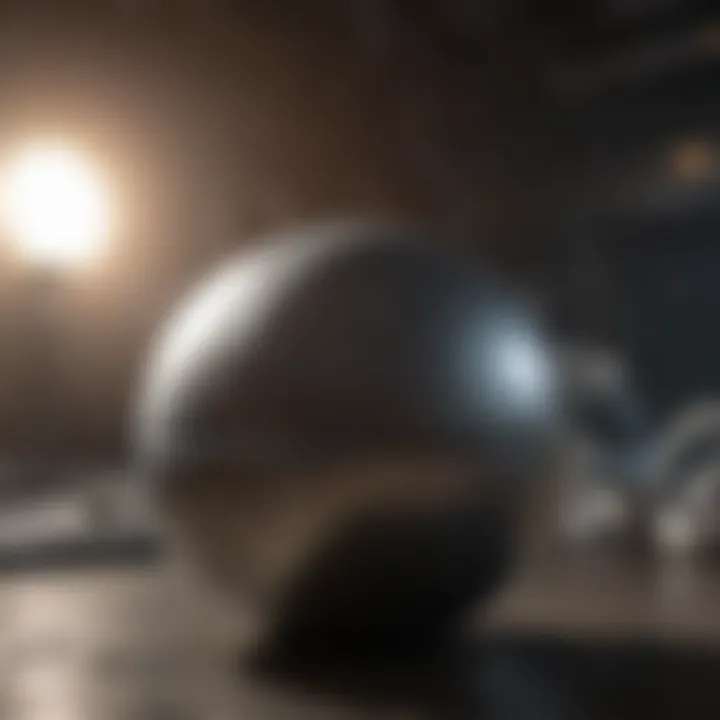
"The photoelectric effect illustrates the vital interplay between radiation energy and electron behavior, impacting the design of radiation shields in practical applications."
Compton Scattering
Compton scattering occurs when a photon collides with a loosely bound electron, transferring some of its energy to that electron and changing the direction of the photon. This interaction is most prominent with intermediate energy X-rays and gamma rays. Unlike the photoelectric effect, the photon does not necessarily get absorbed; instead, it continues traveling but with reduced energy.
This process is particularly important in materials like copper, which can effectively scatter radiation. The density and atomic structure play a crucial role in enhancing Compton scattering, making certain metals advantageous for radiation shielding in environments with varying radiation energy. Essentially, the combination of density and atomic number can dictate how well a shielding material can mitigate radiation exposure through scattering.
Pair Production
Pair production is another mechanism where high-energy photons, typically above a threshold of 1.022 MeV, can transform into particle-antiparticle pairs, usually an electron and a positron. This phenomenon occurs in the presence of a nucleus, which conserves momentum during the transformation. This interaction usually happens in very high radiation environments, such as those near nuclear reactors or high-energy physics laboratories.
The ability of a metal to facilitate pair production depends largely on its atomic number as well. Heavier metals, particularly tungsten, can be more efficient at engaging this process, proving essential for optimal shielding in high-energy scenarios. While not as common as the photoelectric effect and Compton scattering, understanding pair production elevates our grasp of effective radiation shielding systems, especially in more extreme applications.
By examining these mechanisms - the photoelectric effect, Compton scattering, and pair production - we gain invaluable insights into how specific metals can shield against different forms of radiation. Each mechanism highlights the critical role of fundamental physics in practical applications, driving forward advancements in radiation protection technology.
Applications of Radiation-Blocking Metals
The significance of radiation-blocking metals cannot be overstated. These metals play a pivotal role in various fields, ensuring safety and protection in environments where radiation is prevalent. It is essential to understand how metals like lead, tungsten, and copper are employed in specific applications. The advantages that these materials bring—be it in healthcare or energy production—underscore their importance.
In fields ranging from medicine to nuclear energy, the application methods of these metals are tailored to suit their unique properties. Here, we'll take a look at three key areas where radiation-blocking metals have made a significant impact: medical radiation protection, nuclear power plants, and the aerospace industry.
Medical Radiation Protection
In healthcare, radiation is used for diagnostic procedures and treatments, notably in X-rays and cancer therapies. As treatments become more advanced, the need for effective radiation protection intensifies.
Consider the role of lead in hospitals. It is often utilized in lead aprons, shields, and walls to protect patients and staff. These aprons are particularly crucial during procedures involving high radiation exposure, as they help minimize unnecessary radiation doses.
- Benefits of Lead in Medical Applications:
- Excellent shielding capability
- Cost-effective solution for healthcare facilities
- Durable and easy to fabricate
However, the usage of lead does raise concerns, as prolonged exposure can pose health risks. Alternatives such as bismuth and other composite materials are gaining traction, offering safer alternatives without compromising effectiveness. Engaging in discussions about these materials is essential to maintain a safe environment for both professionals and patients.
Nuclear Power Plants
In the realm of nuclear energy, the weapons against radiation aren’t just metaphorical; they're very much literal. The shielding requirements in these facilities are paramount for safe operation. Metals like stainless steel and lead are critical in the design of reactor shielding systems, ensuring that radiation leaks do not endanger workers or the public.
Practical implementations focus on shielding walls, storage containers, and transport casks. For instance,
- Key Functions in Nuclear Plants:
- Reducing radiation exposure to plant personnel
- Protecting the environment from potential leaks
- Ensuring integrity during disposal and storage of radioactive materials
This meticulous attention to shielding has converted nuclear power into a reliable energy source while addressing safety concerns. As technology evolves, so does the conversation about the types of metals used, emphasizing the need for innovation in shielding designs and materials.
Aerospace Industry Considerations
When it comes to the aerospace sector, radiation from cosmic rays poses a challenge during manned spaceflight. As astronauts travel beyond the Earth’s atmosphere, they require superior radiation shielding to protect against high-energy particles.
Metals that are lightweight yet effective are crucial here. Aluminum, known for its lightweight nature and reasonable strength, finds its utility in spacecraft design. Yet, for better protection, layering aluminum with components like polyethylene can optimize radiation blocking.
- Priorities in Aerospace Shielding:
- Balancing weight and shielding effectiveness
- Ensuring crew safety for long missions
- Considering the materials’ response to impacts and extreme conditions
In summary, the applications of radiation-blocking metals are diverse and criticallyimportant across multiple sectors. Each application highlights the need for ongoing research and development to improve existing materials and explore new ones. Keeping abreast of these advancements can lead to better protective measures in healthcare, energy production, and space exploration.
"The use of radiation-blocking metals is not merely a precaution; it's a fundamental necessity in safeguarding health and safety across industries."
By keeping these applications in mind, we can appreciate the vital role that metals play in safeguarding individuals, societies, and our planet from the dangers of radiation.
Innovations in Radiation-Blocking Materials
The development of radiation-blocking materials is an ever-evolving field, assumed necessary due to the increasing prevalence of radiation exposure in various sectors such as healthcare, nuclear energy, and aerospace industries. Embracing innovations in this area is not optional; it’s crucial for enhancing safety, efficiency, and performance in products designed to shield against harmful radiation. As the demand for these materials continues to rise, so does the necessity for materials that combine both effective shielding capabilities and practical attributes like weight, flexibility, and cost-effectiveness.
Composite Materials
Composite materials, by their very nature, weave together different substances to harness their strengths while mitigating their weaknesses. When it comes to radiation shielding, composites blend heavy metals with lighter elements, creating a versatile solution that's both effective and manageable. For instance, combining lead with polymer compounds allows for a significant reduction in weight while maintaining effective radiation blocking.
Some notable benefits of composite materials include:
- Enhanced Performance: Many composites demonstrate improved efficiencies compared to their single-material counterparts.
- Tailored Solutions: The ability to customize composites means they can be engineered for specific radiation types or applications.
- Durability: These materials often endure wear and tear better than traditional metals, extending their lifespan in demanding environments.
- Cost-Effectiveness: While the initial investment might be higher, the long-term savings due to reduced material costs and enhanced durability can offset those expenses.
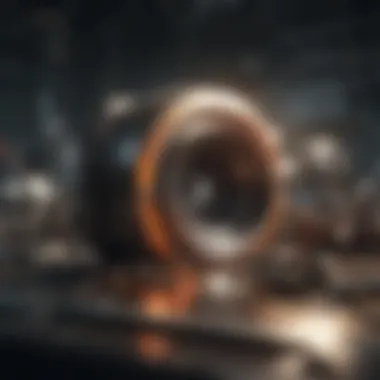
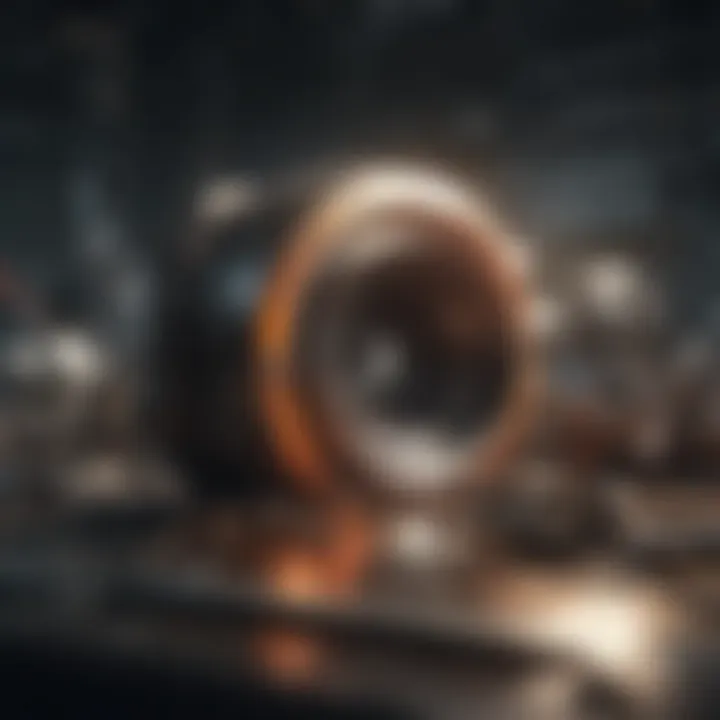
Investments in research into composite materials have proven fruitful. For example, scientists have been experimenting with resin and fiber combinations that not only block radiation but also exhibit superior mechanical properties. These innovations empower industries from healthcare to aerospace, making radiation protection more accessible without compromising safety.
Nanotechnology Applications
Nanotechnology represents a frontier in radiation blocking, leveraging materials on an atomic or molecular scale. It offers unprecedented opportunities to enhance the effectiveness of shielding materials. At the nanoscale, the behavior of materials changes, often leading to unexpected results that can bolster performance.
Key highlights regarding the application of nanotechnology in radiation shielding include:
- Improved Absorption: Nanoscale materials can absorb radiation more efficiently than bulk materials, leading to more compact shielding designs.
- Functionalization: Nanotechnology allows for materials to be engineered with specific properties, enhancing their effectiveness for particular radiation types. This specificity means that solutions can be optimized for medical, industrial, or space applications, addressing the unique challenges presented by different radiation environments.
- Lightweight Solutions: Utilizing nanoparticles often results in lighter materials, which is particularly beneficial in applications like aerospace where weight is a critical factor.
- Environmental Impact: Some research is exploring biodegradable nanomaterials, making shielding solutions more environmentally friendly.
"The fusion of nanotechnology and traditional shielding materials is opening up possibilities once deemed impossible."
As research progresses, it’s exciting to consider how innovations like these may play out in the next decade. This could mean the difference between cumbersome shielding solutions and sleek, reliable designs that provide optimal safety without added bulk. The potential applications in fields such as medical diagnostics, radiation therapy, and even space exploration make this a promising area of study.
Regulatory Standards and Guidelines
The subject of regulatory standards and guidelines in radiation shielding is pivotal in ensuring safety across various industries that deal with radiation. Recognizing the hazardous nature of certain metals and their interaction with radiation necessitates imposing regulations that not only minimize risks but also enhance the efficacy of protective measures. Standards serve as a backbone for compliance, guiding manufacturers, researchers, and practitioners in implementing reliable radiation protection.
Understanding regulatory standards is not merely an exercise in compliance; it's a framework that helps in fostering consistency and promoting best practices. Industries that utilize radiation, such as medicine, nuclear power, and aerospace, find immense value in adhering to established guidelines that ensure their shielding materials are effective and safe. These standards also facilitate better communication among scientists, engineers, and health professionals, ensuring that everyone is on the same page when it comes to safety protocols.
"Standardization is essential. It enhances safety and gives credibility to radiation protection practices across the board."
International Safety Standards
International safety standards are formulated by numerous organizations, like the International Atomic Energy Agency (IAEA) and the World Health Organization (WHO). These bodies set forth regulations that are recognized globally, ensuring a baseline level of safety when working with radiation.
The following points summarize the essence of these standards:
- Global Consistency: Countries adopt these standards to enforce a level of uniformity in safety practices, which is particularly crucial in research that exceeds international borders.
- Performance Criteria: These guidelines lay out specific performance criteria for shielding materials, detailing the necessary qualities for effectiveness against different types of radiation.
- Emphasis on Risk Management: By following these international standards, organizations can better assess and mitigate risks associated with radiation exposure.
- Continual Updates: As technology and understanding of radiation evolve, international standards are routinely updated, ensuring that practices adapt to the latest scientific findings.
National Regulations
While international standards provide a broad outline, national regulations take into account local conditions, practices, and specific needs. Each country has its own regulatory framework that aligns with global standards but also incorporates regional peculiarities.
Key aspects of national regulations include:
- Compliance Monitoring: National bodies regularly inspect organizations to guarantee adherence to established safety standards, instituting penalties for non-compliance to deter lax practices.
- Tailored Applications: Different industries may require variable regulations based on the type of radiation and the specific metals employed. National guidelines provide the flexibility to adapt to local contexts.
- Public Safety: National regulations often emphasize protecting public health as a priority, influencing decisions on how radiation-blocking materials are produced and used.
These layers of regulatory oversight, both international and national, create a safety net. They ensure that metals employed in radiation shielding do not only serve their intended purpose but also protect human health and the environment.
Future Directions in Radiation Shielding Research
The topic of future directions in radiation shielding research is becoming increasingly important in light of advancements in technology and a growing understanding of various radiation sources. As society's reliance on medical imaging, nuclear energy, and aerospace travel continues to expand, the need for effective and innovative shielding strategies becomes paramount. This section explores specific elements of emerging research trends and interdisciplinary approaches that promise to enhance radiation protection.
Emerging Research Trends
There are several key trends shaping the future of radiation shielding materials. Among them:
- Advanced Materials: Researchers are exploring new compounds and innovative material structures that could provide superior shielding properties. For example, studies into polymers infused with heavy metals aim to combine lightweight characteristics with effective radiation blocking.
- Smart Materials: The development of materials that can adapt to changing radiation levels is gaining attention. Such smart materials may offer real-time shielding capabilities, optimizing protection when necessary.
- 3D Printing Technologies: This technology is paving the way for the creation of customized shielding solutions tailored to specific environments, whether that be a hospital setting or a nuclear facility. It reduces waste and allows for rapid prototyping.
- Bio-inspired Designs: Taking cues from nature, researchers are examining how biological systems shield themselves from harmful radiation, potentially leading to the development of novel materials that mimic these processes.
Each of these trends offers unique advantages and challenges, making them critical to both present and future research in the field.
Interdisciplinary Approaches
The complexity of radiation shielding calls for a cooperative effort that draws from various disciplines. Some prominent collaborative fields include:
- Material Science: The synthesis and analysis of new materials require a deep understanding of physics and chemistry to create substances that marry effective radiation blocking with practicality.
- Engineering Innovations: Structural engineers must work alongside radiological experts to design systems that integrate shielding into existing infrastructures, ensuring that safety does not compromise functionality.
- Health Physics: This field focuses on protecting individuals from radiation hazards, emphasizing the necessity of practical applications in medicine and industrial processes.
- Sustainability Studies: As environmental concerns continue to rise, integrating sustainable practices into the development of radiation shielding materials is becoming essential. This approach not only addresses regulatory requirements but also aligns with societal expectations.
"Innovation lies at the intersection of disciplines, where the confluence of ideas leads to creation."
The collaboration between these diverse disciplines fosters a rich environment for innovative problem-solving, allowing researchers to approach radiation shielding from various angles.
Epilogue
In our examination of metals that block radiation, we conclude that this is not just a technical subject, but one deeply intertwined with safety, innovation, and future applications in various fields. Understanding the effectiveness of metals like lead, copper, tungsten, aluminum, and steel in shielding against harmful radiation is pivotal. Each metal presents its own unique properties that contribute significantly to its application in real-world scenarios, such as in medical settings and nuclear facilities.
Summary of Key Findings
In summarizing our key findings, it is clear that:
- Different metals offer distinct advantages in terms of density, atomic structure, and overall effectiveness in radiation shielding.
- Lead, while traditional, remains a stalwart choice in many applications despite its weight and toxicity.
- Copper and tungsten shine due to their versatility and robustness, often being favored in environments where efficiency is paramount.
- The lightweight characteristics of aluminum make it ideal for aerospace applications, where weight is a critical factor.
- Steel emerges as a practical solution, combining strength with relatively lower costs, suitable for various industrial uses.
These findings highlight the intricate balance between properties such as density, cost, and shielding efficiency, which must be considered when selecting materials for radiation protection.
Implications for Future Applications
The implications of our findings extend beyond academia and into practical applications. As we leap into an era of renewed focus on energy production, medical technologies, and space exploration, the demand for more effective radiation shielding materials will only intensify. Important considerations include:
- Innovative Material Development: Future research needs to focus on developing composite materials and leveraging advances in nanotechnology. This can lead to lighter, more effective shielding solutions that could revolutionize industries.
- Regulatory Framework Evolution: As technology advances, so too must the regulations that govern the use and handling of these materials. Ensuring safety while promoting innovation will be crucial.
- Interdisciplinary Collaborations: The challenges of radiation shielding will require input from various scientific disciplines to enhance the understanding of material behavior under radiation exposure, broadening the scope of shielding applications.
Ultimately, as we seek to protect human health and improve operational efficiency in sectors like healthcare, energy production, and aerospace, continuous exploration and improvement of radiation-blocking metals will remain essential. Through this commitment, we harness not just metals, but the core of our advancement into safer, more efficient technologies.