Exploring Monoclonal Antibodies: Mechanisms and Applications
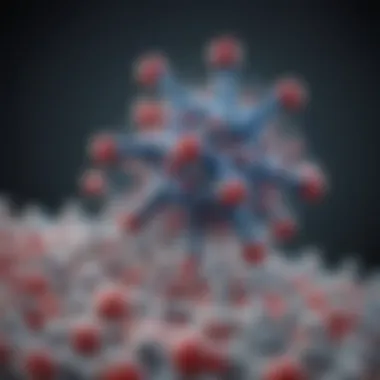
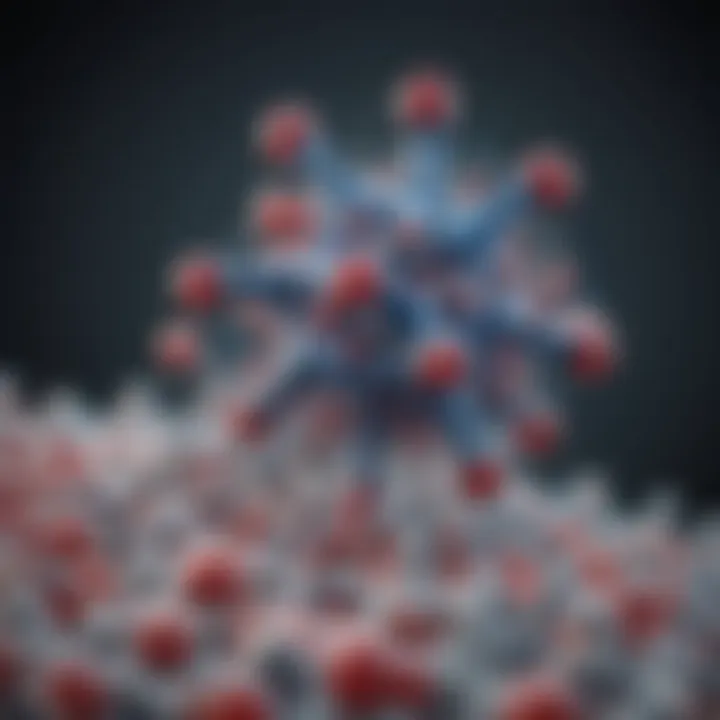
Intro
Monoclonal antibodies represent a remarkable leap in medical technology, serving as powerful tools in both diagnostics and therapeutics. Their creation and utilization have changed the landscape of treatment options for a multitude of diseases, from cancer to autoimmune disorders.
Often produced from a single clone of immune cells, these antibodies can target specific proteins or antigens with exceptional precision. In this article, we will navigate through the intricacies of monoclonal antibodies, examining how they are produced, their mechanisms of action, and their diverse applications in modern medicine.
Research Overview
Summary of Key Findings
The pivotal role of monoclonal antibodies in healthcare cannot be overstated. Key findings include:
- Enhanced Targeting: They provide targeted therapy, effectively diminishing damage to surrounding healthy cells.
- Broad Applications: Their use spans a wide array of conditions, notably cancer, infectious diseases, and inflammatory disorders.
- Innovation Potential: Ongoing research is revealing novel monoclonal antibody designs that may enhance efficacy and reduce side effects.
Background and Context
To understand monoclonal antibodies fully, it's essential to look back at their origin and development. Initially delineated in the late 1970s, these antibodies brought forth a revolutionary way to harness the immune system. Unlike traditional polyclonal antibodies which can target various antigens, monoclonal antibodies focus on a single epitope, offering high specificity. This is akin to using a scalpel instead of a machete—both can cut, but one does so with much greater precision.
The subsequent decades have witnessed tremendous advances in the production methodologies, including hybridoma technology and recombinant DNA techniques, leading to a rise in their therapeutic applications and making them vital components in contemporary clinical practice.
Methodology
Experimental Design
The study of monoclonal antibodies often involves intricate experimental designs, particularly when exploring their mechanisms. Typically, researchers create a hybridoma by fusing B cells with myeloma cells, thus generating a source of antibodies that can be cultured indefinitely. The specificity of these antibodies is critically assessed through various assays, ensuring that they bind effectively to the targeted antigens.
Data Collection Techniques
Data collection in this field relies on several techniques, which include but are not limited to:
- ELISA (Enzyme-Linked Immunosorbent Assay): This method quantifies proteins by utilizing enzyme-linked antibodies.
- Flow Cytometry: This technique allows for the analysis of the physical and chemical characteristics of cells or particles.
- Western Blotting: A powerful tool to detect specific proteins in a sample, validating the effectiveness of monoclonal antibodies.
Utilizing these methodologies aids in understanding how monoclonal antibodies function and evolve within various therapeutic and diagnostic settings, ultimately enhancing their impact on patient outcomes.
Improving patient care is at the heart of research into monoclonal antibodies. Each therapeutic advancement brings us a step closer to personalized medicine, where treatments can be tailored to individual patient needs.
Foreword to Monoclonal Antibodies
Monoclonal antibodies represent a cornerstone of modern therapeutic and diagnostic practices. Their unique ability to selectively target specific antigens has solidified their role in both research and clinical settings. The significance of monoclonal antibodies lies not only in their precision but also in their vast applications, ranging from treating cancers to diagnosing infectious diseases. Understanding the foundation of these biological tools is crucial for anyone involved in the medical and research fields.
Definition and Significance
A monoclonal antibody (mAb) is a laboratory-made molecule, engineered to mimic the immune system's ability to fight off harmful pathogens like viruses and bacteria. These antibodies are identical copies of a single type of immune cell, known as a B-cell, that has been selected because of its specific affinity for a particular epitope on an antigen. This specificity is a game-changer in both diagnosing and treating diseases.
The importance of monoclonal antibodies can be encapsulated in a few key points:
- Targeted Therapy: Unlike traditional methods that may affect all cells indiscriminately, mAbs can zero in on targeted cells, thereby minimizing damage to healthy tissue.
- Versatility in Applications: From cancer treatments like trastuzumab to diagnostic tests such as ELISA, their versatility is remarkable. They find use in a range of conditions, making them invaluable to medical practice.
- Research Utility: In laboratories, monoclonal antibodies facilitate a multitude of experiments, enabling scientists to track proteins, identify cells in tissues, and even conduct drug development.
Because of their precision, monoclonal antibodies have not just improved outcomes for patients, but they have also transformed the scientific landscape.
Historical Context
The journey of monoclonal antibodies began in the 1970s, driven by the groundbreaking work of Georges Köhler and César Milstein, who developed the first hybridoma technology. This method allowed for the production of large quantities of identical antibodies, thus giving rise to the term 'monoclonal.' The significance of this innovation cannot be overstated; it marked the onset of a new era in biomedical research and therapeutic interventions.
In the early years, the applications of monoclonal antibodies were mostly confined to research laboratories. However, by the late 1980s, the first monoclonal antibodies were approved for clinical use, with muromonab-CD3 hitting the market in 1986 as a treatment for transplant rejection. This paved the way for an explosion of monoclonal antibody drugs in various fields, particularly oncology.
Notably, the trajectory of monoclonal antibodies has been one of rapid evolution, moving from basic research tools to essential components of modern therapeutics. Today, the market is flooded with monoclonal antibody therapies aimed at various diseases, further demonstrating their indispensable role in contemporary medicine.
Mechanisms of Action
The mechanisms of action of monoclonal antibodies (mAbs) form the backbone of their effectiveness in both therapeutic and diagnostic applications. Understanding these mechanisms not only sheds light on how these antibodies can target specific cells but also highlights their role in modulating immune responses and engaging various biological pathways. This section will explore binding specificity, immune response modulation, and targeting mechanisms, providing insights that are crucial for anyone involved in related fields such as medicine, biotechnology, or research.
Binding Specificity
Binding specificity is one of the most defining features of monoclonal antibodies. Essentially, these antibodies are designed to bind to very specific antigens, usually found on the surface of pathogens or diseased cells. Think of it like a key fitting perfectly into a lock; if the antibody is the key, then the antigen is the lock.
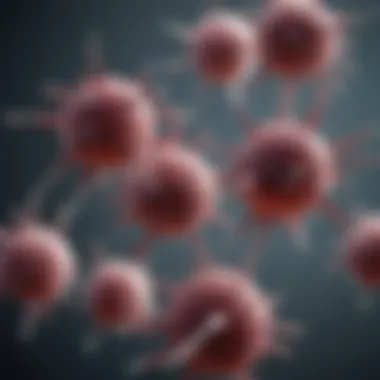
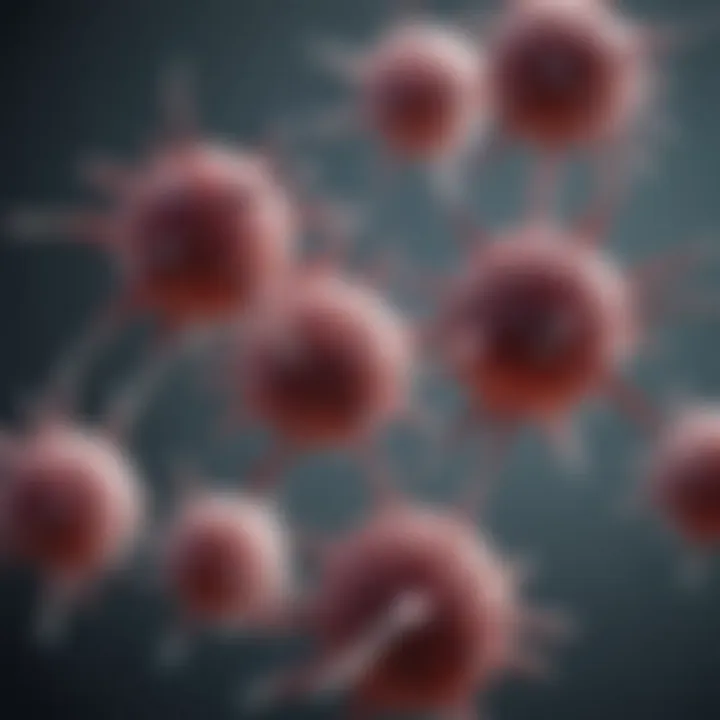
The mechanism works through high-affinity interactions, which means that once a monoclonal antibody locks onto its target, it can exert its effect without the risk of detaching easily. This specificity leads to numerous advantages:
- Minimized Damage: Only targeting certain cells reduces collateral damage to healthy cells.
- Enhanced Efficacy: The better the fit, the more efficient the antibody can clear the unwanted cells or pathogens from the body.
- Potential for Combination: When paired with other treatments, the unique binding can enhance the effectiveness of therapies.
This specificity is not just a matter of chance; it involves meticulous design and optimization at the molecular level. Different techniques also help in refining binding affinities, ensuring that the resulting products are as effective as possible.
Immune Response Modulation
Monoclonal antibodies also play a vital role in altering immune responses. They can either boost the immune system's activity or dampen it, depending on the therapeutic need. This modulation is critical in treating autoimmune diseases, where the immune system's hyperactivity can lead to tissue damage. Here's how they achieve this:
- Blocking Inhibitory Signals: Some monoclonal antibodies can block receptors or ligands that otherwise signal the immune system to halt its attack, thus enhancing immune activity.
- Enhancing Effector Functions: They can also promote the activity of immune cells, such as T-cells and natural killer cells, making them more effective in targeting cancers and infections.
- Cytokine Modulation: By binding to specific cytokines, these antibodies can regulate the inflammatory response, which helps in managing chronic conditions.
The ability to manipulate the immune system is a powerful tool in modern medicine. For instance, the monoclonal antibody Rituximab, used to treat certain types of lymphoma, functions by mediating a reduction of B-cells, demonstrating how these antibodies can scaffold entire therapeutic strategies.
Targeting Mechanisms
Targeting mechanisms are where monoclonal antibodies pull some impressive tricks from their sleeves. These mechanisms can be classified broadly into several categories:
- Direct Targeting: This involves binding directly to antigens on the surface of cancer cells, triggering cell death through various pathways.
- Antibody-Drug Conjugates: Here, mAbs are conjugated with cytotoxic drugs. The antibody directs the toxic agent straight to the tumor cell, minimizing side effects on healthy tissue.
- Immune Checkpoint Inhibition: Certain monoclonal antibodies block checkpoint proteins from binding with their partner proteins. This prevents the ‘off’ signal from being sent, allowing T-cells to kill cancer cells.
By leveraging these targeting strategies, monoclonal antibodies have transformed many treatment protocols. They have shifted the paradigm of cancer therapies from traditional chemotherapies to more refined and directed approaches, offering personalized treatment solutions.
"Monoclonal antibodies are not just another tool; they are a linchpin for future therapeutic innovations in disease management."
Production of Monoclonal Antibodies
Producing monoclonal antibodies is not just a technical feat; it's the backbone of numerous advancements in medical diagnostics and treatments. This process holds great importance as it not only influences the efficacy of therapies but also impacts patient outcomes and the overall costs associated with production and treatment. In this section, we dive into the various techniques and intricacies involved in monoclonal antibody production, emphasizing their significance in the landscape of modern medicine.
Hybridoma Technology
Hybridoma technology represents the first and perhaps one of the most pivotal methods for producing monoclonal antibodies. This technique was pioneered in the 1970s and has since become the gold standard in the field. The process involves fusing a specific type of immune cell, known as a B-cell, that produces an antibody with a myeloma (cancer) cell. The resulting hybrid cell, or hybridoma, retains the ability to produce the desired antibody while also undergoing infinite replication.
This method has several notable benefits:
- Specificity: Hybridomas can be engineered to produce antibodies that are highly specific to a particular antigen, ensuring that the resulting antibodies target only the intended biological markers.
- Quantity: Once established, hybridomas can proliferate indefinitely, generating large amounts of the desired antibodies at a significantly lower cost compared to polyclonal antibody production.
However, one must also consider some challenges that might arise:
- Immunogenicity: In some situations, human patients may mount an immune response against murine (mouse-derived) antibodies, raising concerns about safety and efficacy.
Recombinant DNA Technology
Recombinant DNA technology has revolutionized the production of monoclonal antibodies by allowing for more precise engineering of antibody molecules. This technique involves manipulating the genetic material of an organism to create altered protein products, offering a level of customization that hybridoma technology cannot achieve.
Through recombinant DNA technology, scientists can:
- Design Tailored Antibodies: Specific regions of antibodies can be modified to enhance either their binding characteristics or to avoid immunogenic reactions.
- Produce Humanized Antibodies: This approach minimizes the risk of eliciting an immune response from human patients since these antibodies are designed to closely resemble human antibodies.
While the potential of this technology is vast, it does come with its own array of considerations, such as the regulatory hurdles related to genetically modified organisms and the complexity of the overall production process.
Scaling Up Production
As the demand for monoclonal antibodies continues to rise, the scalability of production becomes crucial. Scaling up production poses both a challenge and an opportunity to improve access to these vital medical tools. Here, we will explore methods and considerations in effectively increasing the production volume of monoclonal antibodies.
Some strategies include:
- Bioreactors Optimization: Utilizing advanced bioreactors can allow for a more controlled environment for cell growth, thereby optimizing yield and efficiency.
- Process Automation: Automating various stages of production, from culturing cells to purification, can help ensure consistency and reduce costs in large-scale operations.
Nevertheless, the process is not without its issues, such as the need for stringent quality control measures to ensure that antibodies meet safety and efficacy standards. This attention to quality is paramount, as any defects in the production process can pose significant risks to patients.
Overall, the production of monoclonal antibodies is a meticulous and complex endeavor, yet it provides invaluable contributions to the realm of healthcare. By understanding and refining these production techniques, particularly hybridoma and recombinant DNA technologies, we pave the way for groundbreaking advances in diagnosis and treatment across various medical fields.
Therapeutic Uses
The application of monoclonal antibodies in therapeutic contexts marks a revolutionary shift in modern medicine. Their ability to target specific antigens makes them vital in treating a wide array of diseases, offering precision that was previously unattainable. This section discusses several critical domains where monoclonal antibodies have made their mark, particularly in oncology, autoimmune diseases, and infectious diseases. Each category not only highlights how these antibodies function but also emphasizes their transformative impact on treatment methodologies.
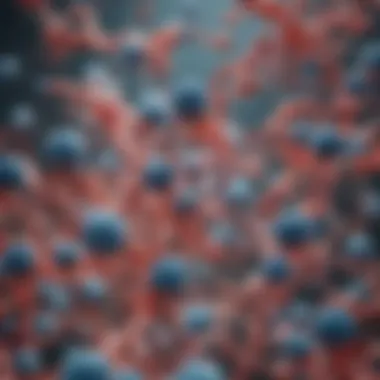
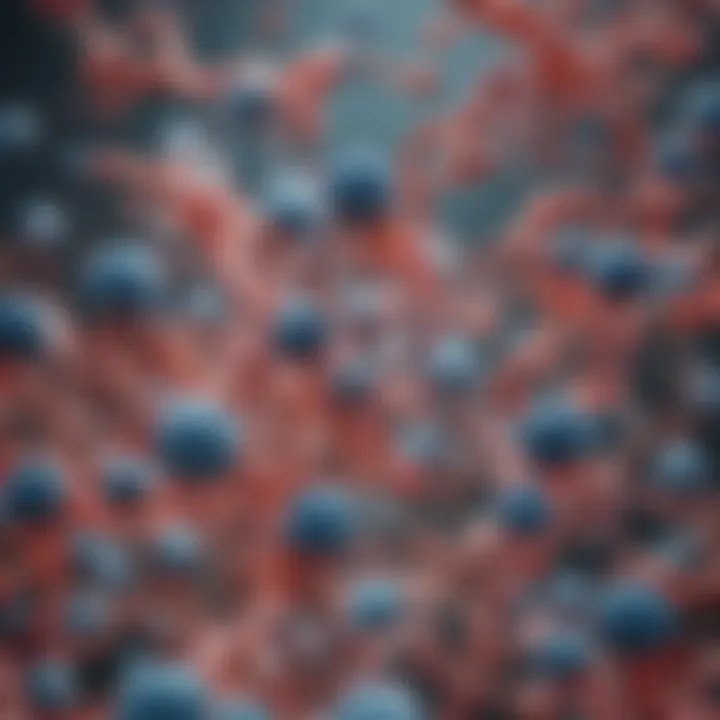
Oncology Applications
Monoclonal antibodies have carved a niche within the realm of oncology, a field where the need for innovative therapies is pressing. One of the most prominent examples is the use of rituximab, which targets CD20 on B cells, making it exceptionally effective against certain types of non-Hodgkin lymphoma and chronic lymphocytic leukemia. This treatment has significantly improved patient outcomes, often transforming a previously terminal diagnosis into a manageable condition.
Further, monoclonal antibodies can function in various ways:
- Direct Modulation: Some antibodies may work by blocking the growth signals received by cancer cells, hampering their ability to proliferate.
- Immune System Engagement: Others can recruit the immune system to target and destroy tumor cells, an approach epitomized by treatments such as trastuzumab for HER2-positive breast cancer.
In addition to these mechanisms, combination therapies utilizing monoclonal antibodies alongside traditional treatments, like chemotherapy or radiation, have become commonplace. Researchers are now focused on next-generation monoclonal antibodies that can target multiple antigens, potentially enhancing efficacy while reducing side effects.
Autoimmune Diseases
In the realm of autoimmune diseases, monoclonal antibodies offer hope where conventional treatments often fall short. Conditions such as rheumatoid arthritis, lupus, and multiple sclerosis can benefit from targeted therapies that specifically inhibit pathways contributing to the disease process. An example is adalimumab, which blocks tumor necrosis factor-alpha (TNF-α), a pro-inflammatory cytokine crucial in autoimmune responses.
These targeted monoclonal therapies provide several benefits:
- Precision: By focusing on specific immune pathways, they minimize collateral damage to healthy tissues, resulting in fewer side effects compared to broad immune suppressants.
- Long-term Remission: Many patients experience significant relief and longer periods of remission, which greatly enhances their quality of life.
However, challenges remain, such as the risk of developing anti-drug antibodies (ADAs), which can neutralize the effect of the treatment. Ongoing research aims to address these issues and optimize the therapeutic strategies involving monoclonal antibodies.
Infectious Diseases
The landscape of infectious diseases has also been altered by the incorporation of monoclonal antibodies in treatments and diagnostics. For instance, monoclonal antibodies have been instrumental against viral pathogens, including the treatment of Ebola and respiratory syncytial virus (RSV). The utility of therapies like palivizumab, which binds to RSV, underscores how these antibodies can provide passive immunity to particularly vulnerable populations, such as premature infants or those with underlying health conditions.
Moreover, monoclonal antibodies are increasingly deployed in diagnostic contexts as well, enabling rapid identification of pathogens. Their specificity is leveraged to develop sensitive assays for HIV, hepatitis, and even emerging infectious diseases.
The potential of monoclonal antibodies in the setting of infectious diseases is not just about treatment. It is also about prevention and early detection, offering a dual approach to combating infections.
In summary, therapeutic uses of monoclonal antibodies extend beyond what was traditionally thought possible. They provide not only targeted therapies but also encourage a shift towards more personalized treatment options. Their versatility continues to be a subject of intense research, paving the way for innovative solutions to some of the most pressing health challenges.
Diagnostic Applications
The realm of diagnostics faces constant evolution, and monoclonal antibodies play an essential role in shaping its future. Diagnostic applications are critical not only for identifying diseases but also for guiding treatment decisions and monitoring responses. With their inherent specificity and ability to bind distinct antigens, monoclonal antibodies enable enhanced accuracy in various tests. This section delves into significant aspects of how they are utilized in diagnostics, particularly in oncology, infectious diseases, and autoimmune disorders.
Cancer Diagnostics
Cancer detection early on can greatly influence prognosis, and monoclonal antibodies are fundamental here. Utilizing these antibodies can lead to the identification of tumor markers, specific proteins found on cancer cells. Tests like the CA-125 for ovarian cancer or HER2 for breast cancer exemplify how monoclonal antibodies facilitate diagnosis and help tailor therapies to individual tumors. By targeting these markers, the specificity is enhanced, allowing for less invasive yet robust detection methods.
"Monoclonal antibodies represent a game changer in oncology diagnostics, offering precision in tests that can lead to timely interventions."
Moreover, advancements in imaging techniques now incorporate monoclonal antibodies tagged with radioactive isotopes. These enable clinicians to visualize tumors accurately, shining a light on otherwise hidden malignancies. An added benefit is that these methods contribute less strain to patients compared to traditional biopsy techniques, which can be invasive and painful.
Infectious Disease Testing
Infectious diseases present a different diagnostic challenge. Here, monoclonal antibodies serve as a rapid response tool. Take, for instance, the use of these antibodies in the testing for HIV and COVID-19. Tests that utilize monoclonal antibodies can detect specific viral proteins or antibodies present in the patient’s blood, leading to faster diagnostics.
The specificity of monoclonal antibodies assists not only in confirming infections but also in distinguishing between different pathogens. This specificity helps eliminate false positives which can lead to unnecessary anxiety and treatment. Furthermore, the development of lateral flow assays that use monoclonal antibodies has revolutionized point-of-care testing, allowing for real-time diagnosis, even in remote settings.
Autoantibody Detection
Understanding autoimmune diseases often hinges on detecting autoantibodies, which are produced when the immune system mistakenly targets the body’s own cells. Monoclonal antibodies have simplified the identification of these markers in conditions like rheumatoid arthritis and lupus. The advantage here lies in their ability to capture autoantibodies with high affinity, reducing background noise in tests and improving diagnostic accuracy.
They can be employed in various formats, from enzyme-linked immunosorbent assays (ELISA) to more advanced multiplex assays that simultaneously test for several autoantibodies. This versatility and efficiency streamline diagnosis, greatly aiding in the management and treatment decisions for patients with autoimmune disorders.
In summary, the role of monoclonal antibodies in diagnostics emerges as a cornerstone in medicine today. They enhance detection methods across a range of diseases, bringing newfound accuracy and efficiency, ultimately contributing to better patient outcomes.
Challenges and Limitations
In the fast-evolving landscape of biomedical research and clinical application, the potential of monoclonal antibodies is hardly under debate. However, as with any groundbreaking technology, there are distinct challenges that could put a brake on their progress. Understanding these limitations is crucial for researchers and healthcare professionals who aim to harness the full power of monoclonal antibodies in medicine. The following sections dive into key challenges including immunogenicity, cost of production, and regulatory hurdles.
Immunogenicity
The issue of immunogenicity in monoclonal antibodies is a thorny one. Simply put, immunogenicity refers to the ability of a substance to provoke an immune response. For monoclonal antibodies, this can be particularly problematic if the patient's body recognizes the therapeutic proteins as foreign invaders. This reaction can lead to adverse effects, such as reduced efficacy of treatment or even severe allergic reactions.
- Consequences:
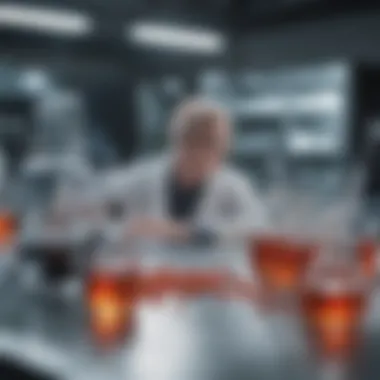
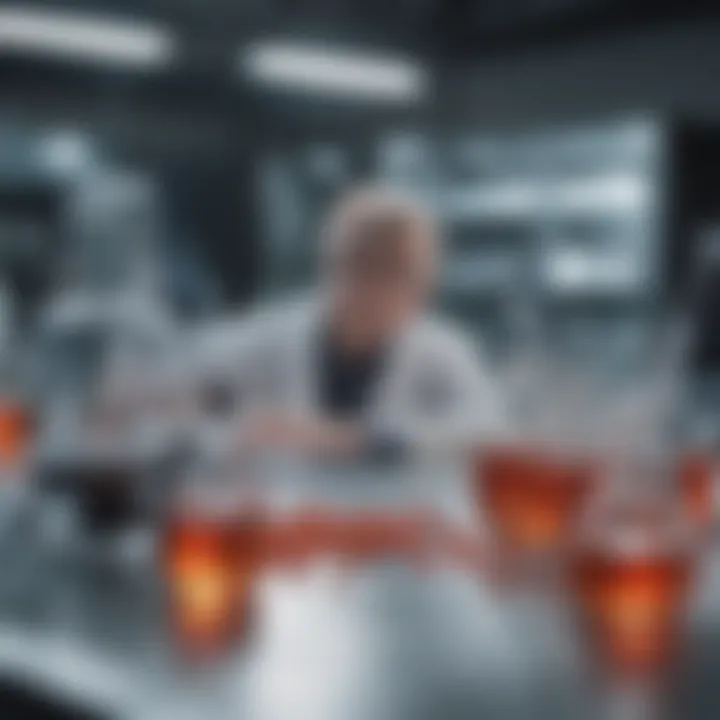
- Decreased therapeutic effectiveness
- Risk of anaphylaxis
- Development of neutralizing antibodies that can render treatments useless
- Potentially dangerous side effects
Strategies such as humanization of antibodies or the use of fully human monoclonal antibodies, like those derived from phage display libraries, are developed to mitigate this issue. However, there's no silver bullet; each patient’s immune system is unique, creating variability in response.
Cost of Production
Putting the complexity of monoclonal antibody production into context, the costs can be staggering. The production process, starting from the initial immunization of animals to the final purification of antibodies, is labor-intensive and requires advanced technology.
- Cost Factors:
- Materials: High-quality reagents and cell lines contribute significantly to the cost.
- Facilities: The need for specialized laboratory environments increases overhead.
- Labor: Skilled personnel are vital, and their training takes time and money.
These factors make monoclonal antibodies among the more expensive treatments available. This poses a barrier for many healthcare systems and patients, particularly in lower-income regions, where access to effective therapies remains limited.
Regulatory Hurdles
Navigating the regulatory landscape for monoclonal antibodies can often feel like traversing a minefield. Given the potential risks associated with their use, regulatory bodies such as the Food and Drug Administration (FDA) require extensive clinical trials and detailed documentation before any new monoclonal antibody can reach the market.
- Key Regulatory Concerns:
- Safety and Efficacy: Extensive and rigorous trials must demonstrate that the antibodies are safe and effectively treat the targeted conditions.
- Post-market surveillance: Once approved, continuous monitoring for adverse effects is mandatory.
- Labeling and manufacturing: Regulatory guidelines are stringent regarding how monoclonal antibodies are labeled and produced to ensure patient safety and consistency.
These requirements can delay the introduction of new therapies and drive up the costs associated with developing and producing monoclonal antibodies.
As the field of monoclonal antibody research grows, understanding these challenges becomes even more crucial. Balancing innovation with caution will ensure that the potential of monoclonal antibodies is fully realized for future applications in medicine.
"Understanding the limitations of monoclonal antibodies is as vital as their production; it sets the stage for overcoming obstacles in clinical applications."
By grasping these aspects, stakeholders can better navigate the complex landscape of monoclonal antibodies and work towards refining and advancing this promising therapeutic approach.
Future Prospects in Monoclonal Antibody Research
The future of monoclonal antibody research is both exciting and filled with potential. Given their unique ability to specifically target antigens, these antibodies hold promise for revolutionizing various fields of medicine and biotechnology. This section takes a closer look at what lies ahead, exploring next-generation antibodies, combination therapies, and personalized medicine.
Next-Generation Antibodies
Next-generation monoclonal antibodies are designed to enhance efficacy and reduce adverse effects in patients. One of the main focuses of current research is the development of bispecific antibodies, which can bind to two different antigens at once. This provides a strategic advantage in targeting disease mechanisms that involve complex interactions. For instance, in cancer treatment, these antibodies might harness a patient's immune response by targeting both a tumor antigen and a CD3 molecule on T-cells.
Moreover, advancements in engineering techniques like phage display and transgenic mice technology promise to create antibodies with unprecedented specificity and affinity. These enhancements can potentially lead to better therapeutic outcomes and even long-term remissions in chronic conditions.
"Next-generation antibodies could lead to treatments that were once thought impossible, paving the way for breakthroughs in challenging diseases."
Combination Therapies
Combining monoclonal antibodies with other therapeutic modalities offers a powerful way to tackle complex diseases, especially cancers. For example, monoclonal antibodies used in conjunction with checkpoint inhibitors are showing great promise. This multi-pronged approach can more effectively dismantle the defenses that tumors build against the immune system.
The challenge lies in determining the optimal combinations for various patient populations. With the aid of biomarkers and personalized medicine strategies, clinicians can select treatment plans specifically tailored to individual responses. As this field progresses, the aim will be to fine-tune combination regimens to maximize efficacy with minimal side effects, thus improving patient quality of life.
Personalized Medicine
The role of monoclonal antibodies in personalized medicine is another crucial frontier. Each patient’s disease profile is unique, and understanding this variance can help design therapies that are most effective on a case-by-case basis. Genetic profiling is already being used to identify which patients are likely to benefit from specific monoclonal antibody treatments.
Additionally, companion diagnostics are emerging as a key component of this personalized approach. These diagnostics assess an individual’s genetic makeup or the specific characteristics of their tumors, ensuring the selected monoclonal antibody matches the patient’s requirements.
This trajectory towards personalized care not only stands to improve treatment efficacy but also to reduce unnecessary side effects, allowing patients to lead healthier lives during therapy.
Finale
In wrapping up our exploration of monoclonal antibodies, it is crucial to reflect on the significance these tools hold within both therapeutic and diagnostic landscapes. Monoclonal antibodies have not only revolutionized treatment paradigms across various diseases but have also set the stage for innovative diagnostics that can provide invaluable insights into patient health.
Summary of Key Points
As we traverse through the various applications and mechanisms of monoclonal antibodies, several key points stand out:
- Mechanisms of Action: These antibodies engage specifically with target antigens, either stimulating or suppressing immune responses as needed.
- Production Techniques: From hybridoma technology to recombinant DNA methods, scientists have developed methods to generate these complex molecules in large quantities.
- Diverse Applications: Their therapeutic roles in oncology, autoimmune diseases, and infections demonstrate their versatility and effectiveness.
- Challenges: Issues such as immunogenicity and production costs can hinder their widespread utilization, making it essential for ongoing research to address these hurdles.
- Future Prospects: The future seems bright with promising avenues like next-generation antibodies and personalized medicine transforming patient care.
Impact on Future Research
The implications of monoclonal antibody technology extend far beyond what we see today. As researchers continue to innovate and refine these techniques, we can expect:
- Enhanced Specificity: Future antibodies may be engineered to bind even more precisely to target antigens, minimizing side effects while maximizing therapeutic benefits.
- Combination Therapies: By pairing monoclonal antibodies with other treatments, such as small molecules or gene therapies, the effectiveness of interventions can be dramatically increased.
- Personalized Approaches: Customizing treatment based on individual patient profiles could become a standard practice in healthcare, with monoclonal antibodies at the forefront of these advances.
The endeavor of researching monoclonal antibodies will only intensify, promising to push the envelope in medical science. As we transition into an era of personalized medicine, these antibodies will undoubtedly play a vital role in shaping the future of healthcare.