The Role of Chemical Elements in Science and Society
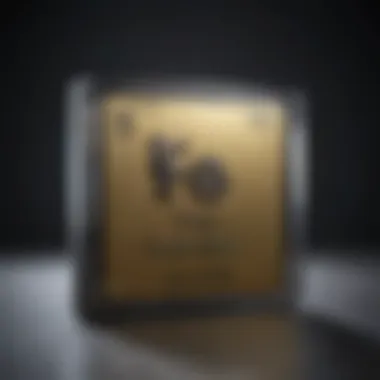
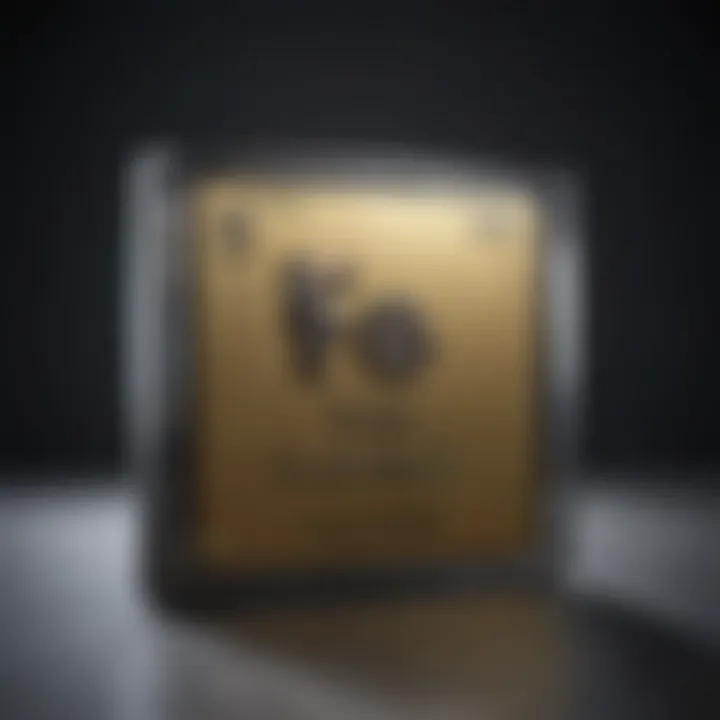
Intro
In the grand tapestry of the cosmos, chemical elements serve as the fundamental threads weaving together the very fabric of our world. From the air we breathe to the technology we rely upon, these elements not only form the foundation of matter but also play pivotal roles in numerous scientific and societal contexts. Whether through their elemental interactions or their contributions to various domains of human endeavor, understanding their essence is paramount.
The journey of dissecting these elements goes beyond mere categorization. It extends into a rich exploration of their properties, significance, and the historical milestones that have shaped our comprehension. As we delve into this article, we invite students, researchers, educators, and industry professionals alike to engage in meaningful dialogue about the chemical elements that underpin our reality.
This exploration revolves around several key components: the classification of elements in the periodic table, the relationships and trends exhibited by these elements, and the myriad ways they influence both natural processes and human-made innovations. In doing so, we seek to shed light on the profound impact that chemical elements have on our daily lives and on the advancement of science.
"Elements have been the building blocks of life and the structure of society, forming connections we utilize in every aspect of our existence."
As we navigate through this complex landscape, we will reflect on what these elements reveal about our universe and ourselves, enhancing our grasp of their enduring relevance. Let's embark on this enlightening journey that reveals the interconnectedness of science, society, and the very chemicals that form our existence.
Preamble to Chemical Elements
Chemical elements, the building blocks of matter, play an integral role in both the natural and designed worlds. They are not just symbols on a table; they form the essence of everything around us—plants, animals, and even the air we breathe. Understanding these elements is crucial for students, researchers, educators, and professionals engaged in various scientific fields. In this section, we will explore what makes chemical elements significant and how their study has unfolded throughout history.
Definition and Characteristics
At its core, a chemical element is a substance that cannot be broken down into simpler substances by chemical means. Each element is defined by the number of protons in its nucleus, known as the atomic number. Take hydrogen, for instance, which has one proton and is the simplest and lightest of all elements. Its presence is foundational in numerous chemical and biological processes.
Some common characteristics of elements include:
- Physical State: Elements can exist as solids, liquids, or gases at room temperature. For example, iron is a solid, while mercury is a liquid at this temperature.
- Atomic Mass: This reflects the average mass of an atom of an element, factoring in isotopic variations.
- Reactivity: Elements showcase different reactivity levels. Sodium, a highly reactive metal, quickly reacts with water, while gold remains practically inert in most environments.
Understanding these characteristics not only aids in the classification of elements but also provides insight into their behaviors in various chemical interactions.
Brief History and Discovery
The history of chemical elements is as captivating as it is educational. In ancient times, elements were categorized based on observable properties. The Greeks believed in four fundamental elements: earth, air, fire, and water. Fast forward to the late 18th century, and we see the groundwork of modern chemistry being laid by scientists like Antoine Lavoisier, who is often referred to as the father of modern chemistry. He categorized elements into metals, nonmetals, and gases and introduced the law of conservation of mass.
The 19th century brought about the formulation of the periodic table by Dmitri Mendeleev. By organizing elements according to their atomic weights and properties, he highlighted the periodicity of element characteristics. This was a pivotal moment in chemistry that sparked further research and led to the discovery of many elements we recognize today.
"The periodic table is the most important tool for chemists, providing a systematic way of understanding the vast variety of elements that exist and their relationships to each other."
The Periodic Table of Elements
The periodic table is more than just a colorful grid filled with numbers and letters; it's a map of the elements that shapes our understanding of chemical behavior and interactions. The intricate arrangement of elements allows scientists, students, and anyone curious about science to glean significant insights into the relationships among various chemical substances. Each element's position hints at its properties, behavior in reactions, and potential applications in both industry and daily life.
Through this structure, the periodic table serves as a powerful tool, grounding discussions about the roles and characteristics of elements in all areas of science and society.
Structure and Organization
Groups and Periods
Every element in the periodic table finds its home in either a group or a period. Groups run vertically and are numbered from one to eighteen, while periods run horizontally; they increase from one to seven. The unique aspect of groups is that elements within the same column often share similar chemical properties. For example, elements in group one, like lithium and sodium, exhibit strong reactivity because they each possess a single electron in their outermost shell. This characteristic makes them highly valuable for various chemical applications and reactions.
Additionally, the arrangement in periods helps scientists predict how elements will react. As you move from left to right across a period, elements tend to gain electrons, which affects their reactivity. This structure is essential not just for understanding chemistry but also for educating students and researchers about elemental behaviors in a clear and organized manner.
Metals, Nonmetals, and Metalloids
The classification of elements into metals, nonmetals, and metalloids is a defining feature of the periodic table. Metals dominate the left and center of the table, characterized by properties like conductivity and malleability. Nonmetals, found on the right, possess contrasting features, such as poor conductivity and a tendency to gain electrons. Metalloids, positioned along the zigzag line that separates metals from nonmetals, have properties of both, which makes them particularly useful in diverse applications, like semiconductors in electronics.
A beneficial choice for this article, this classification highlights the practical aspects of chemistry in fields such as materials science, where the understanding of these categories can lead to innovations in technology and engineering.
Lanthanides and Actinides
Lanthanides and actinides refer to two rows of 14 elements, starting from lanthanum and actinium, respectively, that are often displayed at the bottom of the periodic table. These elements are crucial, playing key roles in areas like nuclear science and material development. For instance, lanthanides are known for their use in high-tech applications such as phosphors in lighting and catalysts in refining processes. On the flip side, actinides include elements such as uranium and plutonium, which are vital in energy production but come with various concerns related to safety and environmental impact.
This distinction not only enriches the narrative of the periodic table but also emphasizes the societal implications of chemical research and use.
Periodic Trends
Atomic Radius
The atomic radius is a pivotal concept in understanding the size of atoms, which influences their reactivity and bonding characteristics. As you move down a group, the atomic radius generally increases because additional electron shells are added, which also leads to increased shielding effect making it easier for atoms to lose electrons. This can affect properties like conductivity or reactivity, making atomic radius a central aspect in predicting an element's behavior.
For instance, sodium has a larger atomic radius compared to lithium, which directly influences their reactivity levels; sodium is far more reactive. In an educational or research context, understanding atomic radius allows for deeper predictions about chemical behavior.
Electronegativity
Electronegativity is a key concept that measures an atom's ability to attract and hold onto electrons. This trait develops significant consequences when elements form compounds. In general, electronegativity increases across a period and decreases down a group on the periodic table. Consequently, elements like fluorine, with the highest electronegativity, are shown to attract electrons more effectively than metals such as lithium, creating a profound understanding of chemical bonding and reaction types.
In this article, targeting this aspect empowers students or professionals to grasp how and why some elements readily form bonds while others do not, shedding light on both fundamental chemistry and complex biochemical processes.
Ionization Energy
Ionization energy refers to the energy required to remove an electron from an atom. This value significantly influences the reactivity of an element. It tends to increase across a period due to the increasing nuclear charge that attracts electrons more strongly, making them harder to remove. Conversely, it decreases down a group since additional shells of electrons introduce increased distance from the nucleus and more shielding.
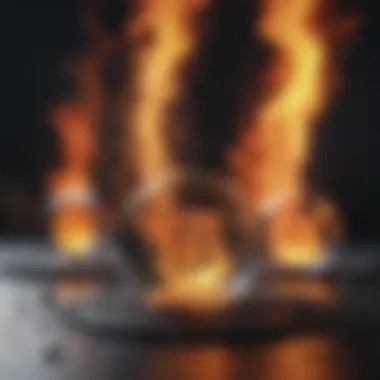
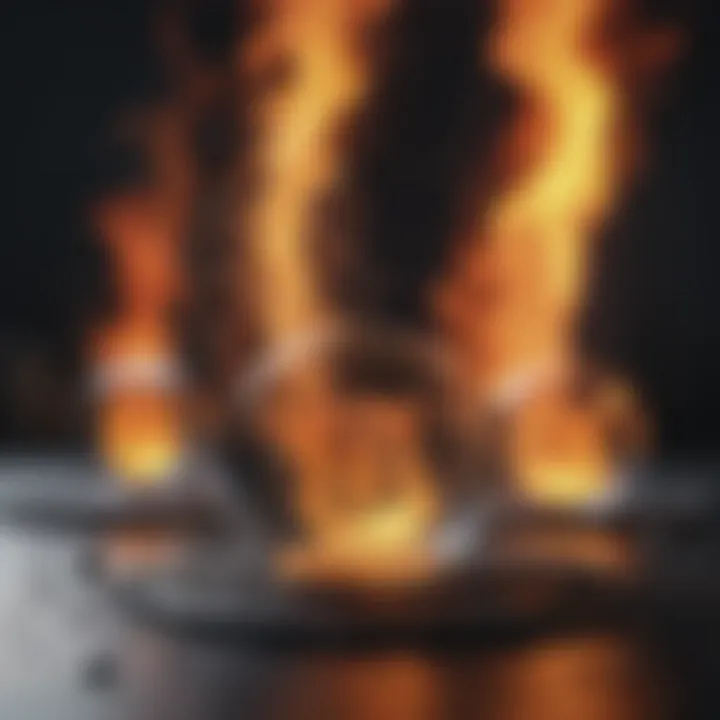
Understanding ionization energy is essential for explaining the behavior of elements during chemical reactions. For example, elements in the upper right-hand corner of the table, like helium or neon, require considerable energy to remove an electron, rendering them largely inert and resistant to reactions. Thus, this knowledge has broad implications, particularly in predicting elemental interactions in chemistry.
Elemental Properties and Their Significance
Understanding elemental properties is essential for grasping how these building blocks influence both science and society at large. Each element possesses unique physical and chemical properties that determine its behavior and interactions, rendering them fundamental to countless applications. Whether in industrial contexts or everyday life, these properties pave the way for the development of various technologies and substances, making them immensely relevant in contemporary discussions about science.
Physical Properties
State of Matter
The state of matter is arguably the most recognizable physical property of elements. It can be classified into solid, liquid, and gas forms, and this classification is crucial for various scientific applications. For instance, metals like iron are solid at room temperature, while mercury exists as a liquid. The state of an element not only reflects its atomic structure but also influences its physical interactions.
Solid metals offer structural stability in construction and manufacturing. On the flip side, gases like oxygen and nitrogen are vital for various biological processes. Thus, recognizing an element’s state is foundational in contexts ranging from chemical reactions to environmental science.
Density and Melting Points
Density and melting points are additional physical characteristics that are of great importance. Density can affect how elements behave when mixed with others; for instance, when oil and water are combined, oil, being less dense, floats atop the water. This property is not just an academic notion; in industries, it helps inform processes like separation and filtration.
Meanwhile, melting points reveal much about the metal's structure and bonding. Gold, with a high melting point, is often used in electronics because it ensures durability under various heating conditions. Knowing these properties assists in multiple applications, from crafting jewelry to manufacturing technologically advanced devices.
Color and Luster
The characteristics of color and luster similarly contribute to our understanding of elements. Metalloids like silicon can appear shiny, while elements like sulfur offer a more subdued appearance. This visual aspect affects both usage and aesthetics in various fields.
For example, the lustrous quality of metals makes them an attractive choice in jewelry and decorative items. Moreover, color can serve as an indicator of certain physical or chemical states. In acidity tests, the color change in litmus paper can signal the presence of acidic or basic substances. This interplay of appearance and function furnishes an array of significant applications in art and science alike.
Chemical Properties
Reactivity
Reactivity is a cornerstone of chemical behavior, indicating how readily an element engages in chemical reactions with others. Highly reactive elements like sodium react vigorously with water, while noble gases like neon display very low reactivity. Understanding reactivity is pivotal for safe handling and application in various settings.
For example, in the production of batteries, lithium's high reactivity underpins its utility, yet it also necessitates stringent handling protocols to prevent undesired reactions. Recognizing these nuances allows chemists to navigate potential hazards effectively while leveraging favorable traits.
Acid-Base Behavior
Acid-base behavior is another essential chemical property. This behavior is classified according to an element's ability to donate protons (acids) or accept them (bases). Such interactions are pivotal in various reactions, including neutralization and titration processes.
Acids like hydrochloric acid are widely used in industry for metal cleaning and pH adjustment. Recognizing the behavior of elements in this context aids scientists in formulating products for food, pharmaceuticals, and cleaning materials.
Combustibility
Combustibility indicates whether an element can ignite and sustain a chemical reaction in presence of oxygen. This property has substantial practical implications. For instance, hydrocarbons found in fossil fuels are crucial energy sources but also raise concerns about environmental effects due to their combustibility.
Meanwhile, elements like hydrogen have potential as clean fuel sources. Thus, understanding combustibility allows researchers to explore environmentally-friendly alternatives while also acknowledging the risks associated with highly combustible elements.
The study of elemental properties and their implications forms a bridge between theoretical concepts and real-world applications, making chemistry not just an academic discipline but a pillar of modern society.
Classification of Elements
Understanding the classification of elements is crucial for grasping their role in science and our daily lives. This system helps in organizing the elements based on their properties, which in turn makes it easier for chemists, engineers, and other professionals to communicate about them effectively. Elements are categorized mainly into metallic, non-metallic, and metalloid groups, each with its own distinct characteristics and relevance.
Metallic Elements
Metallic elements are an essential category within the periodic table. They exhibit unique properties that make them widely utilized in various applications, from structural materials to electronic devices.
Alkali Metals
Alkali metals, like lithium, sodium, and potassium, are known for their high reactivity, especially with water. This reactivity makes them invaluable in various chemical reactions and in the production of compounds. Their low melting points and densities set them apart from many other metals, making them particularly suitable for uses in batteries and other technologies. However, handling them requires caution due to their vigorous reactions.
- Key Characteristic: Extremely reactive, especially with water.
- Benefit in Article: Their role in batteries and other technologies highlights their relevance in modern applications.
- Unique Feature: The ability to easily lose one electron makes them ideal for forming compounds quickly, though this also represents a challenge during their storage and usage.
Transition Metals
Transition metals, such as iron, copper, and nickel, stand out due to their versatility and ability to form various compounds. These metals are known for their resilience and conductivity, making them indispensable in numerous industries, from construction to electronics.
- Key Characteristic: Ability to form variable oxidation states.
- Benefit in Article: Their use in construction, machinery, and electronics underscores their significance in global economies.
- Unique Feature: They can often catalyze reactions, which means they play critical roles in speeding up chemical processes without being consumed by the reaction.
Heavy Metals
Heavy metals, which include lead, mercury, and cadmium, pose particular challenges due to their toxicity at high concentrations. Yet, their properties provide unique advantages in various contexts, such as shielding and electrical applications.
- Key Characteristic: High density and often toxic to living organisms at elevated levels.
- Benefit in Article: Understanding their applications and repercussions can help in better industrial practices.
- Unique Feature: Their ability to conduct electricity and heat efficiently makes them useful, but managing their environmental impact is essential.
Nonmetals and Metalloids
Nonmetals and metalloids display a wide variety of characteristics and applications, significantly contributing to both industry and biological fields.
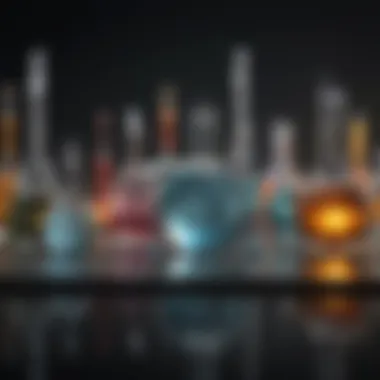
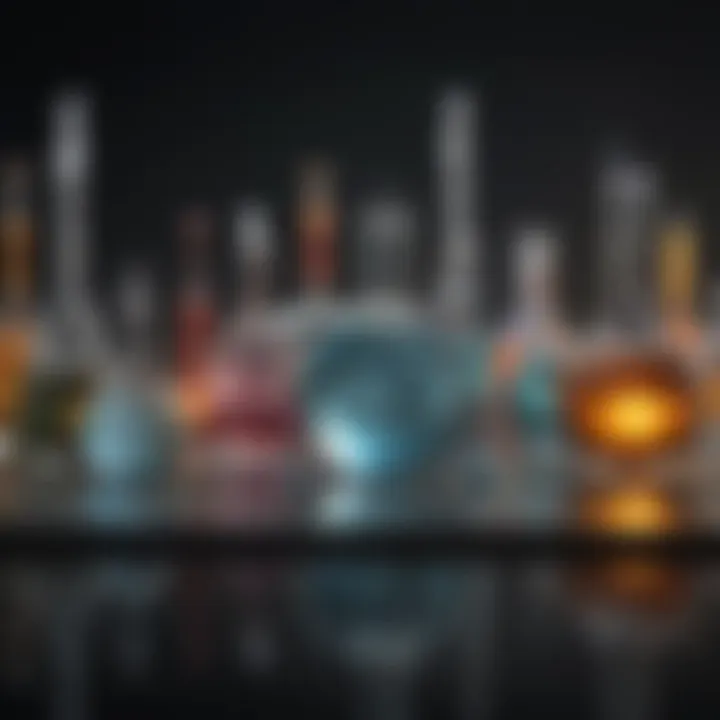
Properties and Examples
Nonmetals include elements like nitrogen and oxygen, while metalloids, like silicon and arsenic, bridge the gap between metals and nonmetals. Their distinct properties lead to their varied applications.
- Key Characteristic: Nonmetals are generally poor conductors, whereas metalloids possess mixed properties.
- Benefit in Article: Highlighting examples such as oxygen's role in respiration or silicon's utility in electronics enhances understanding.
- Unique Feature: Nonmetals often exist in gaseous or brittle solid states, adding diversity to the general concept of elements.
Uses in Industry
Nonmetals and metalloids play pivotal roles in industries ranging from agriculture to technology.
- Key Characteristic: Variety of applications based on diverse properties.
- Benefit in Article: Understanding these uses elucidates their impact on technology and everyday life.
- Unique Feature: For instance, silicon is a staple in the production of semiconductors, highlighting its dual nature as both a metalloid and a critical resource in technology.
Biological Importance
Many nonmetals are essential for life. Elements like carbon, hydrogen, and nitrogen form the backbone of biological molecules. Metalloids, too, have roles, albeit less direct.
- Key Characteristic: Biological necessity of nonmetals like carbon in DNA and proteins.
- Benefit in Article: Emphasizing their biological relevance showcases the intersection between chemistry and life.
- Unique Feature: These elements are involved in fundamental processes like photosynthesis and respiration, representing vital flow of energy in ecosystems.
Role of Elements in Chemical Reactions
Chemical reactions are the backbone of all matter transformations, and the elements involved play pivotal roles in these processes. Understanding how these elements function during reactions—each with its own characteristics and behaviors—is crucial for grasping broader concepts in science and society. Elements interact in unique ways; their reactions can lead to the creation of new compounds, energy release, and changes in material properties. Thus, the role elements play in chemical reactions affects everything from industrial applications to biological functions.
Types of Chemical Reactions
Synthesis Reactions
Synthesis reactions, often referred to as combination reactions, are where two or more reactants combine to form a single product. This type of reaction is characterized by the formation of more complex substances from simpler ones. A primary feature of synthesis reactions is their ability to produce essential compounds, such as water from hydrogen and oxygen gases. This aspect is highly beneficial in chemical manufacturing processes, making it a cornerstone of industries ranging from pharmaceuticals to materials science.
The unique property of synthesis reactions is their straightforwardness; they often yield predictable results. However, a downside can be that these reactions may require specific conditions like temperature and pressure for optimal outcomes. Lack of control can lead to unwanted byproducts, posing challenges in practical applications.
Decomposition Reactions
Conversely, decomposition reactions take one reactant and break it down into two or more simpler products. A key characteristic of these reactions is that they often require an input of energy, such as heat, light, or electricity, to occur. Commonly seen in processes like electrolysis, where water is decomposed into hydrogen and oxygen gases, this reaction type illustrates the fundamental principle of chemical breakdown.
Decomposition reactions contribute significantly to the recycling of materials and energy conservation. However, they can also be unpredictable, sometimes yielding gases or other substances that must be managed properly, especially in industrial settings. The challenge lies in controlling the energy input to avoid miscalculations that lead to inefficiencies or safety issues.
Redox Reactions
Redox, or reduction-oxidation reactions, involve the transfer of electrons between substances. In this reaction type, one element is oxidized (loses electrons) while another is reduced (gains electrons). This duality of change highlights the interconnectedness of elements in reaction dynamics.
What makes redox reactions particularly compelling is their ubiquitous presence in both natural and artificial processes. For instance, cellular respiration and combustion are governed by redox mechanisms, playing vital roles in energy production and material transformations.
However, they can also present complexities that must be managed in various applications. Precise conditions must be maintained to ensure that reactions proceed without detrimental side reactions, making them a delicate balancing act in chemistry.
Catalysis and Reaction Mechanisms
Catalysis is a crucial aspect of chemical reactions, as it facilitates reaction processes without being consumed in the reaction itself. Understanding the mechanisms behind catalysis allows for improved efficiency and cost-effectiveness in achieving desired products.
Enzymatic Catalysis
Enzymatic catalysis represents a significant area in biochemistry, where specific enzymes accelerate biological reactions. Enzymes lower the activation energy needed for reactions, making processes that would otherwise take an exceedingly long time occur at a much faster rate. The specificity of enzymes means they can facilitate reactions with remarkable precision, yielding only the intended products.
The unique feature of enzymatic catalysis is that it operates under mild conditions, unlike many industrial catalysts that require extreme temperatures or pressures. However, their sensitivity to environmental changes can be a disadvantage; factors like pH and temperature can affect enzyme activity, which might lead to loss or reduced efficiency.
Homogeneous vs. Heterogeneous Catalysts
In discussing catalysis, it’s essential to differentiate between homogeneous and heterogeneous catalysts. Homogeneous catalysts are in the same phase as the reactants, usually dissolved in a solution, allowing for more intimate interactions. This can lead to more rapid reaction rates and high selectivity. On the flip side, heterogeneous catalysts are in a different phase, typically solid catalysts operating in gaseous or liquid mixtures.
While homogeneous catalysts can be highly efficient, their recovery can be complicated since they remain mixed with the product. Heterogeneous catalysts, while easier to separate post-reaction, may have slower reaction rates due to limited surface area exposure.
Understanding these differences is vital for selecting the right type of catalyst for specific applications, whether in industrial production, environmental remediation, or sustaining life processes.
Applications of Chemical Elements
Understanding the applications of chemical elements reveals their undeniable importance in science and society. These elements are not merely theoretical concepts found in textbooks; they play pivotal roles in various industries, shaping technologies, our health, and even our environment. From practical utilities to innovative advancements, elements lie at the core of many foundational processes in both industry and daily life.
In Industry
Metals in Construction
Metals are the backbone of construction materials. Steel, for instance, combines iron with carbon, enhancing strength while maintaining ductility. This characteristic makes it a favored choice in building skyscrapers, bridges, and various infrastructures. Furthermore, aluminum, known for its lightweight property, is often utilized in applications like roofing and windows.
"The elements we choose can change the very skyline of our cities."
However, there's the double-edged sword of cost and resource availability. Using metals can sometimes escalate project expenses and lead to environmental concerns, particularly through mining and resource depletion. Striking a balance between cost-effectiveness and sustainability remains a challenge within the industry.
Elements in Electronics

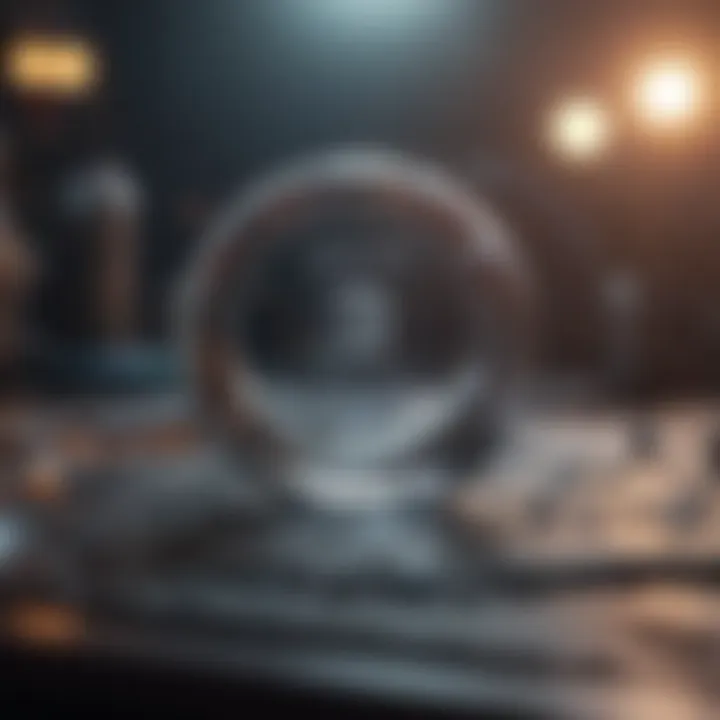
In the realm of electronics, specific elements like silicon and copper cannot be overstated. Silicon, a semiconductor, is crucial for manufacturing computer chips. Its ability to control electrical flow has revolutionized the digital age. In contrast, copper is an excellent conductor, used extensively in wiring and circuit boards.
What truly stands out about silicon is its abundance and versatility, forming the bedrock of modern technology. The downside, however, is that the processing of these elements can be energy-intensive, contributing to higher carbon footprints. Ensuring an eco-friendly approach in the tech industry is essential for future sustainability.
Chemicals in Pharmaceuticals
The pharmaceutical sector is another domain where chemical elements are critical. Elements such as carbon, hydrogen, oxygen, and nitrogen form the building blocks of countless drugs. For instance, compounds derived from these elements have led to life-saving medications like antibiotics and analgesics.
The unique feature here is the specificity of these elements. Their chemical properties allow for targeted therapies in treating various diseases, making them a popular choice in medicine. Yet, the challenge lies in the need for constant innovation in creating more effective and less harmful drugs, balancing the benefits of chemistry with patient safety and environmental considerations.
In Daily Life
Household Chemicals
Household chemicals, composed of various elements, find themselves in our cleaning products, personal care items, and even our food. Sodium and chlorine, for instance, combine to form table salt, an essential nutritional element. Meanwhile, carbon compounds are omnipresent in detergents and surfactants.
The characteristic feature of household chemicals is their accessibility. They are readily available, often at low costs, making them popular among consumers. However, their environmental impact can be significant, often causing pollution if not disposed of properly. Raising awareness about sustainable practices in household usage is crucial to mitigate these effects.
Nutrition and Elements
Elements also play a vital role in nutrition. Essential minerals like calcium, potassium, and iron contribute directly to our health, affecting everything from bone density to blood pressure. A balanced diet incorporates various nutrient-rich foods that provide these essential elements.
The unique aspect of nutritional elements is their necessity; without them, our bodies wouldn't function optimally. Yet, with the rise of processed foods, there is a risk of deficiency, emphasizing the need for conscious choices in our diets.
Environmental Impacts
The environmental implications of chemical elements are profound. The extraction and use of elements can either harm or benefit ecosystems. For instance, sustainable practices in resource extraction can help preserve biodiversity, while overuse of certain elements can lead to habitat destruction.
Key features of understanding these impacts focus on awareness and responsibility. By recognizing which elements contribute to pollution or ecological harm, society can work towards better strategies in both industry and daily practices. Advocating for eco-friendly materials and recycling processes has become an essential part of modern environmental science.
The Future of Elemental Research
The study of chemical elements is not static; it is an evolving field that holds promises and challenges for the future. As we continue to unravel the complexities of elemental properties and behaviors, the importance of ongoing research becomes clear. The future of elemental research can significantly impact both scientific advancements and societal developments. This evolution is characterized by the increasing emphasis on sustainability, the incorporation of innovative technologies like nanotechnology, and the pressing need to address challenges around resource conservation and environmental regulations.
Emerging Trends in Chemistry
Sustainable Chemistry
Sustainable chemistry champions the development of chemical processes that minimize waste and energy use while maximizing efficiency. Its main goal is to create a balance between the benefits of chemical research and the preservation of our environment. One of the key characteristics of sustainable chemistry is its focus on green processes, which often rely on renewable resources. This approach is gaining traction because it aligns well with global initiatives aimed at reducing ecological footprints.
A unique feature of sustainable chemistry is its application in the design of new materials and chemical products that degrade harmlessly in nature. For example, biodegradable plastics and eco-friendly solvents can dramatically reduce pollution levels. However, there are challenges, such as the higher initial costs of green chemistry alternatives compared to traditional methods, which can deter widespread adoption. Moreover, the need for new regulations and standards complicates the transition toward more sustainable practices.
Nanotechnology
Nanotechnology is another frontier that holds significant potential in the realm of chemical elements. It involves manipulating matter on an atomic and molecular scale, creating materials with novel properties. The key characteristic of nanotechnology is its ability to enhance material performance while often using fewer resources. This makes it a compelling option for various applications, from drug delivery systems to energy storage solutions.
A unique aspect of nanotechnology is its versatility; it can improve existing materials or create entirely new ones with tailored properties. For instance, in the field of electronics, nanotechnology contributes to smaller, faster, and more efficient devices. However, its rapid development raises questions about safety and long-term environmental impacts. As the field progresses, balancing innovation with responsible use is paramount.
Challenges in Elemental Study
Despite the advancements, there are several challenges regarding the study and application of chemical elements.
Resource Conservation
Resource conservation remains a critical concern in elemental study, as the demand for natural resources grows alongside population increase. Effective resource management practices must be developed and implemented to ensure the sustainable use of materials. One important characteristic of resource conservation is the push for recycling and upcycling existing materials rather than relying solely on new ones. This practice can drastically reduce waste while conserving valuable natural elements.
A unique feature of resource conservation is the promotion of circular economy principles, where products are designed for durability and reuse. However, the transition to such models can be slow and hindered by economic factors, including the perceived high costs of recycling technologies compared to traditional disposal methods.
Environmental Regulations
Environmental regulations are ever-tightening across the globe, aiming to control emissions and waste associated with chemical production and usage. These regulations are a key characteristic of modern chemical research, pushing industries to innovate in ways that comply with legal frameworks. This pressure can foster creativity and lead to breakthroughs in cleaner technologies.
A notable unique feature of environmental regulations is their dynamic nature, often shifting in response to public sentiment and new scientific findings. While these regulations are essential for protecting ecosystems, they can pose challenges for researchers and manufacturers seeking to innovate. In some cases, compliance can require significant modifications to existing processes, which may not be feasible for all companies.
Understanding and navigating the intersection of these emerging trends and challenges is crucial for advancing research in chemistry and ensuring societal benefits.
The End
The significance of chemical elements spans far beyond their individual properties; they are the backbone of not just scientific exploration but also daily living. The better one understands these building blocks of matter, the more adept one becomes at appreciating the intricacies of life itself. The articles discusses how elements contribute to various domains, from industrial applications to the deeper insights they provide into the universe. This examination reveals layers of interaction beneath what might initially appear a simple classification of substances.
Recognizing the vital role of chemical elements equips individuals with knowledge that extends into numerous fields, stimulating innovation, environmental awareness, and improved health.
Recapitulation of Key Concepts
In revisiting the essential concepts laid forth in the article, it’s crucial to acknowledge that chemical elements are categorized and analyzed not only for their isolated characteristics but also for how they interact within compounds and mixtures. Through the dissection of the periodic table, readers grasp the organization of elements, understanding how trends like atomic radius and electronegativity play into the reactivity of substances.
Additionally, the importance of classifications, such as metals, nonmetals, and metalloids, showcases how divergent properties can lead to varied applications in different spheres—from the construction industry to perspectives on biological significance. The examination of chemical reactions, particularly in terms of catalysts, paves the way for advancements in both industrial and medicinal chemistry, altering the landscape of technological growth.
Final Thoughts on Chemical Elements
As we interpret the role of chemical elements, it becomes clearer that they are not merely tools for scientists; they are vital components of an interconnected ecosystem. The study of elements invites an ever-expanding dialogue about sustainability, conservation, and innovation. Such understanding is not confined to labs; it extends into our homes and communities, influencing everything from the products we use every day to the way we consider environmental impact.
Engagement with elemental research holds promise for addressing future challenges, such as resource limitations and environmental degradation. Ultimately, the continual exploration in this realm sparks questions about the ethical implications of element manipulation, making it imperative that society stays informed and proactive.
Furthermore, as new technologies emerge, the study of chemical elements will need to adapt, revisiting old paradigms while embracing new scientific discoveries. Such dynamism reflects the ongoing journey of knowledge, reminding us that beneath the surface of each element lies potential yet to be unlocked.