Precision in Scientific Inquiry: Accuracy Across Disciplines
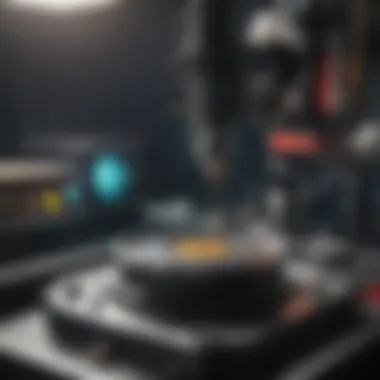
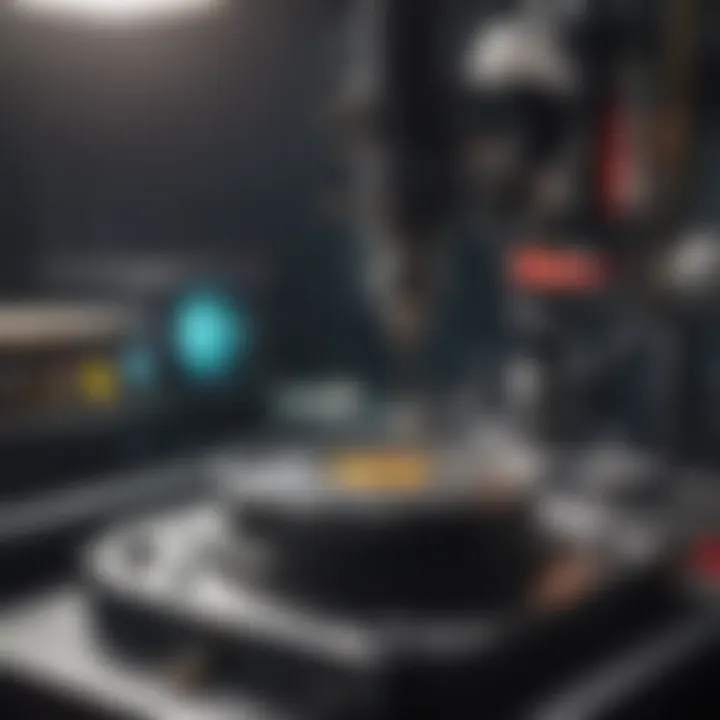
Intro
In scientific research, accuracy is fundamental. It not only drives the integrity of findings but also shapes further inquiry. Understanding how precision is maintained across disciplines is vital for researchers and educators alike. This exploration dives into the nuances of accuracy, examining its definition, measurement, and implications across various scientific fields.
Accurate measurements lay the foundation for credible conclusions. Whether it is assessing the speed of light in physics or quantifying cellular responses in biology, each discipline employs specific methods to ascertain accuracy. A closer look at these methods reveals the complexities involved in ensuring that results reliably reflect reality.
Through this discussion, we aim to provide clarity on the methodologies utilized within key scientific domains. We will also highlight the challenges faced in maintaining precision, as well as the ongoing efforts to improve research reliability.
This article serves as a guide for students, researchers, and professionals who seek a deeper understanding of the critical role accuracy plays in scientific inquiry.
Prologue to Accuracy in Science
Accuracy is a crucial element in the scientific method. It serves as the backbone for reliability and validity in research findings. When discussing accuracy, one is often referring to how close a measured value is to the true value. This concept transcends various fields of study, from physics to biology to chemistry. Each discipline has its own methods and standards of defining and measuring accuracy. In this section, we will unpack the intricacies of accuracy and discuss its vital role in fostering trust and credibility in scientific inquiry.
Defining Accuracy
Defining accuracy involves both qualitative and quantitative elements. It is not merely about numbers but also about the context in which those numbers exist. Accuracy is commonly assessed through calibration, comparison against standards, and statistical analyses. In essence, accuracy is determined by the degree of closeness of measurements to the actual value. What constitutes a 'true' value can be complex, but it often relies on established reference standards.
In scientific disciplines, accuracy is essential. For example, in chemistry, it could involve how closely a measured concentration matches an expected value. Similarly, in biological studies, ensuring accurate counts of cells can mean the difference between understanding a disease process and misdiagnosing it. Thus, a coherent definition of accuracy is foundational to effective research.
Importance of Accuracy in Research
The importance of accuracy in research cannot be overstated. Accurate data helps to form valid conclusions, which, in turn, inform public policy, medical practices, and technological advancements. An inaccuracy in data can lead to erroneous conclusions and potentially harmful consequences.
Accurate measurement contributes to:
- Model Validation: Reliable data enables researchers to validate or improve upon existing models.
- Decision Making: Accurate research informs decision-making on critical issues, such as health policies and environmental regulations.
- Reproducibility: Science thrives on reproducibility. Accurate methods and results are essential to ensure that others can replicate findings.
- Trust in Science: Public trust in scientific inquiry hinges on the accuracy of research findings. When accuracy is compromised, it can lead to skepticism and mistrust.
"Accuracy is not a luxury; it is a necessity in scientific inquiry and the trust of society in research"
In summary, accuracy plays a pivotal role in determining the integrity of scientific inquiry. Without it, the reliability of research findings collapses, thereby diminishing the purpose of science itself.
The Role of Accuracy in Physics
Accuracy plays a pivotal role in the field of physics. It dictates the reliability of measurements and influences the outcomes of experimental findings. In physics, precision is not simply a matter of numbers; it significantly affects the interpretations and applications of data collected. From fundamental principles to complex experiments, the role of accuracy underpins every facet of this discipline.
A high level of accuracy in physical measurements allows researchers to build upon previous findings confidently. This confidence can enhance the development of new technologies and theories. Conversely, inaccuracies can lead to flawed results, which may skew our understanding of physical laws and hinder technological progress.
Specific benefits of accuracy in physics include:
- Enhanced Experimental Validity: Accurate measurements validate theories and concepts, fostering scientific advancement.
- Improved Technological Development: Accurate data support the innovation of new technologies based on reliable information.
- Informed Decision Making: In industries relying on physical data, accuracy guides decisions that could affect safety and efficacy.
The considerations surrounding accuracy in physics are multifaceted. Researchers must continually assess the methodologies employed in their experiments, ensuring they adopt suitable measurement techniques and tools. An understanding of how errors can arise—be it systematic or random—allows physicists to refine their approaches and minimize inaccuracies in future work.
Accuracy in Biological Research
Biological research relies heavily on accuracy as it shapes the understandings and outcomes of scientific inquiry within the life sciences. The precision with which scientists collect and analyze biological data can significantly influence the conclusions drawn from their experiments. Accuracy in biological research is not just a metric; it serves as the foundation upon which valid hypotheses are built and theories are tested. This section will present key aspects of accuracy as it pertains to experimental design and the various challenges that researchers face in maintaining precision.
Experimental Design and Accuracy
The design of an experiment is crucial in determining the accuracy of the results achieved. A well-structured experimental design should consider several elements that contribute to precision and reliability.
- Control Mechanisms: Establishing control groups is vital. Control groups allow researchers to observe changes that occur due only to the experimental treatment, eliminating confounding variables.
- Sample Size: A larger sample size often leads to greater accuracy. This is because larger samples can reduce variability and provide a more accurate estimate of the population parameters.
- Randomization: Randomly assigning subjects to treatment and control groups helps to mitigate bias, hence enhancing accuracy. This ensures that each participant has an equal chance of being assigned to any group, which helps to maintain the integrity of the study.
- Replicates: Performing multiple replicates of an experiment helps confirm findings and improves accuracy. Replicates can reveal patterns or anomalies that single trials might miss.
- Instrumentation: Proper calibration of measuring instruments is essential. Inaccurate instruments could lead to erroneous data, skewing results and conclusions.
Ensuring accuracy in experimental design requires careful planning and execution. If any part of the design is flawed, the reliability of the entire research outcome can be compromised.
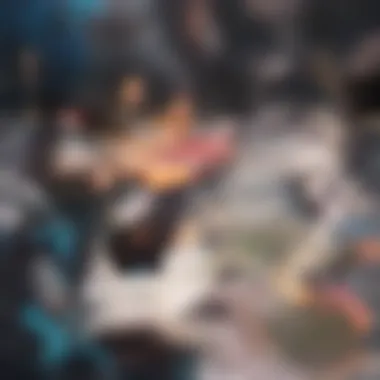
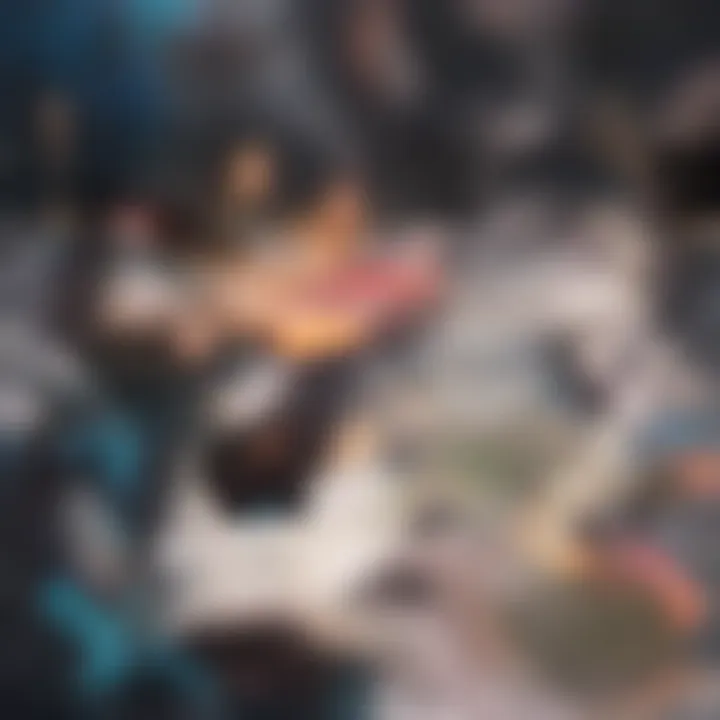
Challenges in Biological Accuracy
While the importance of accuracy in biological research is widely recognized, researchers face numerous challenges in achieving it. Some of these challenges include:
- Biological Variability: Living organisms are inherently diverse. Genetic differences among individuals can lead to variability in response to treatments or environmental conditions, complicating the analysis.
- Environmental Factors: Changes in external conditions, such as temperature or humidity, can affect biological experiments. Such factors must be controlled to avoid distorting results.
- Measurement Limitations: Tools and techniques for measuring biological processes may have inherent limitations. For example, errors can occur during sample collection, preparation, or analysis. This can lead to inaccuracies in data.
- Time Constraints: Biological research can be time-sensitive. Rushing through experiments may lead to oversight of critical steps that ensure accuracy.
- Subjectivity: In some biological fields, interpretation of data can be subjective. This might happen in areas such as histopathology, where visual assessments can vary among pathologists.
"Striving for precision in biological research is not just a methodological choice but a commitment to advancing knowledge reliably."
In summary, accuracy plays an essential role in the integrity of biological research. Through thoughtful experimental design and awareness of the challenges involved, researchers can work towards minimizing inaccuracies, thus enhancing the validity of their scientific findings. This ongoing effort is crucial for establishing credible claims in the ever-evolving field of biology.
The Significance of Accuracy in Chemistry
Chemistry relies heavily on accuracy. Precise measurements can decide the outcome of chemical reactions and subsequent conclusions drawn from those results. Inaccuracies can lead to erroneous findings, which may misguide future research or even impact public safety. Thus, understanding the significance of accuracy in chemistry is essential for building a reliable scientific framework.
The importance of accurate measurements in chemistry cannot be overstated. Accurate data allows chemists to replicate experiments, verify results, and develop theoretical models. Moreover, in a field where small discrepancies can yield large differences in outcomes, the role of accuracy becomes even more crucial. Without rigorous standards for measuring and reporting data, the integrity of scientific inquiry in chemistry may be compromised.
Analytical Methods and Their Accuracy
Analytical chemistry encompasses various methods aimed at determining the composition of substances. Techniques such as chromatography, spectrometry, and titration are often employed to achieve accurate results. Each method requires careful calibration and optimization to ensure precision. The suitability of a method often depends on the type of sample being analyzed and the desired outcome.
- Chromatography is utilized to separate components of a mixture, allowing for the determination of their concentrations. The accuracy here depends on the calibration of the system and the choice of stationary phase.
- Spectrometry measures the interaction between light and chemical substances. Accuracy relies on specific settings, including wavelength selection, and calibration against known standards.
- Titration requires precise addition of reagents until a reaction reaches completion. The endpoint must be accurately identified to ensure valid results.
Each technique faces challenges concerning accuracy due to factors such as operator error, instrument limitations, and external environments. Hence, continual improvement and rigorous validation of analytical methods are essential.
Impacts of Inaccurate Chemical Data
Inaccurate chemical data can have wide-ranging consequences. In research settings, erroneous data leads to flawed conclusions that can misdirect future studies. In practical applications, such as pharmacology, inaccurate data can result in unsafe dosages or ineffective treatments. Ultimately, the consequences extend beyond the laboratory, affecting public health and policy decisions.
Moreover, inaccuracies can undermine trust in scientific research. If results are repeatedly challenged due to faults in accuracy, the credibility of chemistry as a science may be called into question.
In summary, accuracy remains a key pillar in chemistry. It influences the validity of experimental outcomes, the safety of chemical products, and the trustworthiness of the discipline itself. Continuous efforts to enhance accuracy, through improved methodologies and robust validation processes, are vital in supporting the overarching goals of chemistry.
Earth Sciences and Accurate Measurements
The topic of Earth Sciences is essential for understanding the dynamics of our planet. Accurate measurements in this field play a crucial role in assessing environmental changes, predicting natural disasters, and managing natural resources. These measurements help scientists interpret geological processes, climatological data, and other Earth systems. The precision of these measurements affects not just scientific knowledge but also public policy and safety.
Accurate geological measurements help in identifying mineral resources, analyzing soil health, and understanding tectonic movements. Each of these aspects relies on rigorous methodologies that ensure data reliability. As the Earth continues to change due to various factors, it becomes imperative to adopt updated techniques in measurement that reflect this dynamism.
Geological Survey Techniques
Geological surveys require sophisticated techniques to gather accurate data. Geophysical methods, like seismic surveys, gravity measurements, and magnetic surveys, are pivotal. These techniques enable scientists to map subsurface structures without extensive drilling. They provide insights about natural resources, such as oil and gas reserves.
In addition to geophysical methods, remote sensing has transformed geological surveys. Satellites equipped with advanced sensors collect data about surface composition and changes over time. This provides a comprehensive view of large areas and reveals information that ground-based measurements might miss.
Moreover, GPS technology is crucial in measuring land movement. It allows researchers to track minor shifts in tectonic plates, potentially leading to better earthquake predictions. The integration of these technologies ultimately leads to more accurate interpretations of Earth's processes.
Consequences of Inaccuracy in Earth Sciences
Inaccurate measurements in Earth Sciences can have dire consequences. For instance, misinterpreting geological data can lead to poor resource management. This could result in over-extraction of resources or failure to discover new deposits, impacting economies.
Furthermore, inaccuracies in seismic data can mislead earthquake preparedness efforts. If scientists underestimate the magnitude of a potential quake, communities may not be adequately prepared. This can lead to increased casualties and damage when disasters strike.
"Accurate data is essential not just for scientific theory, but for practical applications that affect lives and economies."
In summary, precision in geological measurements directly influences public safety and environmental sustainability. As Earth Sciences continue to evolve, the necessity for improved accuracy remains a priority. Ensuring that these measurements are robust can help us better address the challenges posed by our changing planet.
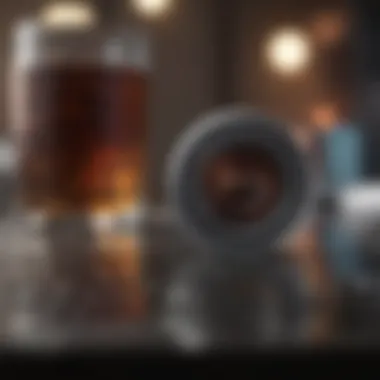
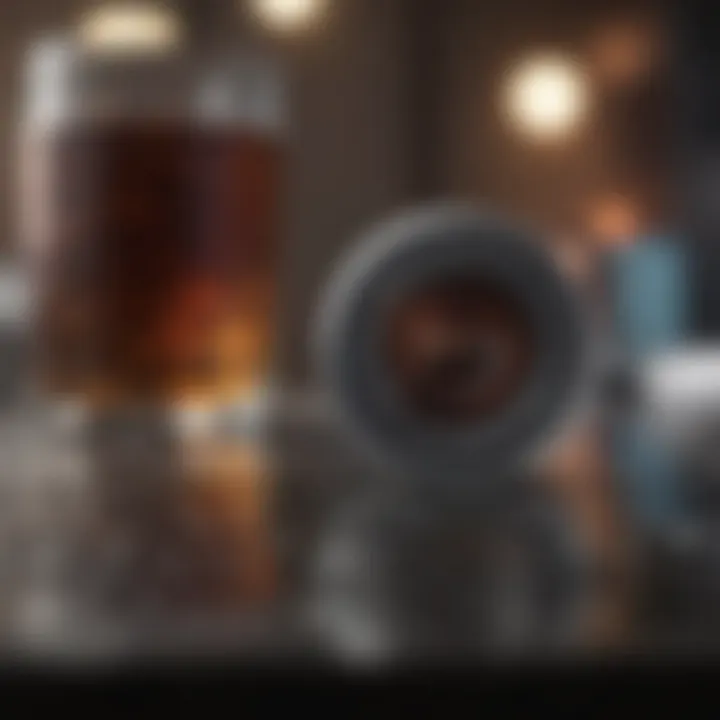
Methodologies for Ensuring Accuracy
Ensuring accuracy in scientific research is crucial to advancing knowledge and maintaining trust in findings. Methodologies for ensuring accuracy encompass various strategies aimed at reducing errors, improving reliability, and validating results across scientific disciplines. These methodologies not only enhance the quality of research but also provide frameworks that researchers can follow to ensure their work meets established standards.
Statistical Approaches to Assessing Accuracy
Statistical methods play a significant role in assessing accuracy. They enable researchers to quantify uncertainty and variability, allowing for a clearer understanding of the data's reliability. Common statistical approaches include:
- Descriptive Statistics: Summarizes data sets to provide a clearer picture of basic features. Measures like mean, median, and standard deviation help illustrate the precision of the data.
- Inferential Statistics: Facilitates generalizations from a sample to a larger population. Techniques such as confidence intervals and hypothesis testing are used to assess if findings are reliable and applicable beyond the sample.
- Error Analysis: Identifies potential sources of error in data collection and analysis. By assessing systematic and random errors, researchers can better understand the limitations of their findings.
By employing these statistical methods, researchers can critically evaluate the accuracy of their results, allowing them to make informed decisions based on quantitative evidence.
Calibration and Standardization Techniques
Calibration and standardization are vital in ensuring accuracy across scientific disciplines. These techniques are essential for minimizing discrepancies in measurement and enhancing reliability.
- Calibration: This involves comparing a measurement instrument with a standard reference to ensure accuracy. Regular calibration helps to identify any drift or bias in instruments, ensuring that subsequent measurements are reliable and accurate.
- Standardization: The establishment of consistent measurement protocols is key. Adhering to standardized methods helps ensure that data collected is comparable across different studies and contexts. For example, the use of standardized units and practices in clinical trials ensures that results can be trusted and replicated by other researchers.
Both calibration and standardization are foundational to maintaining the integrity of scientific research. They not only fortify the reliability of the results but also contribute to a collaborative environment where findings can be compared and validated against similar studies.
Ensuring accuracy through these methodologies is crucial for advancing knowledge and maintaining trust in scientific outcomes.
Ultimately, incorporating solid statistical approaches along with effective calibration and standardization techniques presents a comprehensive framework for ensuring accuracy across various scientific disciplines.
Technological Advances in Precision Measurement
Technological advances in precision measurement have fundamentally reshaped the landscape of scientific inquiry. As disciplines evolve, the need for ever more accurate data becomes paramount. These advancements not only enhance the precision of measurements but also enable scientists to explore previously uncharted territories within their respective fields.
One significant element of these advances is the development of highly sensitive measurement instruments. The introduction of devices such as atomic force microscopes and laser interferometers allows researchers to obtain measurements at atomic scales. This precise level of clarity is essential in fields such as nanotechnology, where even the smallest variations can lead to significant changes in materials and processes.
Additionally, advancements in data acquisition technologies have transformed how measurements are recorded and analyzed. High-speed data loggers and digital sensors produce large volumes of data that can be processed with unprecedented speed and accuracy. This capability not only improves the reliability of data but also supports more complex analyses that can reveal subtle trends and patterns.
"The pursuit of precision is not just a quest for number accuracy but also a path toward deeper understanding of scientific phenomena."
The integration of these technologies into routine experimentation marks a leap in scientific methodology. However, it is crucial to recognize the evolving nature of these technologies. With rapid changes, certain challenges emerge. For example, the calibration of advanced measurement tools requires ongoing attention. Improperly calibrated instruments can lead to erroneous data, affecting downstream research outputs.
In summary, technological advances have revolutionized precision measurement by improving sensitivity, data quality, and analysis capabilities. Still, researchers must navigate the complexities that accompany these innovations to maintain data integrity and accuracy in their work.
Innovations in Measurement Technology
Innovative technologies are at the forefront of enhancing measurement precision. One notable example is the development of quantum measurement techniques. These methods exploit quantum mechanics to achieve a level of accuracy unattainable by classical means. Quantum sensors, such as gravimeters and magnetometers, provide insights into physical phenomena that were previously difficult to observe.
Another promising innovation is the emergence of 3D printing in measurement tools. Customizable devices allow for bespoke solutions tailored to specific research needs. For instance, researchers can create unique probes or fixtures that adhere closely to their experimental goals without the constraints of traditional manufacturing processes.
Moreover, advancements in spectroscopy, including techniques like Fourier-transform infrared spectroscopy, have illuminated new pathways for analysis in chemistry and biology, further empowering researchers to derive accurate measurements with ease.
Impact of AI on Accuracy in Research
Artificial Intelligence (AI) has emerged as a transformative force in enhancing accuracy across various fields of research. One of its most impactful applications lies in data analysis. AI algorithms can swiftly analyze extensive datasets, identifying trends and anomalies that may elude manual analysis.
Machine learning models improve the prediction of experimental outcomes, allowing for real-time adjustments during experiments. This adaptiveness can minimize errors and boost the accuracy of results. For example, in drug discovery, AI helps in predicting which compounds are likely to succeed based on historical data, ultimately reducing the chances of inaccuracies in trial results.
Furthermore, AI techniques can also aid in improving calibration processes. Automated calibration systems powered by machine learning adapt and improve over time, ensuring that measurement devices maintain precision without extensive manual input.
Implications of Inaccurate Data
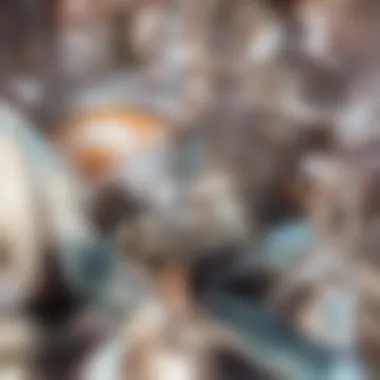
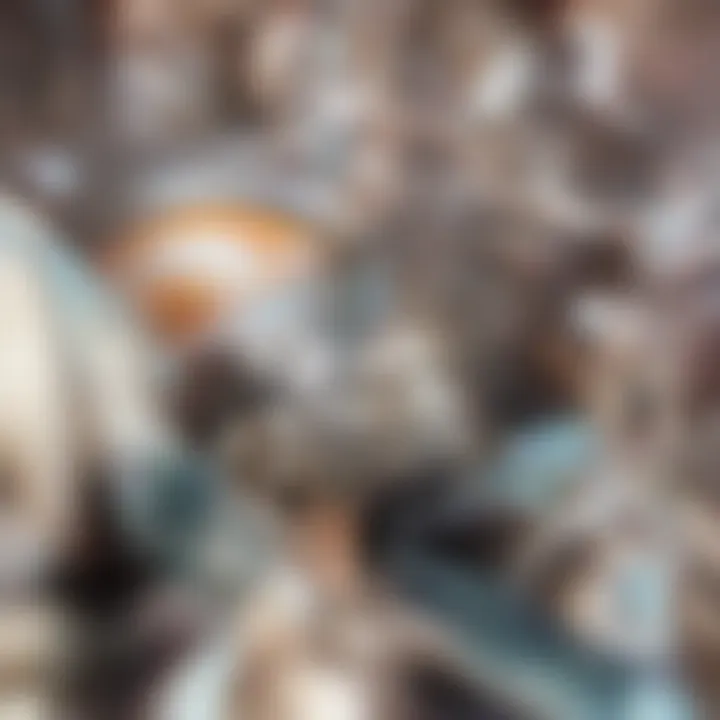
In the realm of scientific inquiry, the implications of inaccurate data are profound and far-reaching. These inaccuracies can originate from various sources, including flawed methodology, poor measurement techniques, or data processing errors. Understanding the significance of these implications allows researchers to appreciate the weight their findings carry in broader contexts. As such, accuracy is not merely an academic concern; it determines the integrity of research outcomes, guiding future studies and policy-making decisions.
Inaccurate data can lead to misinformed conclusions, which then influence further research and development. For instance, in health sciences, if a study misrepresents the efficacy of a treatment based on faulty data, it could result in incorrect medical guidelines and practices, potentially endangering patient health. Furthermore, public trust in scientific institutions can diminish when inaccuracies surface, highlighting the critical need for rigor in research practices.
"The reliability of data is the foundation upon which scientific integrity is built."
Ethical Considerations in Scientific Research
Ethics play a central role in scientific inquiry, especially concerning data accuracy. Researchers have a moral obligation not only to produce accurate findings but to uphold the standards of truthfulness and transparency in their methodologies. Deceptive reporting or selective presentation of data can severely undermine the credibility of the research. Academic integrity is paramount; thus, when inaccuracies are discovered, the additional ripple effects can involve retractions of published articles and reputational damage.
Researchers must consider the potential impact of their findings. When designing studies, they should prioritize accuracy from the beginning, incorporating robust data collection methods and validation processes. A commitment to ethical standards fosters a culture of credibility and trust within the scientific community.
Consequences for Public Policy and Trust
Inaccurate scientific data can have dire consequences for public policy. Policy decisions that are informed by erroneous findings could lead to inefficient allocation of resources or ineffective interventions. For example, environmental policies based on flawed climate data may fail to address pressing issues, leaving communities vulnerable.
Public trust in scientific research is also at stake. When citizens perceive that scientists provide unreliable information, their faith in scientific guidance diminishes. Trust is crucial in public health, climate action, and other areas where scientific recommendations directly influence daily life. Restoring that trust requires strict adherence to accuracy in data reporting.
In summary, the implications of inaccurate data extend beyond academia. They shape ethical frameworks, influence policies, and dictate the level of trust the public has in science. By prioritizing accuracy, researchers not only contribute to the advancement of knowledge but also uphold the essential pillars of reliability and integrity.
Future Directions in Accuracy Enhancement
In the landscape of scientific research, accuracy remains a pillar of credibility. This section discusses Future Directions in Accuracy Enhancement to address ongoing challenges across various disciplines. Enhancing accuracy involves adapting to technological advancements, evolving methodologies, and recognizing the interconnectedness of scientific inquiry. Each advancement not only serves to enrich research outcomes, but also fosters trust among stakeholders in the scientific community. It is crucial to stay attuned to emerging trends and collaborative efforts in accuracy research to drive innovation and reliability in findings.
Emerging Trends in Accuracy Research
The field of accuracy research is dynamic, evolving alongside technological advancements and scientific methodologies. Current trends show a significant movement towards integrating machine learning and data analytics in assessing accuracy. As researchers seek to enhance precision, predictive analytics can analyze vast datasets, revealing patterns that traditional methods may overlook. The inclusion of real-time monitoring tools also facilitates immediate adjustments and corrections in experimental designs.
Moreover, there is an emphasis on open science practices. These practices promote transparency and data sharing among researchers, allowing for collective validation of results. Open-source software and collaborative platforms offer researchers ways to improve accuracy collaboratively, enriching the scientific dialogue. Adopting these practices reflects a shift towards accountability, as they invite scrutiny and foster a culture of excellence in research.
"The pursuit of enhanced accuracy will likely depend on our ability to integrate interdisciplinary insights and leverage new technologies effectively."
Collaborative Efforts Among Scientific Disciplines
Collaboration across scientific disciplines plays a pivotal role in enhancing accuracy. As the complexity of global challenges increases, scientists must work together to develop innovative approaches. Interdisciplinary research teams bring unique perspectives and methodologies that can enhance the overall precision of findings.
Some notable examples include:
- Environmental Science and Biology: Joint studies on ecosystems leverage biological accuracy to inform environmental assessments effectively.
- Chemistry and Data Science: Chemists now collaborate with data scientists to model chemical interactions more precisely, ensuring accurate predictive capabilities in simulations.
- Engineering and Physics: Partnerships between these fields advance measurement technologies, leading to better instruments for capturing data in experimental setups.
The importance of these collaborations cannot be overstated. They foster an environment where diverse skill sets meet, leading to more accurate, reliable outcomes in research. By bridging gaps between various scientific domains, researchers can tap into a broader knowledge base, continuously enhancing the methodologies necessary for precision in inquiry.
Finale: The Pursuit of Accurate Knowledge
In the realm of scientific inquiry, accuracy stands as a fundamental pillar essential for achieving reliable and valid results. Throughout this article, we have explored various dimensions of accuracy across multiple disciplines including physics, biology, chemistry, and earth sciences. Each section has highlighted that precision is not merely a technical concern, but a philosophical underpinning that influences research methodologies, ethical considerations, and public trust in scientific findings.
The pursuit of accurate knowledge is crucial for several reasons. First, it enhances the credibility of scientific research. Without accuracy, data can mislead, causing inappropriate conclusions that impact further studies and applications. Second, accurate research fosters confidence among stakeholders, including policymakers, educators, and the general public. When findings are reliable, they can be effectively used to make informed decisions.
"Scientific accuracy is not just about numbers; it’s about truth and the foundation upon which progress is built."
Effective strategies for ensuring accuracy must be adopted widely. These strategies include stringent experimental designs, robust statistical methods, and regular calibration of instruments. The significance of adopting these measures cannot be overstated, as they directly influence the efficacy and impact of research outcomes.
Ultimately, the pursuit of accurate knowledge extends beyond immediate scientific endeavors. It shapes future research landscapes and fosters interdisciplinary collaboration, which is essential in overcoming complex global challenges. As we move forward, it is vital for scientists across fields to remain committed to accuracy, ensuring that their contributions to knowledge can lead to tangible benefits for society.
Summary of Key Points
- Central Role of Accuracy: Accuracy is fundamental to the credibility and reliability of research findings across disciplines.
- Credibility and Public Confidence: Accurate data fosters trust among stakeholders and informs sound decision-making processes.
- Methodological Standards: Emphasizing rigorous experimental design and statistical validation is crucial for achieving accuracy.
- Interdisciplinary Collaboration: A collective commitment to precision enhances the ability to address complex scientific challenges effectively.
The Continuing Importance of Accuracy
The importance of accuracy in scientific inquiry is an ever-present need. As fields advance and become more interconnected, the demand for high-quality, precise research escalates. Inaccuracies not only compromise individual studies but can have cascading effects on broader scientific understandings and societal implications.
In a rapidly evolving landscape, where interdisciplinary approaches are becoming more common, integrating precise methodologies across scientific disciplines is essential. This collaborative spirit fosters innovation while ensuring that accuracy is upheld as a critical value. Moreover, advancements in measurement technology and data analysis are continually shaping how accuracy is defined and achieved, making ongoing education and adaptation vital for researchers.