Understanding qPCR Efficiency Formula in Molecular Biology
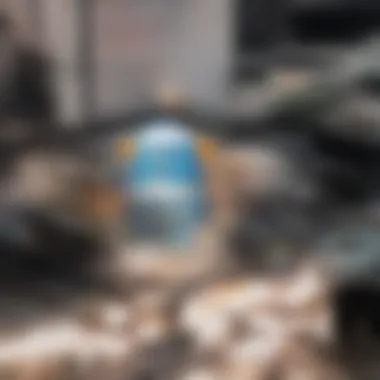
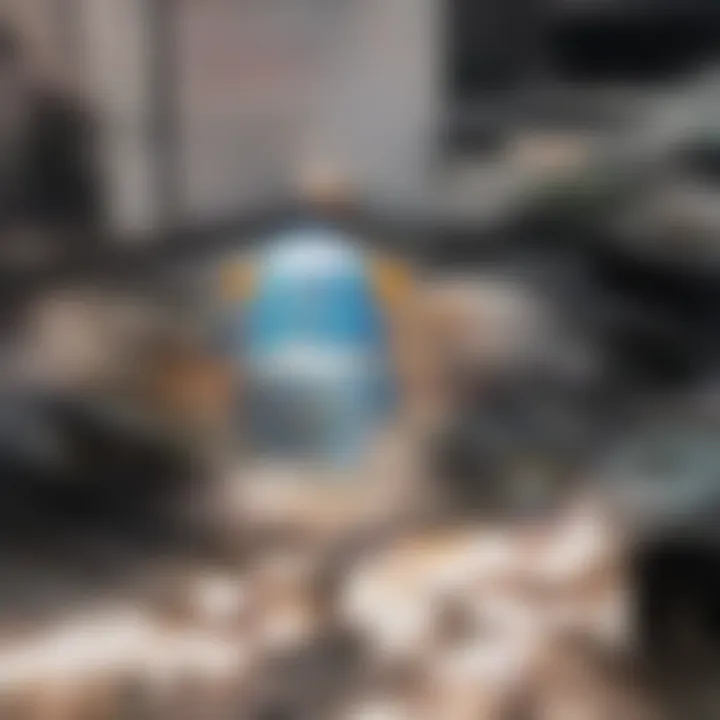
Intro
Quantitative Polymerase Chain Reaction (qPCR) is essential for anyone in molecular biology. This method enables researchers to measure DNA and RNA targets accurately, presenting real-time data that can be analyzed instantly. One crucial aspect is the qPCR efficiency formula, which assesses how effectively the amplification occurs. Understanding this formula is not merely academic; it carries significant implications for experimental design and data interpretation.
Research Overview
Summary of Key Findings
Throughout numerous studies, it has been shown that qPCR efficiency plays a pivotal role in obtaining reliable results. Typically, an efficiency range between 90% to 110% is considered acceptable, indicating that each cycle roughly doubles the amount of DNA. Variances outside this range can lead to misleading conclusions about the quantity of the original target.
Background and Context
The concept of qPCR efficiency dates back to the evolution of PCR itself. Traditional PCR provided qualitative results, but with advancements in technology, quantitative measurements became feasible. Many factors influence the efficiency, including primer design, template integrity, and the specific reagents used during the process. Each of these factors must be scrutinized to ensure experimental reliability.
Methodology
Experimental Design
A well-structured experimental design is vital for accurate qPCR results. Researchers often employ a standard curve method, where they prepare serial dilutions of known concentrations. This enables them to establish a reference for efficiency calculations.
Data Collection Techniques
Data collection in qPCR involves monitoring the fluorescence signal during the amplification process. This is typically accomplished through specialized software that captures the data in real time. As the PCR progresses, a graph is generated, facilitating the analysis of the amplification plot. It is this plot that forms the basis for calculating efficiency using the slope of the standard curve.
"The efficiency of qPCR is critical for interpreting results accurately. Even slight deviations can lead to significant misinterpretations of the biological significance."
Foreword to qPCR
Quantitative Polymerase Chain Reaction, known as qPCR, is an essential technique in molecular biology. It provides researchers with the ability to quantify the amount of specific DNA or RNA in a sample. By converting these nucleic acids into measurable data, qPCR has transformed our approach to genetics, diagnostics, and research. This introduction will elaborate on the significance of qPCR and outline the core components of this technique.
Overview of Quantitative PCR
Quantitative PCR involves amplifying DNA or RNA while simultaneously measuring the accumulation of the target nucleic acid. Unlike conventional PCR, which only confirms the presence of genetic material, qPCR quantifies it in real-time. This method typically employs fluorescent dyes, which bind to the double-stranded DNA produced during amplification. As the cycles of amplification proceed, the increase in fluorescence correlates to the amount of target nucleic acid present in the sample.
Several platforms exist for performing qPCR, including Applied Biosystems and Bio-Rad systems, each offering unique features tailored to specific research needs. The amplification process allows for precise quantification, making it indispensable in many fields, such as clinical diagnostics and research.
Importance of qPCR in Molecular Biology
The relevance of qPCR in molecular biology cannot be understated. Below are some key aspects that highlight its significance:
- High Sensitivity: qPCR can detect low amounts of nucleic acids, which is crucial in early disease detection, such as cancer or infections.
- Specificity: This technique can differentiate closely related sequences, which is vital for studying genetic variations.
- Versatility: It is applicable in various research areas, from gene expression studies to forensic analysis.
"qPCR is a versatile tool that enables researchers to not only detect but also quantify genetic material accurately."
- Data Output: The method provides quantitative data, which supports more informed decision-making in experiments and therapeutic interventions.
As we analyze the intricacies of qPCR, it becomes clear that the efficiency of this technique plays a critical role in the results obtained. Understanding the efficiency formula and its components will equip researchers with the knowledge to optimize their experiments, ensuring accuracy and effectiveness in their studies.
Defining Efficiency in qPCR
Defining efficiency in qPCR is essential for understanding how well the amplification of nucleic acid sequences occurs during the PCR process. The efficiency of a qPCR reaction can greatly influence the quantitative results obtained from experiments. High efficiency indicates effective amplification, while low efficiency can point to issues in the PCR setup.
Understanding efficiency is significant for multiple reasons. First, it helps in evaluating the reliability of the results. Second, knowing the efficiency allows researchers to make necessary adjustments to improve performance. Lastly, it supports the intercomparison of different experiments, making it easier to share and validate findings.
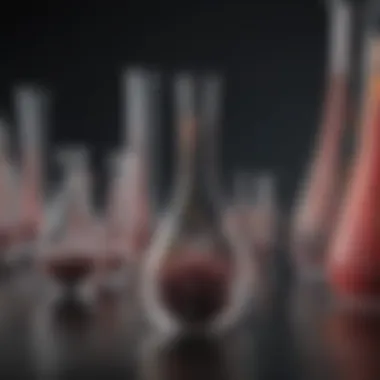
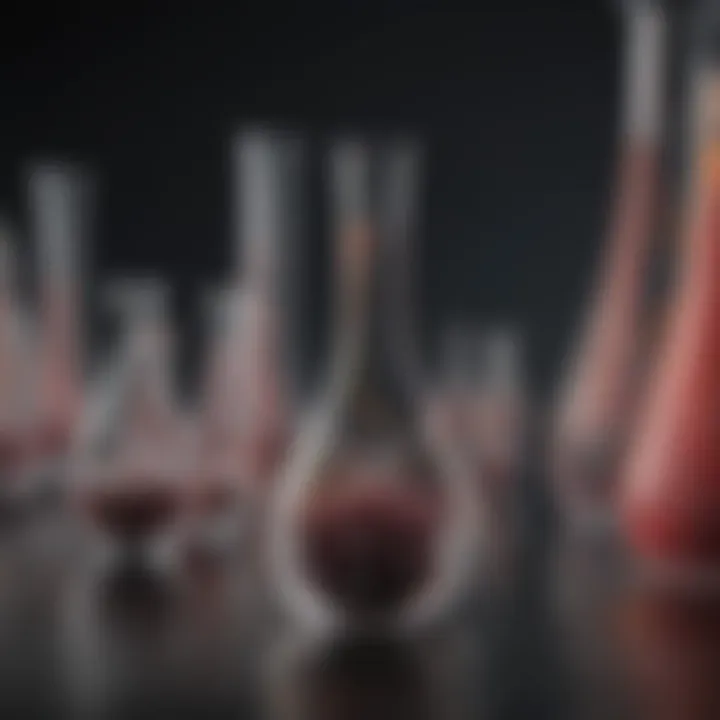
What is Efficiency?
Efficiency in qPCR is defined as the ability of the PCR process to amplify a target sequence. It is usually expressed as a percentage. A perfectly efficient reaction reaches 100%, meaning every cycle doubles the amount of targeted DNA. However, in practical scenarios, efficiencies typically range from 90% to 110% for optimal reactions. This value is calculated based on the slope of the standard curve generated from a serial dilution of known quantities of nucleic acid.
The efficiency can be influenced by various factors including primer design, template quality, and reaction conditions. For example, poorly designed primers can cause non-specific amplifications, leading to lower efficiency.
Interpretation of Efficiency Values
Interpreting efficiency values is critical for assessing the quality and reliability of qPCR results.
- 100% Efficiency: This indicates that the PCR reaction produces a perfect exponential amplification of the template.
- Below 90% Efficiency: Values below this threshold can suggest problems, like suboptimal conditions, reagents that are not functioning properly, or template degradation. These issues can mislead data interpretation.
- Above 110% Efficiency: Values that exceed this threshold can indicate artifacts in amplification, possibly due to primer-dimer formations or contamination, resulting in inflated readings.
Researchers are often tasked with ensuring optimal efficiency. By closely monitoring reaction conditions and making necessary adjustments, they can enhance the accuracy of their quantitative assessments in molecular biology experiments.
Efficiency in qPCR plays a pivotal role in the reliability of experimental data, emphasizing the need for rigorous evaluation during every phase of the PCR process.
The qPCR Efficiency Formula
Understanding the qPCR efficiency formula is essential for anyone working in molecular biology. This formula provides a quantitative measure of how effectively the PCR process amplifies the target nucleic acid. For researchers, knowing the efficiency can dictate the success of an experiment. An efficient qPCR reaction can lead to reliable data, while inefficient reactions may result in faulty conclusions.
The qPCR efficiency formula is fundamentally defined as the rate at which DNA is amplified during each cycle of the PCR. It allows the scientist to determine whether the reaction conditions are optimal. This method plays a critical role when quantifying specific genes in various samples. Furthermore, high efficiency is often required for detecting low abundant targets, making the formula a key aspect of experimental design in qPCR.
Optimizing this efficiency can enhance the validity of quantitative results considerably. Therefore, a clear understanding of the formula and its implications cannot be overlooked. Researchers often need this knowledge to interpret data accurately, leading to robust scientific findings that could advance their studies.
Mathematical Representation
In qPCR, the mathematical expression for efficiency is often represented as:
Where:
- E represents the efficiency of the PCR reaction.
- slope refers to the slope of the standard curve obtained from plotting the log of the starting quantity of template versus the threshold cycle (CT) values.
This formula illustrates how the efficiency relates to the slope of the standard curve. A slope of -3.32 corresponds to 100% efficiency, meaning the amount of product doubles with each cycle. Conversely, slopes steeper than this indicate lower efficiency, signaling potential issues in the reaction.
Components of the Formula
The components within the qPCR efficiency formula provide deeper insights into the factors affecting PCR performance. The following elements are crucial:
- Threshold Cycle (CT): This is the cycle number at which the fluorescence of the PCR product crosses a specific threshold. It is directly related to the initial amount of DNA.
- Standard Curve: Generated from known concentrations of DNA, the standard curve helps in determining the slope, which in turn influences the efficiency value.
- Slope: The slope obtained from the standard curve reflects how well the reaction is working. A shallower slope indicates higher amplification and thus higher efficiency.
In summary, each component of the qPCR efficiency formula interconnects to provide a comprehensive analysis of amplification performance. Understanding these components allows researchers to troubleshoot and optimize qPCR experiments effectively.
Calculating qPCR Efficiency
Calculating qPCR efficiency is crucial in understanding the accuracy and reliability of quantitative PCR experiments. Efficiency not only indicates how well the reaction amplifies the target DNA or RNA but also shapes the interpretations of experimental data. Inaccurate efficiency calculations can lead to flawed conclusions in research and diagnostics. Thus, mastering this calculation is essential for any researcher or student in molecular biology.
Sample Data Collection
Before proceeding with any calculations, accurate sample data collection is paramount. This entails gathering real-time PCR data which includes threshold cycle (Cq) values from a dilution series of your target nucleic acid. The quality of your data greatly influences the outcome and reliability of your efficiency calculations. Here are key points for effective data collection:
- Consistency in Sample Handling: Same procedures must be followed for preparing each dilution.
- Use Appropriate Dilution Factors: A good range is usually between 10-fold to 5-fold dilutions to encompass a wide array of target concentrations.
- Replicates are Essential: Collect data in biological and technical replicates to account for variation. It helps increase the reliability of the results.
- Inclusion of Controls: Always include no-template controls and positive controls to ensure the reaction conditions are optimum and the reagents are functioning properly.
Step-by-Step Calculation Process
Determining qPCR efficiency involves a clear, step-by-step approach. Here’s how to do it effectively:
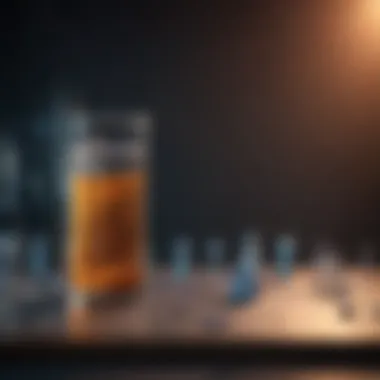
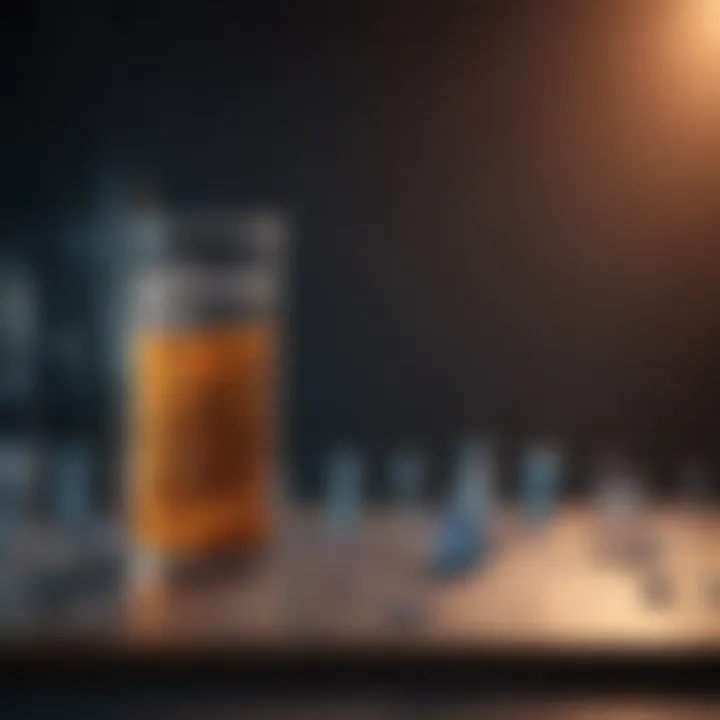
- Plot your Data: Create a standard curve using the Cq values obtained from your dilution series. Graph the log of the initial template concentration against the Cq values.
- Determine the Slope (m): Calculate the slope of the linear regression line. The slope will reflect the efficiency of the PCR amplifications.
- Apply the Efficiency Formula: Utilize the following equation :Efficiency (%) = (10^(-1/slope) - 1) * 100Where the slope is derived from the graph of your collected data.
- Interpret your Results: Efficiency values usually range between 90%-110%. Values lower than this suggest potential problems with your reaction or sample quality.
- Document Findings: Recording your calculations and results helps in future reference and publication. Data consistency and detailed methodology improve reproducibility in scientific work.
Accurate calculations in qPCR are more than just numbers; they carry implications for biological understanding and discoveries.
Factors Affecting qPCR Efficiency
The efficiency of qPCR is influenced by various factors that must be thoroughly understood to optimize results. In this section, we will explore these critical elements, outlining their importance and how they form the backbone of effective qPCR performance. By understanding these factors, researchers can improve the accuracy and reliability of their data.
Template Quality and Quantity
Template quality and quantity are fundamental for successful qPCR experiments. High-quality templates ensure that the DNA or RNA you are amplifying is intact and free from contaminants. Contaminants can inhibit the polymerase enzyme, leading to reduced amplification and unreliable data.
Moreover, the quantity of template present is equally important. A too low template concentration may lead to stochastic effects, where the results can vary significantly between replicates. Conversely, too high a concentration can cause amplification efficiency issues due to saturation, where the reaction cannot proceed optimally due to excess template.
To elaborate, it’s crucial to measure the concentration accurately, using methods such as spectrophotometry or fluorometry. These methods will give you a more precise idea of the amount of nucleic acid present, allowing you to adjust concentrations accordingly. Ultimately, maintaining high-quality and optimal quantity is imperative.
Reagent Components
The success of a qPCR experiment relies heavily on the reagent components. Key reagents include polymerase, primers, dNTPs, and buffers. Each of these plays a specialized role in the amplification process.
- Polymerase: The enzyme responsible for DNA synthesis must be of high fidelity and heat-stable, such as Taq polymerase, to withstand the thermal cycling. The efficiency of this enzyme can directly impact how well the DNA is amplified.
- Primers: Primers should be designed to bind specifically to the target sequence, facilitating accurate amplification. Inappropriate primer design can lead to non-specific amplification or primer-dimer formations.
- dNTPs: These nucleotides must be supplied in adequate amounts since they are the building blocks for new DNA. An imbalance in dNTPs can diminish the efficiency of the amplification.
In summary, every component’s quality and concentration should be carefully considered during the planning phase of your experiment.
Thermal Cycling Conditions
Thermal cycling conditions dictate the efficiency and specificity of qPCR reactions. The accurate adjustment of temperature for denaturation, annealing, and extension phases can reveal profound similarities.
- Denaturation Temperature: Typically set at 95°C, this temperature must be sufficient to separate the DNA strands effectively. If the temperature is not high enough, the strands may not denature fully, hindering the reaction.
- Annealing Temperature: This should be optimized based on primer sequences and the melting temperatures of the primers. If the annealing temperature is too low, primers might bind non-specifically to other sequences, while a too-high temperature might prevent proper binding.
- Extension Time: This is often calculated based on the length of the target DNA fragment. Longer extensions allow for accurate synthesis of larger sequences, but timing must also consider the polymerase’s activity rate.
A proper balance in these conditions enhances the efficiency of the qPCR process, ensuring that the outcomes are not only quantitative but also reproducible.
The interplay between template quality, reagent components, and thermal cycling conditions significantly shapes the overall efficiency of qPCR. By meticulously managing these aspects, researchers can optimize their protocols and achieve clearer insights from their studies.
Common Errors in Efficiency Measurement
In the realm of quantitative PCR, understanding efficiency is paramount, given its critical role in data accuracy and reliability. Errors in measuring efficiency can lead to misleading conclusions, impacting experimental outcomes. Identifying and addressing these errors is essential for researchers to enhance both the validation of their results and the credibility of their methodologies.
Amplification Errors
Amplification errors can significantly skew the perceived effectiveness of a qPCR experiment. These errors may arise from various sources during the PCR process, leading to inaccurate estimation of gene expression or target DNA levels. Some common causes include:
- Inefficient enzyme activity: If the DNA polymerase used in the reaction has suboptimal performance, it may fail to adequately amplify the target, thus yielding lower efficiencies.
- Suboptimal primer design: Primers that do not bind well to the target sequence, or that form dimers, can limit amplification.
- Inhibitors in the reaction mix: The presence of contaminants like proteins or phenol can inhibit the action of the DNA polymerase, adversely affecting the amplification process.
Each of these errors can result in distorted efficiency values, making it crucial for researchers to regularly evaluate their amplification cycle parameters and the quality of reagents employed.
"Consistent monitoring of amplification efficiency is vital to ensure the integrity of qPCR data"
Data Interpretation Mistakes
Data interpretation mistakes often stem from misinterpretation of qPCR results. These errors can lead to overestimation or underestimation of target quantities. Some common pitfalls include:
- Failing to normalize data: Neglecting to use a reference gene for normalization can result in misleading quantification of the target. Proper housekeeping genes must be identified and used.
- Inadequate understanding of the threshold cycle (Ct) values: Misunderstanding how to properly set thresholds can lead to incorrect efficiency calculations. Accurate baseline settings are vital for proper data analysis.
- Ignoring variability in replicates: Not accounting for biological or technical replicates may yield an inaccurate picture of data reproducibility and reliability.
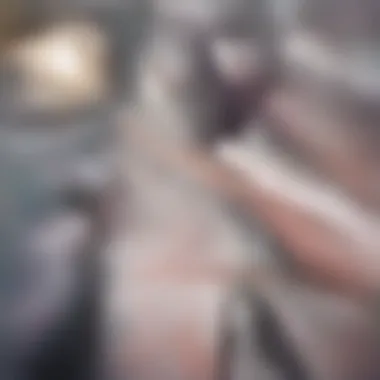
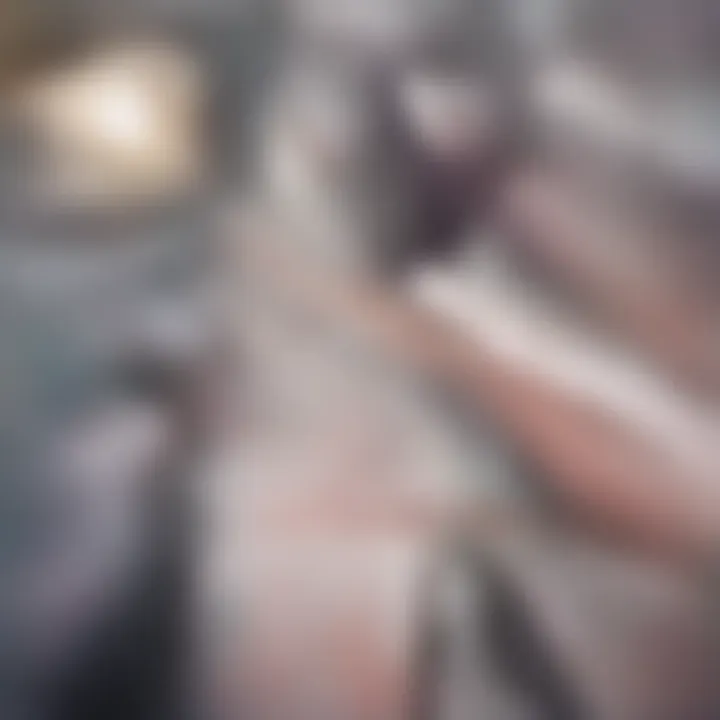
Addressing these interpretation mistakes helps to minimize variance and improve confidence in the results obtained. A meticulous approach to both analysis and data handling is necessary to ensure reliable outcomes.
Optimizing qPCR Efficiency
Optimizing qPCR efficiency is crucial for achieving reliable and accurate results in quantitative PCR experiments. Efficiency affects the fidelity of amplification, which, in turn, influences the interpretations of experiments aimed at quantifying nucleic acids. A high efficiency value signifies that the PCR conditions are conducive for robust amplification, leading to reliable data. Thus, understanding how to optimize efficiency not only enhances experimental outcomes but also allows for better comparisons across different experiments.
Several elements factor into the optimization of qPCR efficiency. These range from sample preparation to the choice of primers and the careful consideration of thermal cycling conditions. Implementing best practices in these areas can lead to significant improvements in amplification efficiency and thus improve the quality of experimental results. Here are some key areas to focus on:
- Cost-effectiveness: Optimizing qPCR conditions can reduce the need for repeated experiments, ultimately saving time and resources.
- Reproducibility: Consistent qPCR results aid in comparing data across studies and experiments.
- Data integrity: Higher efficiency leads to more accurate quantitative results, facilitating reliable scientific conclusions.
Best Practices for Sample Preparation
Sample preparation is a foundational step in qPCR workflows. Proper techniques here can directly impact reaction efficiency. It is vital to start with high-quality, intact RNA or DNA. Contaminants such as proteins, phenol, or salts can hinder enzyme activity, negatively affecting the reaction. Here are some considerations:
- Use Fresh Samples: Whenever possible, utilize fresh biological samples. Biologics such as blood or tissue can degrade over time, altering the nucleic acid integrity.
- Optimize Extraction Protocols: Consider using kits or methods known for high yield and purity. For instance, the Qiagen DNeasy or RNeasy kits are popular for their efficiency.
- Assess Concentration: Quantify your nucleic acids accurately. Too much or too little can drastically skew results. Utilize tools like spectrophotometers or fluorescence-based assays for precise measurements.
Choosing Appropriate Primers
Primers play a crucial role in the specificity and efficiency of the qPCR reaction. Their design should minimize non-specific binding while ensuring robust amplification of the target sequence. Here are key considerations when selecting primers:
- Length and GC Content: Primers are typically 18-25 nucleotides long with a GC content around 40-60%. This balance aids in forming stable and specific primer-template complexes.
- Specificity Testing: Utilize software to predict secondary structures and potential off-target binding. Tools such as Primer3 can assist in designing specific primers.
- Avoid Hairpin Structures: Hairpin formation can reduce the availability of primers for target binding, leading to lower efficiency.
- Evaluate Efficiency: Assess the efficiency of the primers through preliminary experiments. A reaction efficiency between 90-110% is optimal for qPCR.
Proper selection and optimization of primers can dramatically enhance the quality of your qPCR results. It is essential to invest time in this phase to avoid complications in downstream analysis.
Applications of qPCR Efficiency
Quantitative Polymerase Chain Reaction (qPCR) efficiency has a pivotal role in various scientific fields. By understanding and applying qPCR efficiency, researchers and clinicians gain the ability to make informed decisions regarding experimental designs, diagnostics, and therapeutic strategies. This section discusses the importance, benefits, and considerations linked to the applications of qPCR efficiency.
Clinical Diagnostics
The use of qPCR efficiency in clinical diagnostics can greatly enhance patient care. It enables the precise measurement of nucleic acids, making it possible to detect pathogens accurately and rapidly. In infectious disease detection, for instance, qPCR can identify viral or bacterial genomes at low concentrations, allowing for early diagnosis and treatment.
- Accurate Quantification: By calculating the efficiency of each assay, clinicians can trust the reliability of results, leading to better-informed treatment decisions.
- Monitoring Disease Progression: In cases like oncology, measuring the efficiency of qPCR can help assess tumor load in real-time, guiding therapeutic interventions.
- Genetic Testing: In genetic disorders, qPCR efficiency provides clarity on the presence of mutations or alleles, informing potential interventions or preventive measures.
Opting for qPCR involves being aware of inherent challenges. Variability in sample quality, primer design, and reagent quality can impact efficiency. Clinicians must remain vigilant for such factors to maintain the integrity of diagnostic results.
"qPCR is a transformative technology in diagnostics, giving health care providers an edge in managing diseases effectively."
Research and Development
In research and development, qPCR efficiency supports a range of applications, from basic research to vaccine development and biomanufacturing. The quantitative aspect of qPCR is crucial for gathering robust data, enabling scientists to draw accurate conclusions from their experiments.
- Assay Validation: In experimental settings, assessing qPCR efficiency helps validate assays. Researchers can refine their methodologies to ensure the accuracy and reproducibility of results.
- Gene Expression Studies: Understanding how genes are expressed under various conditions relies heavily on qPCR. The efficiency of amplification directly impacts the conclusions drawn from expression studies. High efficiency allows for better detection of low-abundance transcripts.
- Vaccine Development: During vaccine development, qPCR is used to quantify viral loads or detect vaccine-induced immune responses. Efficiency measurements in these assays can dictate the timeline for advancing candidates through clinical trials.
Overall, the applications of qPCR efficiency extend beyond mere amplification. They encompass a variety of domains where precise quantification plays an integral role in advancing scientific knowledge and improving decision-making in clinical practices.
The End
The conclusion of this article focuses on synthesizing the complex elements of the qPCR efficiency formula and its practical implications in molecular biology. Understanding this formula is imperative as it determines the reliability and accuracy of quantitative data produced by qPCR assays. It encapsulates the core principles necessary for rigorous experimental design and data interpretation.
Recap of Key Points
- Significance of qPCR: This article begins with an overview of quantitative PCR's critical role in biology, emphasizing how it allows for precise quantification of nucleic acids.
- Defining Efficiency: Efficiency in qPCR is pivotal as it reflects how effectively the target DNA is amplified. Understanding what constitutes efficiency helps in optimizing experiments.
- Application of the Efficiency Formula: The mathematical representation of the efficiency formula is explained. Its components are highlighted as significant for practitioners looking to enhance their experimental outcomes.
- Factors Influencing Efficiency: Various factors are identified that can impact the efficiency of qPCR, enabling researchers to troubleshoot issues effectively.
- Common Errors and Optimization Strategies: Errors in measurement and conditions affecting efficiency are discussed, along with practical best practices to enhance reliability in qPCR results.
- Applications in Diverse Fields: From clinical diagnostics to research and development, the diverse applications of qPCR efficiency are a focal point, underscoring its relevance across multiple scientific domains.
Future Directions in qPCR Technology
The evolution of qPCR technology is promising. Researchers are currently exploring several avenues:
- Improved Automation: Automation enhancements will streamline workflows, reducing human error and increasing throughput in laboratory settings.
- Increased Sensitivity and Specificity: Innovations in reagents and methodologies aim to boost sensitivity, allowing for detection of targets present in lower concentrations than currently possible.
- Integration with Microfluidics: Microfluidic technologies offer exciting possibilities for conducting numerous qPCR reactions simultaneously in a compact format.
- Real-time Monitoring Improvements: Enhanced real-time monitoring techniques are being developed, allowing researchers to observe amplification dynamics more closely.
In summary, as qPCR technology continues to advance, researchers will benefit from enhanced precision and broader applications. Staying informed about these developments is key for professionals aiming to leverage qPCR in innovative and impactful ways.