Pro Cellular: An In-Depth Analysis of Biology and Tech
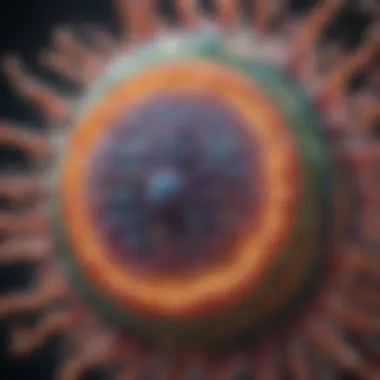
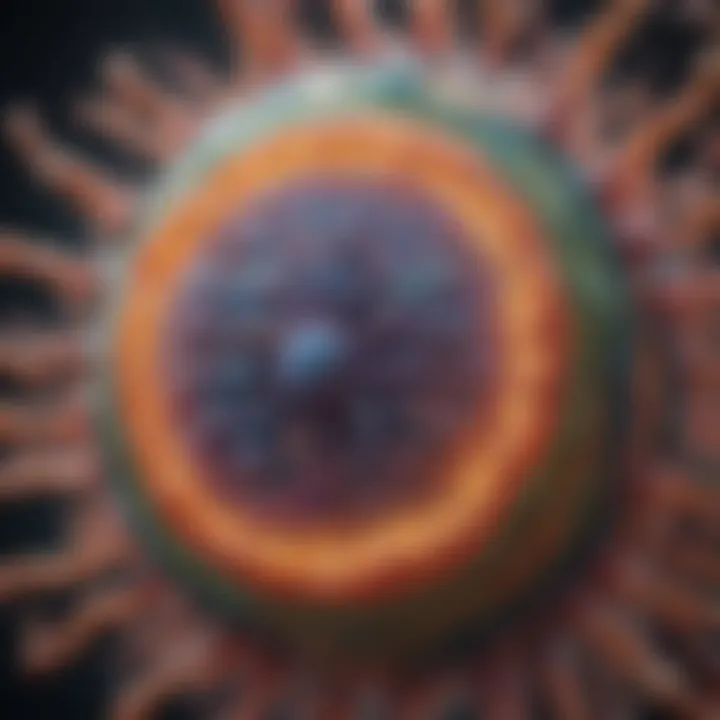
Intro
The concept of pro cellular encompasses various aspects of cellular biology and technology. It serves as a framework for understanding the intricate dynamics governing cellular functions and their applications in contemporary research. By examining cellular structures, signaling mechanisms, and advancements in biotechnology, we can illuminate the multifaceted implications of this concept. Additionally, we must consider the ethical dimensions associated with genetic manipulation and cellular therapies, topics that garner significant attention in both scientific and public discourse.
In this article, we will unpack these elements to provide a comprehensive understanding of pro cellular principles and their relevance. This exploration aims to engage students, researchers, educators, and professionals by presenting information in a detailed yet accessible manner.
Research Overview
Summary of Key Findings
The investigation into pro cellular phenomena reveals several important insights:
- Cellular Structures: Understanding the architecture of cells is foundational. This includes studying organelles, membranes, and cytoskeletons.
- Cellular Signaling: Cells communicate through complex signaling pathways that affect growth, differentiation, and response to environmental stimuli.
- Biotechnology Advancements: Innovations in biotechnology enable researchers to manipulate cellular processes for therapeutic purposes, leading to groundbreaking treatments.
- Ethical Considerations: As we advance in genetic manipulation, ethical dilemmas arise regarding consent, safety, and the implications of altering life at a fundamental level.
Background and Context
The study of cellular biology began centuries ago, with early microscopists contributing to our understanding of cell structure. Over time, the emphasis shifted towards not just what cells are made of, but how they function and communicate. The advent of molecular biology and genomics further transformed this field. Today, cellular research covers diverse areas such as regenerative medicine, cancer therapeutics, and synthetic biology.
With significant advancements in technologies like CRISPR and cellular reprogramming, the pro cellular concept emerges as crucial. We find ourselves at a junction where genetic intervention can lead to novel treatments but also demands careful consideration of ethical implications.
Methodology
Experimental Design
Research into pro cellular topics often follows a multidisciplinary approach. Studies may combine molecular biology, genetics, and bioinformatics to yield comprehensive results. Laboratory settings facilitate controlled experiments, while computational models allow for predictions about cellular behavior under various conditions.
Data Collection Techniques
Data collection in pro cellular research can involve techniques such as:
- Microscopy: Advanced imaging techniques provide insights into cellular structures and dynamics.
- Flow Cytometry: This allows for the analysis of physical and chemical properties of cells.
- Genomic Sequencing: Provides information about the genetic makeup and potential variations.
Through these techniques, researchers build a detailed understanding of cellular operations and their broader implications in science and technology.
Preface to Cellular Biology
Cellular biology serves as the cornerstone of biological sciences, intricately weaving through the fabric of life itself. This section highlights its importance, specifically in understanding the dynamics of life at the cellular level. The study of cells not only opens a window into how organisms function but also how they interact with their environments. Insights gleaned from cellular biology have potential implications in various fields, from medicine to environmental science.
Understanding cells lays the foundation for numerous scientific inquiries. Cells are the basic units of life, and thus, comprehending their structure and dynamics is vital for advancements in biotechnology, genetics, and medical research. This knowledge equips researchers and students alike to explore more complex biological systems, ultimately fostering innovation and discovery in related disciplines.
Detailed examinations of specific cellular components and functions contribute significantly to our knowledge base. By dissecting these elements, one can appreciate not just individual cellular processes but also their roles in larger biological systems. The connections formed across various disciplines—like molecular biology and genetics—further enrich the study of cellular biology, encouraging a multidisciplinary approach that can lead to groundbreaking research.
Understanding Cell Structure
Cell structure is fundamental for grasping how organisms function. Different components of a cell work synergistically to maintain life, each contributing to the overall goal of cellular health and efficiency.
Components of the Cell
The components of the cell, including organelles such as mitochondria, ribosomes, and the endoplasmic reticulum, play crucial roles in cellular functions. These components are often categorized by their distinct features. For example, mitochondria are vital because they generate energy through respiration and regulate metabolic activities. Their efficiency is indispensable for the sustainability of cellular processes.
Cell components not only serve vital functions but also represent targets for many scientific inquiries. By focusing on the organelles' unique characteristics, researchers can find ways to manipulate these functions for therapeutic and technological advancements. However, this dependence can lead to issues regarding cell resilience under stress or damage, raising questions about cellular adaptability.
Membrane Functions
The cellular membrane, known for its selective permeability, delineates the internal environment from the external. This function is crucial for maintaining homeostasis. Membranes facilitate communication between cells and their environments, allowing signals to pass, which is vital in processes such as signaling pathways and nutrient transport.
The unique feature of membrane functions lies in their fluid mosaic model. This model highlights the dynamic nature of membrane components that can change and adapt. While this fluidity allows for flexibility and responsiveness, it requires energy, which can be a limitation in specific cellular contexts.
Nuclear Characteristics
The nucleus is central to a cell's operations, housing genetic material and coordinating cellular activities. Its most notable characteristic is its double membrane, which protects DNA while allowing regulation of gene expression. This regulation is critical for cell differentiation and function.
Nuclear characteristics enable cells to adapt to their environment through gene expression changes. However, any disruption in nuclear integrity can lead to significant cellular dysfunction, which is observed in various diseases including cancer. Understanding these characteristics is essential for advancing research in genetic manipulation and therapies.
Basic Cellular Functions
Basic cellular functions encapsulate the fundamental activities that keep cells alive and functioning properly. Investigating these processes sheds light on not only biological mechanisms but also therapeutic potentials.
Metabolism
Metabolism encompasses all chemical reactions within a cell. From energy production to biosynthesis, metabolic processes are essential for cell survival. Cellular metabolism is categorized into catabolic and anabolic reactions, allowing cells to convert nutrients into usable energy.
The unique aspect of metabolism is its adaptability. Cells can modify metabolic pathways based on environmental conditions, which is crucial in a constantly changing landscape. Understanding metabolic mechanisms opens doors for drug development and metabolic engineering.
Reproduction
Cellular reproduction, whether through mitosis or meiosis, is integral to life continuity. This process ensures growth, development, and repair in multicellular organisms. Each form of reproduction serves different purposes—mitosis for growth and maintenance, meiosis for genetic diversity.
A key characteristic of reproduction is the accuracy and regulation of DNA replication. Any errors can lead to mutations that might affect an organism's viability. Thus, understanding reproductive mechanisms aids in the study of genetics and hereditary diseases.
Homeostasis
Homeostasis refers to the cell's ability to maintain stable internal conditions despite external changes. This balance is essential for cellular function and organismal health. Homeostatic mechanisms involve feedback loops and signaling pathways, which regulate pH, temperature, and ion concentrations.
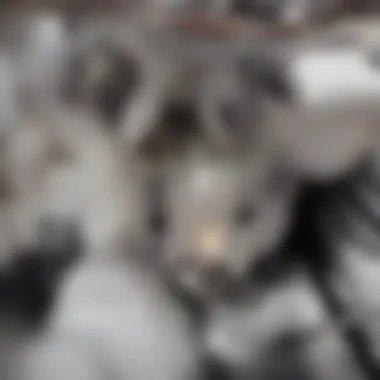
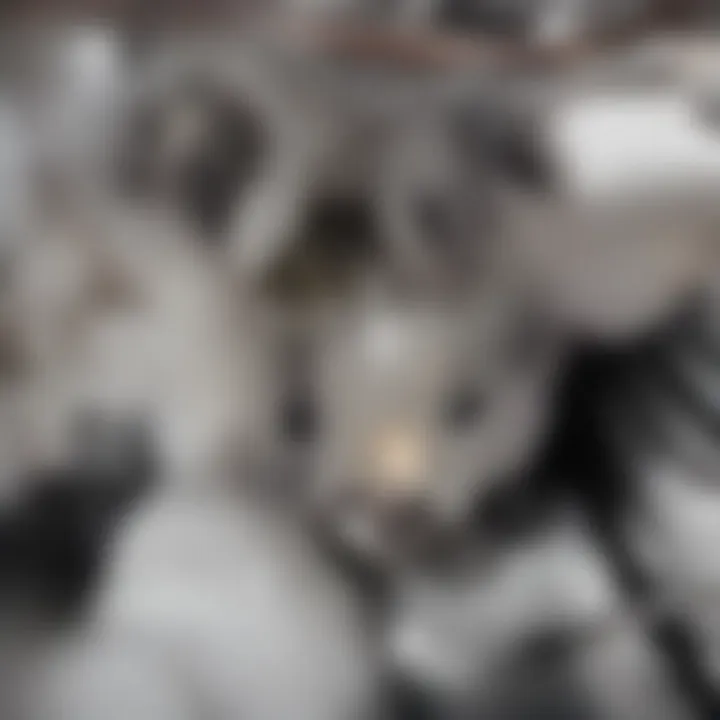
The unique aspect of homeostasis is its reliance on various feedback systems, making it adaptable to different environments. However, its complexity can lead to vulnerabilities, such as in diseases that disrupt homeostatic balance, emphasizing the need for continued research and understanding in this area.
Defining Pro Cellular Concepts
Defining pro cellular concepts is essential to understanding the implications of cellular biology in both scientific research and technology. These concepts include the theoretical underpinnings, practical applications, and interdisciplinary approaches that shape our understanding of how cells operate and interact. Recognizing these elements helps lay the groundwork for advancements in fields like biotechnology and medicine.
Pro Cellular Principles
Theoretical Underpinnings
The theoretical underpinnings related to pro cellular concepts provide a framework for studying cellular mechanisms. This area focuses on fundamental theories that explain processes like signaling, metabolism, and cellular interactions. One key characteristic of these underpinnings is the emphasis on cellular homeostasis. This is a foundational idea that promotes stability within the cell, allowing it to function optimally.
Understanding these principles is beneficial because they enhance our knowledge of biological systems. A unique feature of theoretical underpinnings is their ability to adapt to new findings, thus providing flexibility in interpretation. However, being solely theory-based may limit practical application.
Practical Applications
Practical applications of pro cellular concepts refer to how theoretical knowledge translates into real-world techniques and technologies. For instance, this includes methods like cell culture and genetic manipulation, directly impacting research and medical practices. The deployment of these applications can lead to significant breakthroughs in drug development and disease treatment.
One key characteristic here is the ability to innovate based on foundational principles. This makes practical applications a popular choice among researchers. A unique feature is the capacity to modify existing techniques for specific needs, such as tailoring therapies for individual patients. Nevertheless, practical applications often face challenges, like ethical debates and potential risks involved in manipulation.
Interdisciplinary Approaches
Interdisciplinary approaches within pro cellular concepts highlight the collaboration between various fields, such as biology, chemistry, and engineering. This collaboration allows for a more holistic understanding of cellular behavior and dynamics. One key characteristic of these approaches is their focus on integrating knowledge from different disciplines to address complex problems. This characteristic makes them a beneficial strategy in pro cellular analysis.
The unique feature of interdisciplinary work is the combination of perspectives that can lead to innovative solutions, enhancing both theoretical and practical outcomes. However, this complexity can also complicate communication among specialists, necessitating a balance of expertise across fields.
Historical Context
The historical context of pro cellular concepts reveals how early research has paved the way for current understanding. An examination of this context provides valuable insights into how our current knowledge has developed and the incremental steps that led to today's cellular biology.
Early Research and Discoveries
Early research and discoveries laid the groundwork for the understanding of cellular structures and functions. Scientists like Robert Hooke, who coined the term "cell," marked significant milestones in studying life's basic units. This foundational work is crucial as it defines modern biology's trajectory.
The key characteristic of this research stage is that it encouraged curiosity and exploration, leading to more in-depth studies. This aspect makes early research a vital part of the discussion. Importantly, it provides a timeline of development, showcasing the gradual understanding of cellular life.
However, these early theories often lacked the tools to explore cells at a molecular level, leading to limitations in understanding complex cellular functions fully.
Milestones in Cellular Studies
Milestones in cellular studies refer to key breakthroughs that have significantly advanced knowledge in the field. Discoveries like the cell theory, which states that all living things are made of cells, consolidated previous findings and provided a unifying theory for biology.
The key characteristic of these milestones is their ability to catalyze further research and discovery. They serve as touchpoints that guide future explorations, reinforcing the necessity of a robust theoretical framework. Each milestone often leads to new questions and directions, thus advancing the field.
While these milestones provide a clear progression of knowledge, they sometimes obscure the nuances and complexities of cellular interactions that continue to be explored today.
Evolution of Cell Theory
The evolution of cell theory illustrates how scientific understanding has changed over the years. With contributions from various scientists, the theory has adapted to incorporate new findings. This evolution reflects the dynamic nature of scientific inquiry.
Key characteristics of the evolution of cell theory are its adaptability and ongoing refinement. This aspect is beneficial because it allows for new discoveries without discarding prior knowledge. Moreover, it shows the collaborative nature of scientific progress.
A unique feature of this evolution is the integration of technological advancements, like microscopy, which has continuously expanded our ability to observe cells. However, the iterative nature of evolving theories can lead to confusion if foundational concepts are not solidly understood.
Cell Signaling Mechanisms
Cell signaling mechanisms play a pivotal role in understanding cellular interactions and functions. These mechanisms are essential for cellular communication, helping cells respond to their environment. They facilitate a wide range of biological processes, from immune responses to developmental changes. By delving into the various types of signaling, one can appreciate how cells coordinate and maintain homeostasis within the body.
Understanding signaling is also crucial for advancements in biotechnology and therapeutic interventions. For those engaged in research or clinical applications, recognizing how cells communicate can reveal new pathways for innovative treatments.
Types of Cell Signaling
Autocrine Signaling
Autocrine signaling is characterized by a cell responding to signals that it produces itself. This form of communication allows cells to regulate their own functions. A key characteristic of autocrine signaling is its rapid response time, as the signaling molecules do not need to travel far. This is particularly beneficial in processes such as immune responses, where speed is crucial.
The unique feature of autocrine signaling is its ability to amplify cellular responses. For example, certain immune cells can produce cytokines that enhance their own activity. However, one must consider that this can lead to excessive responses if not properly regulated, which is a disadvantage in some contexts.
Paracrine Signaling
Paracrine signaling involves communication between nearby cells. A distinct aspect of this type is its localized effect, which restricts the signaling molecules' action to neighboring cells. This characteristic is ideal for processes such as tissue repair, where neighboring cells must coordinate to restore function.
Paracrine signaling is popular in developmental biology, as it helps in organizing developing tissues. However, the short range of signal diffusion can limit the impact on distant target cells, potentially requiring additional signaling methods to affect broader areas.
Endocrine Signaling
Endocrine signaling relies on hormones released into the bloodstream. This significantly broadens the scope of cellular communication, allowing signals to reach distant targets. The key characteristic of endocrine signaling is its ability to coordinate complex processes that involve multiple organs, such as growth and metabolism regulation.
A notable advantage of endocrine signaling is its longevity. Hormones can remain active in the bloodstream for extended periods. However, the complexity involved in endocrine responses can lead to delayed effects, which might not be suitable for immediate responses needed in acute situations.
Importance of Signal Transduction
Cellular Responses
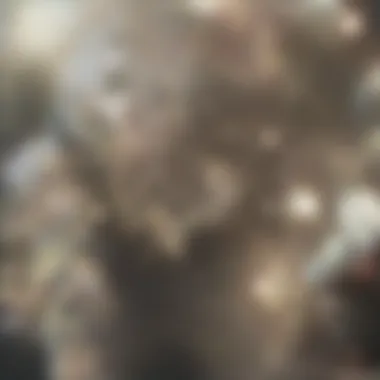
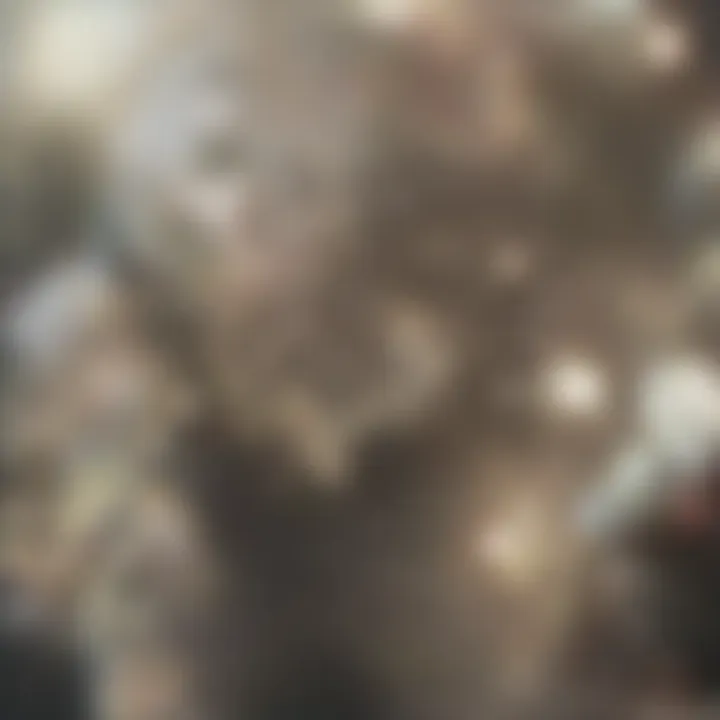
Cellular responses are critical outcomes of signal transduction processes. They dictate how cells adapt to signals from their environment. A key characteristic of cellular responses is their specificity; cells respond uniquely to different signals based on their receptors. This specificity allows for finely tuned biological responses that are tailored to the needs of each cell type.
In the context of this article, understanding cellular responses is beneficial as it highlights the complexities of cell behavior. It also showcases how dysregulation in these responses can lead to diseases, making it a vital focus area in research.
Signaling Pathways
Signaling pathways are intricate networks that relay signals from the cell surface to specific internal targets. A notable feature of these pathways is their modular nature, allowing for various combinations and interactions. This characteristic enables cells to integrate multiple signals, facilitating complex responses essential for maintaining homeostasis.
Studying signaling pathways is key to understanding diseases, as many conditions arise from pathway disruptions. Knowledge in this area can thus contribute to more effective therapeutic strategies.
Impact on Cellular Function
The impact of signal transduction on cellular function is profound. It governs processes such as cell growth, differentiation, and apoptosis. A key characteristic here is the interdependence of signaling pathways and cellular functions; changes in signaling can drastically alter cell behavior.
Recognizing this impact helps contextualize the importance of signaling mechanisms in this article. It assists researchers in identifying potential therapeutic targets to manipulate cellular processes effectively, providing a deeper understanding of cellular dynamics.
Advancements in Biotechnology
Biotechnology is increasingly significant in the field of science and medicine. It encompasses a range of techniques and methods that utilize biological systems for the development of products and technologies. The advancements in this domain profoundly influence several sectors, including healthcare, agriculture, and environmental conservation. This section delves into key areas of biotechnology that are reshaping our understanding and interaction with living organisms.
Genetic Engineering
CRISPR Technology
CRISPR (Clustered Regularly Interspaced Short Palindromic Repeats) technology represents a groundbreaking method in genetic engineering. It allows researchers to edit genes with unprecedented precision and efficiency. The key characteristic of CRISPR is its ability to target specific DNA sequences and alter them, enabling the modification of organisms at a genetic level. This precision makes CRISPR a popular choice in various applications, including agricultural modification and disease research.
One unique feature of CRISPR is its ease of use compared to traditional genetic modification techniques. The system requires fewer resources and less time to achieve results. However, it is not without drawbacks. Ethical considerations and potential off-target effects present risks that are yet to be fully understood. These issues need to be carefully evaluated as the technology advances.
Gene Therapy Techniques
Gene therapy techniques focus on treating or preventing disease by inserting genes into patients' cells. This method is significant because it offers a potential cure for genetic disorders, rather than merely managing symptoms. Techniques often involve the delivery of therapeutic genes using vectors, commonly derived from viruses.
A key feature of gene therapy is its ability to directly tackle the root cause of genetic diseases. This approach can yield a long-lasting effect, potentially eliminating the need for continuous treatment. However, challenges arise in ensuring the safe and effective delivery of these genes. There are also questions concerning the long-term effects on patients' health, making ongoing research critical.
Risks and Benefits
The risks and benefits associated with advancements in biotechnology are multifaceted. While the potential to eradicate genetic diseases and enhance agricultural productivity is tremendous, there are inherent dangers. One of the primary benefits includes the possibility of drastically reducing or eliminating hereditary diseases through technologies such as CRISPR and gene therapy.
However, the key characteristic of these advancements is the ethical implications involved. Public perception plays a significant role in shaping the acceptance of these technologies. The potential for genetic modification raises questions about bioethics, making it crucial to navigate the legal and social ramifications. Balancing innovation with caution will be essential for progressing responsibly in this field.
Cellular Therapies
Stem Cell Research
Stem cell research holds promise for understanding and treating a variety of conditions. Stem cells have the unique ability to differentiate into various cell types, making them crucial for regenerative medicine. The key characteristic of stem cell research is its potential to replace damaged cells, offering hope for conditions previously deemed untreatable, such as spinal cord injuries and neurodegenerative diseases.
One unique aspect of stem cell research is its versatility. Researchers can use stem cells from various sources, including adult tissues and embryos. However, this versatility also leads to ethical debates regarding the use of embryonic stem cells. These ethical concerns can hinder funding and public support, which are critical for advancing research.
Regenerative Medicine
Regenerative medicine is a subset of biotechnology that aims to repair or replace damaged tissues and organs. This field often overlaps with stem cell research and focuses on harnessing the body's own repair mechanisms. A significant characteristic of regenerative medicine lies in its capability to restore function, which is revolutionary for healthcare.
Further, regenerative medicine poses the unique feature of personalized treatment. By utilizing a patient's own cells, the risk of rejection is minimized. However, there remain challenges in ensuring consistent and effective results. Regulatory hurdles and the need for extensive clinical trials are ongoing obstacles that researchers must address.
Challenges in Treatment
Despite considerable advancements, challenges remain in the practical application of cellular therapies. Key characteristics include complexity in understanding cellular behavior and the interplay between different cell types. Such complexities can affect the outcomes of treatments, necessitating robust research to validate approaches.
A unique aspect of these challenges is the disparity in access to cellular therapies. While progress in biotech offers transformative possibilities, not everyone has equal access to such advancements. Disparities in healthcare can lead to significant inequalities, which must be addressed to ensure equitable healthcare solutions. The future of biotech will rely heavily on balancing innovation with social responsibility.
Ethical Implications
The exploration of ethical implications in cellular research is essential. This section delves into how genetic manipulation affects not only biology but also society at large. The encounter with ethics provides a framework for assessing practices in genetics and biotechnology. Understanding these elements is crucial for responsible scientific progress.
Ethics in Genetic Manipulation
Legal Frameworks
Legal frameworks govern genetic manipulation, ensuring it is conducted within ethical guidelines. They provide a set of rules that researchers must follow. The key characteristic of these frameworks is their ability to enforce accountability. They are beneficial in preventing misuse of genetic technologies. An important feature is that they often evolve as technology progresses, allowing adaptation to new discoveries.
However, these frameworks can also face limitations in transparency and public engagement. It makes compliance hard for researchers who might not fully understand them.
Public Perception
Public perception plays a significant role in genetic research. It shapes policies and funding for studies. A key characteristic here is that perception is often influenced by media coverage and personal experiences. This makes it a valid choice for this article, as understanding public sentiment is crucial. Negative perceptions can hinder research and reduce funding.
It is unique in how it reflects societal values and moral beliefs. Because of this, researchers must actively communicate the benefits of their work to the public. *In some cases, misinformation increases resistance, making public engagement even more necessary.*
Consequences of Manipulation
Examining the consequences of genetic manipulation is vital for informed decision-making. The key characteristic is the long-lasting impact on health and environments. Understanding these consequences is important to avoid unintended fallout.
The unique feature of this analysis lies in its dual approach. It considers both potential benefits, like disease elimination, and risks, like ecological disruptions. Striking a balance between innovation and caution is essential for ethical practice.
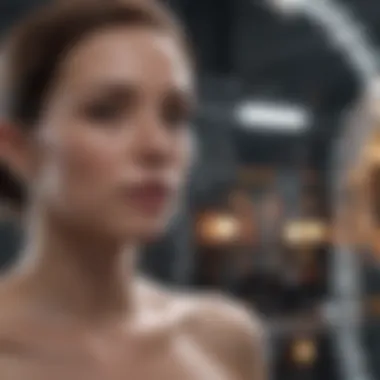
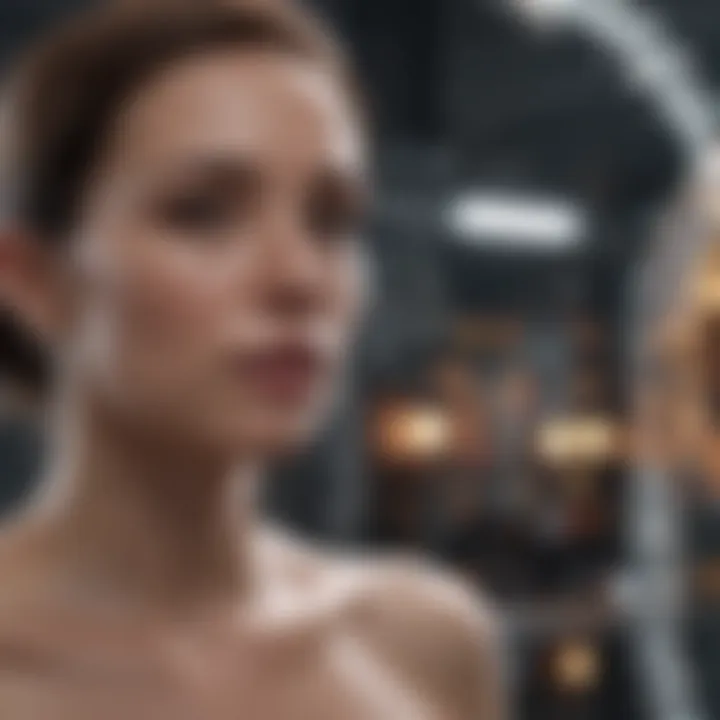
Societal Impact of Cell Research
Access to Technology
Access to technology in cellular research significantly influences its outcomes. This aspect shapes who can benefit from advancements in the field. A key characteristic is the disparity in resources available to different regions and communities. This makes it pertinent to discuss in the context of this article. Access empowers researchers and practitioners to apply findings effectively.
However, limitations in access can create unequal benefits, often siding with wealthier nations or organizations. This leads to a skewed representation of advancements in cellular science.
Healthcare Disparities
Healthcare disparities highlight variations in health access and quality. They are critical when discussing the impacts of cellular research. A key facet here is social determinants that contribute to these disparities. Programs focusing on mitigating these differences can be added as beneficial components of awareness and education.
The unique feature of addressing these disparities in this article is its focus on sustainable solutions. Such approaches can lead to greater equity in health outcomes.
Future Generations
The future generations aspect raises questions about the legacy of current research practices. It highlights the long-term effects of cellular manipulation on health and society. A key characteristic is the responsibility to ensure safety and efficacy for future people. It is a relevant topic in this article as current decisions shape future realities.
This feature's uniqueness lies in its far-reaching implications. Efforts today can either burden or benefit the generations that follow. Thus, caution and informed choices are necessary when engaging in cellular manipulations.
Future-focused research must keep ethical considerations at the forefront to cultivate a responsible scientific community.
Current Research Trends
Current research in cellular studies expands the understanding of cellular processes and their interactions within broader biological systems. This exploration includes how cells communicate with their environment and how neighboring cells influence their functions. Continuous investigation into these current trends contributes significantly to the fields of medicine and biotechnology.
Cellular Microenvironment Studies
Role of Extracellular Matrix
The extracellular matrix (ECM) plays a crucial role in maintaining cell structure and function. It provides a scaffold for cells, facilitating their attachment and proper orientation. Understanding ECM's characteristics is essential because it affects cell differentiation and behavior. A key aspect of ECM is its dynamic nature, which allows for remodeling in response to various stimuli. This adaptability enhances its appeal in researching cell behavior and therapies. Nevertheless, one limitation of ECM studies is the variability in composition among different tissue types, which can complicate analysis.
Influence on Cell Behavior
Cell behavior is significantly influenced by the microenvironment, including the ECM. The interactions between cells and their surrounding extracellular components mold how cells respond to signals. A vital characteristic of this influence is how it can determine cell fate, promoting differentiation or activation. This understanding is beneficial for applications in regenerative medicine and cancer treatments. However, capturing the exact parameters of this influence can be challenging due to the complexity of biological systems.
Applications in Cancer Research
The study of cellular microenvironments directly impacts cancer research. By examining how tumor cells interact with surrounding cells and ECM, researchers can uncover mechanisms behind cancer progression and metastasis. A notable characteristic of these applications is their potential to lead to targeted therapies that disrupt specific interactions. This focus is beneficial as it allows for personalized medicine approaches. Yet, developing these applications can be resource-intensive and may require extensive validation to ensure effectiveness.
Interactions with Other Biological Systems
Immune Responses
Interactions between cells and the immune system are foundational in understanding disease processes. Immune responses can be triggered by cellular signals, affecting how the body responds to pathogens. A defining characteristic of immune responses is their adaptability, which allows them to cope with various challenges. This feature makes studying these interactions important for vaccine development and immunotherapy techniques. On the downside, dysregulation of immune responses can lead to autoimmune diseases, necessitating a more nuanced understanding of these interactions.
Nervous System Connections
Cellular interactions extend into the nervous system, where communication facilitates reflexes and complex behaviors. The connections between nerve cells and other types of cells are essential for maintaining homeostasis and responding to external stimuli. A key characteristic of these connections is their speed and specificity in transmitting signals, which is critical for rapid responses. Research in this area is beneficial, but it also faces challenges in isolating specific pathways for detailed study.
Impact on Overall Health
The overall health of an organism is profoundly influenced by cellular interactions and the cellular microenvironment. Factors such as inflammation, stress, and cellular signaling can dramatically affect health outcomes. A key characteristic of this impact is the cumulative nature of cellular responses leading to systemic effects. Thus, understanding these interactions is beneficial for designing therapeutic strategies. However, untangling these complex interactions often proves difficult, requiring interdisciplinary collaboration and advanced methodologies.
Understanding current research trends provides valuable insight into the complexity of cellular dynamics and their implications for health and disease.
Future Directions in Cellular Research
Understanding future directions in cellular research is crucial for the advancement of biological sciences and related technologies. As we look ahead, innovations in this field can lead to breakthroughs in medical treatments, environmental science, and biotechnology. The intersection of traditional cellular biology with new technologies is creating exciting opportunities.
Innovations in Cellular Observation
Imaging Techniques
The use of advanced imaging techniques has transformed our ability to study cells. High-resolution microscopy, such as confocal and super-resolution microscopy, allows researchers to observe cellular structures in more detail than ever before. These techniques are beneficial because they provide clarity and precision in visualizing cellular processes. A unique feature is their ability to capture live cell behavior in real-time, which enables scientists to witness dynamic changes and interactions within the cell. However, these methods can be expensive and require specialized training, which may limit accessibility.
Data Analysis Tools
Data analysis tools are vital in managing the vast amounts of information generated from cellular studies. Sophisticated software allows scientists to process and interpret data efficiently, contributing to clearer insights. The key characteristic of these tools is their capability to handle complex datasets quickly, making it easier for researchers to draw meaningful conclusions. A unique feature is the implementation of machine learning algorithms, which enhance data interpretation but can also introduce variability. The reliance on automated tools may lead to an overdependence that can complicate traditional analytical methods.
Real-Time Monitoring
Real-time monitoring of cellular activities provides a continuous insight into cellular dynamics. Techniques like fluorescence microscopy facilitate this process, allowing observation of cellular responses to stimuli almost instantaneously. This is a popular choice because it offers immediate feedback during experiments, aiding in hypothesis testing. The unique feature of this approach lies in its ability to capture transient events that might be missed in static observations. On the downside, real-time monitoring can be limited by photobleaching and potential damage to the cells being studied, which raises concerns about the sustainability of experimental conditions.
Predicted Advancements
Integration of AI
The integration of artificial intelligence in cellular research is beginning to shape the landscape of experimental design and data interpretation. Machine learning algorithms could analyze patterns in complex datasets far more efficiently than traditional methods. This choice is beneficial for enhancing the accuracy of predictive modeling in cell biology. A unique feature of this integration is its ability to discover new biological insights hidden in large data pools. However, the potential for biases in AI-driven analysis remains a concern, as it may affect research outcomes if data input is not carefully managed.
Personalized Medicine
Personalized medicine represents a significant advancement in treating diseases based on individual cellular makeup. This approach tailors treatment plans to the unique genetic profile of each patient, potentially leading to more effective care. Its key characteristic is the focus on molecular diagnostics, which helps in understanding patients’ responses to therapies. As a unique feature, it holds a promise of reducing trial-and-error in treatment protocols. Yet, challenges remain regarding accessibility and the ethical implications of genetic information, which could create disparities in healthcare.
Global Collaboration in Research
Global collaboration has become essential in conducting cellular research on a broader scale. Scientists across different countries are pooling resources and knowledge to tackle complex questions facing humanity. The key characteristic of this collaboration lies in its ability to merge diverse expertise, which enhances the quality of research outcomes. A unique feature is the establishment of international research networks, facilitating knowledge exchange. Nonetheless, navigating logistics and ensuring equitable partnerships can sometimes be challenging, complicating efforts toward common goals.
"The future of cellular research depends on how effectively we can leverage technology and collaboration across the globe."