Exploring the Role of Murine Embryonic Fibroblasts
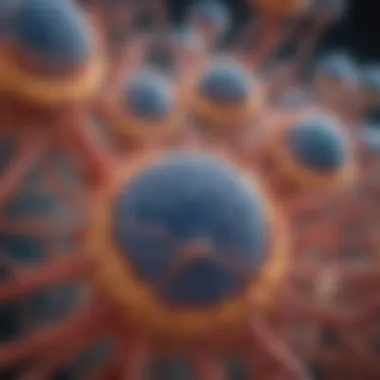
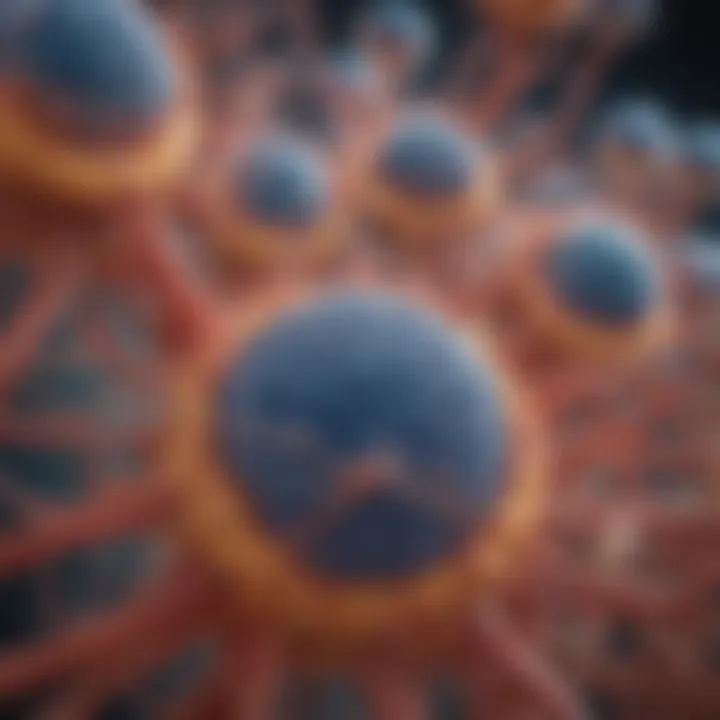
Intro
Murine embryonic fibroblasts (MEFs) play a pivotal role in modern biomedical research. These cells are derived from the early-stage embryos of mice and have been extensively utilized in various scientific investigations. Understanding MEFs is crucial, as they serve as a model system to elucidate cellular behaviors, assess genetic variations, and investigate disease mechanisms. Given their unique properties, they have become integral to regenerative medicine, cancer research, and developmental biology studies.
The utility of MEFs stems from their ease of manipulation and their responsiveness to experimental conditions. Researchers can transform these cells for specific studies, making them a flexible tool in the laboratory. This article provides an in-depth examination of MEFs, highlighting their significance in various fields of research. It aims to offer a comprehensive guide that reveals the complexities of these cells, starting from their origins to the implications of their use in advanced scientific inquiries.
Reserach Overview
Summary of Key Findings
The analysis of murine embryonic fibroblasts indicates several crucial points that contribute to ongoing research:
- Versatility in research: MEFs are adaptable for different experiments, enabling various genetic modifications and assays.
- Cellular behaviors: Studies of MEFs provide insights into cell signaling pathways, differentiation, and other cellular mechanisms.
- Disease modeling: MEFs are valuable in modeling genetic disorders, offering paths to understand disease progression and potential therapies.
Overall, these findings underline the essential role of MEFs in advancing scientific knowledge and potential therapeutic applications.
Background and Context
Murine embryonic fibroblasts were first isolated for their properties that mimic those of human cells. Since then, they have been a cornerstone for various experiments aimed at dissecting complex biological questions. The historical context of MEFs extends back to initial studies on somatic cell nuclear transfer and pluripotency, where these fibroblasts facilitated significant breakthroughs in stem cell research.
Their importance is underscored by their use in various applications, such as gene targeting techniques and studies on cellular response to external stimuli. With increasing attention towards regenerative medicine and cancer research, MEFs continue to yield insights into both normal physiology and pathological processes.
Methodology
Experimental Design
Research involving MEFs often employs an experimental design that takes into account the specific hypothesis being tested. Common focuses include:
- Manipulating genetic expression: Using techniques like CRISPR/Cas9 to understand gene functions.
- Analyzing cell behaviors: Observing the response of fibroblasts to stress or other signaling molecules.
Understanding these methodologies is key for designing effective experiments that yield reliable results when working with MEFs.
Data Collection Techniques
Data collection involving MEFs typically utilizes a variety of analytical methods and technologies. Common techniques include:
- Flow cytometry for assessing cellular characteristics and viability.
- Immunofluorescence to visualize specific proteins within the cells.
- Genomic sequencing to examine genetic alterations.
Such techniques enable researchers to gather quantifiable data, facilitating a thorough understanding of the underlying biological processes.
Prolusion to Murine Embryonic Fibroblasts
Murine embryonic fibroblasts (MEFs) play a pivotal role in biomedical research. Their significance stems from their unique properties, which enable researchers to investigate cellular behavior, genetic manipulation, and disease modeling. These cells derived from mouse embryos provide an accessible model to draw parallels with human biology. Consequently, understanding MEFs can lead to breakthroughs in regenerative medicine and contribute to advancements in cancer therapies.
Definition and Characteristics
Murine embryonic fibroblasts are primary cells obtained from the tissues of mouse embryos, specifically from the fibroblastic layer of the embryonic mesoderm. They exhibit several defining characteristics. MEFs are known for their high proliferative capacity, allowing them to be cultured extensively in laboratory settings. Moreover, they maintain a stable karyotype, which contributes to their utility in genetic studies. MEFs can be isolated from different embryonic stages, typically from 12.5 days of gestation onward. Their ability to differentiate into various cell types is another aspect that makes them valuable in research.
The ability of MEFs to retain their identity while adapting to various experimental conditions underscores their importance in scientific investigations.
Historical Context
The history of murine embryonic fibroblasts traces back to early research in developmental biology. The first attempts to culture these cells were initiated in the mid-20th century. Researchers recognized the potential of MEFs for studying mammalian genetics and cellular differentiation. Over decades, protocols were refined, enhancing the reproducibility and reliability of MEF cultures. In the 1980s, MEFs gained prominence particularly with the rise of gene manipulation techniques such as homologous recombination. This advance allowed for the creation of knockout mice, thus expanding the scope of functional studies in genetics. Throughout the years, MEFs have remained a cornerstone in cell and molecular biology research, contributing to our understanding of key biological processes.
Developmental Biology and Cellular Origins
Understanding the developmental biology and cellular origins of murine embryonic fibroblasts is crucial for grasping their role in research and therapeutic applications. This section outlines how these cells are vital to the study of various biological processes, including differentiation, lineage specification, and tissue formation. The knowledge gained from studying embryonic fibroblasts impacts fields such as developmental biology, regenerative medicine, and cancer research.
Embryonic Development Stages
Murine embryonic fibroblasts emerge during specific stages of mouse embryonic development. The key stages include:
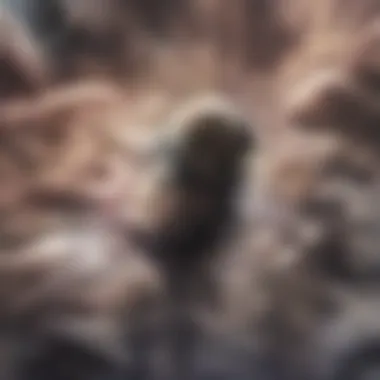
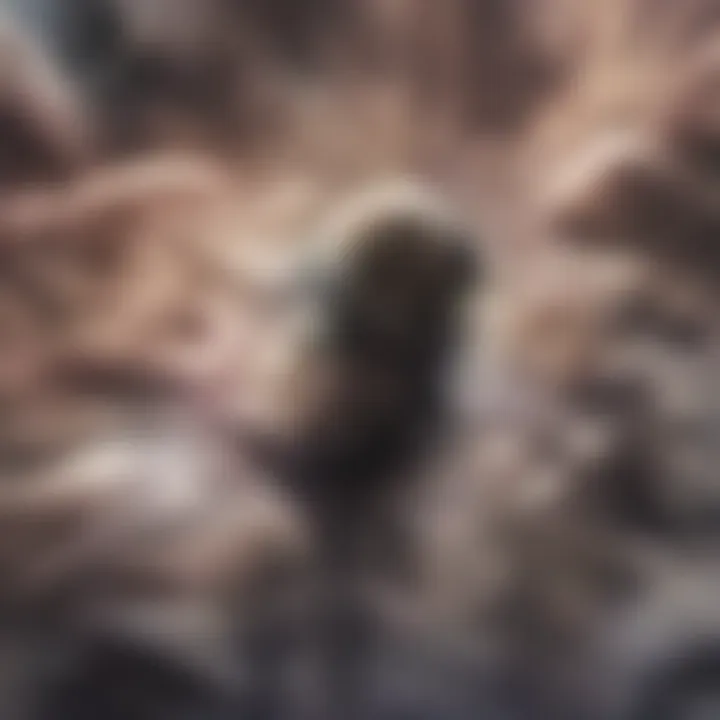
- Pre-Implantation Stage: Cells undergo cleavages leading to the formation of the blastocyst. At this stage, the inner cell mass forms which differentiates into various cell lineages, one being the fibroblasts.
- Implantation Stage: Following successful implantation in the uterine wall, cells begin to specialize. The outer layer, which is the trophectoderm, facilitates implantation and surrounding structures start to form.
- Early Gastrulation: This is when significant differentiation occurs. The epiblast cells, from which fibroblasts can later derive, transition into distinct lineages contributing to the mesoderm and endoderm layers.
Through these stages, murine embryonic fibroblasts become characterized by their unique properties, which set them apart from other cell types. This differentiation is fundamental to their later applications in research.
Cell Lineage and Differentiation
Cell lineage and differentiation are pivotal in understanding the functional roles of murine embryonic fibroblasts. As stem cells differentiate, they take on specific functions and characteristics.
- Lineage Specification: From a developmental standpoint, mesodermal progenitors give rise to various lineages. Fibroblasts, as part of this lineage, contribute to tissue formation and repair.
- Extracellular Matrix (ECM) Interactions: Fibroblasts play an essential role in synthesizing the extracellular matrix, which is vital for tissue integrity and cell signaling. They interact closely with different cell types, facilitating communication and structural support.
In summary, the study of developmental biology provides a comprehensive framework for understanding murine embryonic fibroblasts. This knowledge is not only essential for academic inquiry but also lays the groundwork for practical applications in regenerative medicine and cancer biology. The implications of how these cells differentiate and engage in their microenvironments will continue to shape future research.
Culturing Techniques for Murine Embryonic Fibroblasts
The importance of culturing techniques for murine embryonic fibroblasts cannot be overstated. These methods enable researchers to manipulate and study these cells effectively, ensuring reliable and reproducible results in various experimental contexts. Understanding the nuances of culturing techniques allows scientists to maximize the potential of murine embryonic fibroblasts in both basic and applied research. Key considerations include isolation procedures, maintenance and propagation methods, and cryopreservation practices. Each aspect contributes significantly to ensuring cellular viability and functionality over time.
Isolation Procedures
Isolation procedures for murine embryonic fibroblasts are the first step in working with these cells. This process typically begins with the extraction of embryos from pregnant mice. Researchers often use specific strains, such as C57BL/6 or BALB/c, based on their genetic backgrounds. After obtaining the embryos, the next step involves dissection to isolate the fibroblastic cells. This could include the following methods:
- Tissue dissociation: Mechanical and enzymatic dissociation methods are used to separate cells from connective tissues. Enzymes like trypsin or collagenase are often employed.
- Filtration and Centrifugation: The dissociated cellular mixture is filtered and centrifuged to separate fibroblasts from other cell types and debris.
Proper isolation techniques are crucial as they impact the purity and quality of the cell cultures. Ensuring minimal contamination and selecting the right growth medium can significantly influence downstream applications.
Maintenance and Propagation
Once isolated, the maintenance and propagation of murine embryonic fibroblasts become paramount. These cells require specific culture conditions to thrive. Key elements include:
- Growth Media: Commonly, DMEM (Dulbecco's Modified Eagle Medium) supplemented with fetal bovine serum (FBS) and antibiotics like penicillin-streptomycin are used.
- Culture Environment: Fibroblasts are typically cultured in a humidified incubator at 37 degrees Celsius with 5% CO2.
- Subculturing: Regular passaging is necessary to maintain healthy cell populations. Typically, cells are subcultured at a ratio of 1:3 to 1:5 when they reach approximately 70-80% confluency.
Maintaining proper conditions allows cells to exhibit their biological properties more accurately. Frequent monitoring for contamination and cellular health is essential for successful propagation.
Cryopreservation Practices
Cryopreservation is essential for the long-term storage of murine embryonic fibroblasts. This technique enables scientists to preserve cell lines for future experiments. Key aspects of cryopreservation include:
- Cryoprotectants: Commonly used cryoprotectants like DMSO (dimethyl sulfoxide) and glycerol help protect cells during the freezing process.
- Cooling Rates: Controlled cooling is necessary to avoid ice crystal formation, which can damage cells. Generally, a rate of about 1 degree Celsius per minute is ideal for optimal cell survival.
- Storage: Cells are typically stored in liquid nitrogen, maintaining an environment that is -196 degrees Celsius.
When properly implemented, cryopreservation can maintain cell integrity for extended periods. This allows researchers to preserve valuable cell lines without compromising their functionality.
"Effective culturing techniques form the backbone of successful experimental outcomes in murine embryonic fibroblast research."
Molecular Properties of Murine Embryonic Fibroblasts
Molecular properties of murine embryonic fibroblasts are fundamental to understanding their relevance in various research fields. These properties underline the potential of these cells in developmental biology, regenerative medicine, and cancer research. The analysis of molecular characteristics shed light on the behavior, adaptability, and functionality of murine embryonic fibroblasts, making them instrumental in experimental setups.
Gene Expression Profiles
Gene expression profiling of murine embryonic fibroblasts provides critical insights into the cellular machinery. By examining the specific genes that are active, researchers can determine how these cells respond to various stimuli and conditions. For instance, fibroblasts in different developmental stages demonstrate distinct gene expression patterns. This specificity is crucial when considering their application in research.
- Developmental Signals: Certain genes activate during embryonic development, highlighting the cells’ role as precursors to more specialized tissues.
- Response to Stress: In conditions like hypoxia or nutrient deprivation, unique gene expressions occur that can lead to insights into cellular survival mechanisms.
Understanding these profiles helps in studying how fibroblasts influence other cell types and their environment. Such knowledge also allows for manipulation of these cells for therapeutic purposes. Researchers often utilize tools like qPCR and microarrays to quantify gene expression levels.
Pathways and Mechanisms
Investigating the pathways and mechanisms that govern the function of murine embryonic fibroblasts reveals how these cells interact with their surroundings. Various signaling pathways are involved, which drive processes such as proliferation, differentiation, and migration. Here are key pathways worth noting:
- TGF-β Pathway: This pathway plays a significant role in regulating cell growth and differentiation. Its activation results in fibroblast activation, which can impact wound healing and tissue fibrosis.
- Wnt/β-catenin Signaling: This pathway is crucial for maintaining stem cell properties. Murine embryonic fibroblasts expressing Wnt can self-renew and contribute to tissue engineering applications.
The mechanisms behind these pathways are intricate. For example, interactions between different signaling pathways can create a complex network that alters gene expression in response to external factors. Understanding these connections not only enhances knowledge about fibroblast biology but also underscores their potential in therapeutic interventions.
The molecular properties of murine embryonic fibroblasts unveil their fundamental roles in biological research, bridging gaps in understanding development, regenerative medicine, and cancer biology.
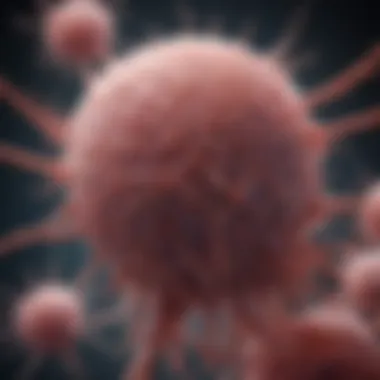
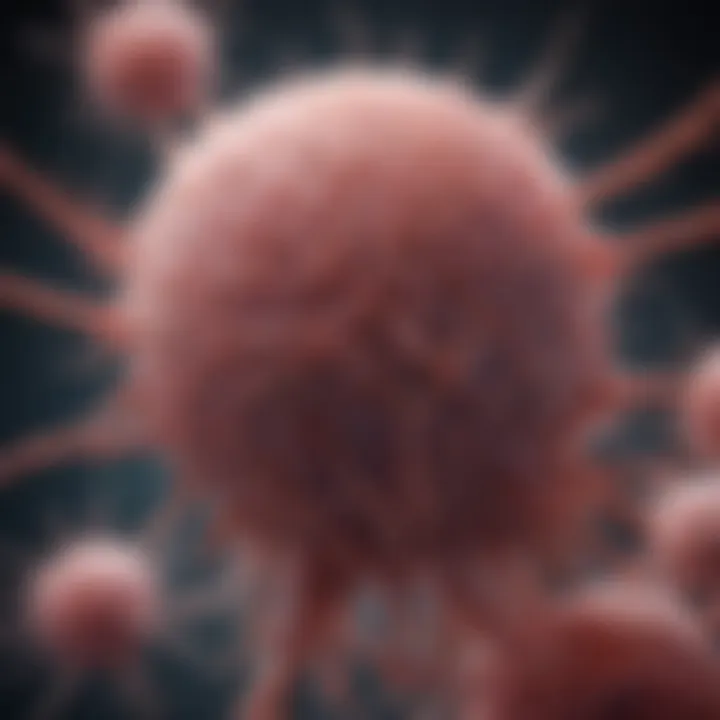
Functional Applications in Research
Murine embryonic fibroblasts (MEFs) are pivotal in various realms of scientific inquiry. Their unique properties and versatility make them invaluable not just as a cell model but as a vital component in translational research. This section elucidates the significance of functional applications of MEFs, focusing on how they contribute to understanding biological processes and their implications in various fields, such as regenerative medicine and cancer research.
In Vitro Studies
In vitro studies utilizing murine embryonic fibroblasts have revolutionized our understanding of cellular mechanisms. These studies allow researchers to manipulate variables within a controlled environment, offering insights into cell behavior. MEFs serve as a robust platform for assessing drug responses, investigating cell signaling pathways, and exploring cellular interactions.
One of the main advantages of using MEFs in vitro is their ability to mimic physiological conditions. As a result, researchers can obtain data that is often predictive of how cells behave in vivo. Furthermore, MEFs can be genetically modified, enabling targeted studies into gene function and disease modeling. This flexibility fosters a deeper comprehension of oncogenic processes, which is essential for developing targeted therapies.
In vitro, MEFs have been particularly useful in stem cell research. They provide a supportive microenvironment that facilitates the isolation and characterization of stem cells. Their role in stem cell culture contributes to the advancement of stem cell therapies and regenerative medicine applications.
In Vivo Models
In vivo studies are crucial for translating findings from in vitro models to real biological systems. Murine embryonic fibroblasts have been extensively used as a tool in animal models for evaluating cellular behaviors and therapeutic interventions. When MEFs are introduced into vivo systems, they interact intricately with host tissues, enabling researchers to assess their functionality in a living organism.
Research involving MEFs in vivo provides insights into various disease contexts, including cancer progression and tissue repair mechanisms. Notably, tumors often contain fibroblasts that influence growth and metastasis. By utilizing MEFs in these studies, scientists can explore the tumor microenvironment, elucidating the role of fibroblasts in cancer biology.
Additionally, MEFs serve in regenerative medicine studies, where they are employed to assess tissue repair processes. Their applications range from evaluating wound healing to providing insight into organ development.
"Murine embryonic fibroblasts remain a cornerstone in biological research, facilitating both in vitro and in vivo insights into complex processes."
Role in Regenerative Medicine
Murine embryonic fibroblasts (MEFs) play a crucial role in regenerative medicine. Their unique characteristics make them an essential part of research and development efforts aimed at understanding and applying regenerative therapies. This section explores the significance of MEFs in the realm of regenerative medicine, particularly in relation to stem cell research and tissue engineering.
Stem Cell Research Connections
MEFs are often used as feeder cells for stem cell culture. They provide a supportive environment that promotes the growth and maintenance of pluripotent stem cells. This interaction is pivotal because it ensures that stem cells retain their undifferentiated state. By using MEFs, researchers can better study cell differentiation pathways and mechanisms.
The importance of MEFs extends beyond mere support; they actively influence stem cell behavior. The secreted factors from MEFs, such as growth factors and cytokines, contribute to the modulation of stem cell fate. By understanding these interactions, scientists can enhance protocols for stem cell generation and differentiation, which are vital for developing therapies for various diseases and injuries.
"The relationship between MEFs and stem cells is a foundation for advancements in regenerative medicine."
Tissue Engineering Developments
In the context of tissue engineering, MEFs offer numerous advantages. Their ability to proliferate and maintain viability under various conditions makes them ideal candidates for creating engineered tissues. Researchers utilize MEFs in scaffolding structures due to their extracellular matrix components, which mimic the natural tissue environment.
The applications of MEFs in tissue engineering are diverse, including skin regeneration, cartilage repair, and even muscle tissue formation. Their versatility allows for experimentation with different tissue types, enhancing our understanding of how to effectively regenerate damaged or diseased tissues.
Some key points regarding MEFs in tissue engineering include:
- Biocompatibility: MEFs can integrate well into host tissues.
- Growth Factor Production: They secrete various growth factors essential for tissue development.
- Modeling Human Diseases: MEFs can be genetically manipulated to model human conditions, providing a practical approach to testing new treatments.
Collectively, the relationship between murine embryonic fibroblasts and regenerative medicine highlights the importance of these cells in advancing scientific knowledge and therapeutic strategies. As research progresses, it is likely that the applications of MEFs will expand, further embedding them in the future of regenerative therapies.
Murine Embryonic Fibroblasts and Cancer Research
Murine embryonic fibroblasts present a compelling avenue for cancer research due to their unique properties and versatile applications in understanding tumor biology. These cells are often used to create in vitro models that mimic the tumor microenvironment. By studying murine embryonic fibroblasts, researchers can gain insight into how tumors develop, progress, and respond to various treatments. This section will explore the two significant aspects: tumor microenvironment studies and genetic manipulation for oncological research.
Tumor Microenvironment Studies
The tumor microenvironment plays a critical role in cancer progression and metastasis. It consists of various cell types, including fibroblasts, immune cells, and endothelial cells. Murine embryonic fibroblasts facilitate the simulation of this environment, providing valuable context for studying how tumors interact with surrounding tissues.
Several factors can influence the behavior of these cells within the tumor microenvironment:
- Cytokine Secretion: Murine embryonic fibroblasts can secrete cytokines that influence the activity of tumor cells and immune responses. This crosstalk can unveil the complexities of tumor-driven signaling pathways.
- Extracellular Matrix Composition: The extracellular matrix influences cell proliferation, migration, and differentiation. The matrix produced by fibroblasts can affect tumor cell behavior, providing a rich area for investigation.
- Cell-Cell Interactions: Interactions between tumor cells and fibroblasts can alter the tumor phenotype, including drug resistance and invasion capabilities.
Researchers utilize murine embryonic fibroblasts to model these interactions, allowing them to assess therapeutic strategies aimed at disrupting the supportive roles played by fibroblasts in tumor growth.
Important Note: Understanding the interplay between tumor cells and murine embryonic fibroblasts is crucial for developing targeted therapies that can inhibit cancer progression.
Genetic Manipulation for Oncological Research
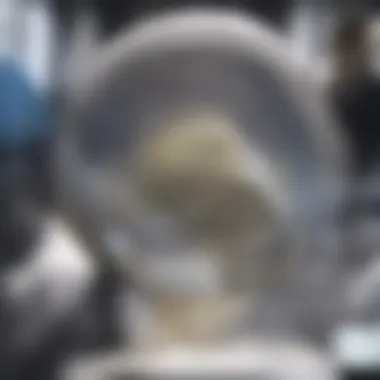
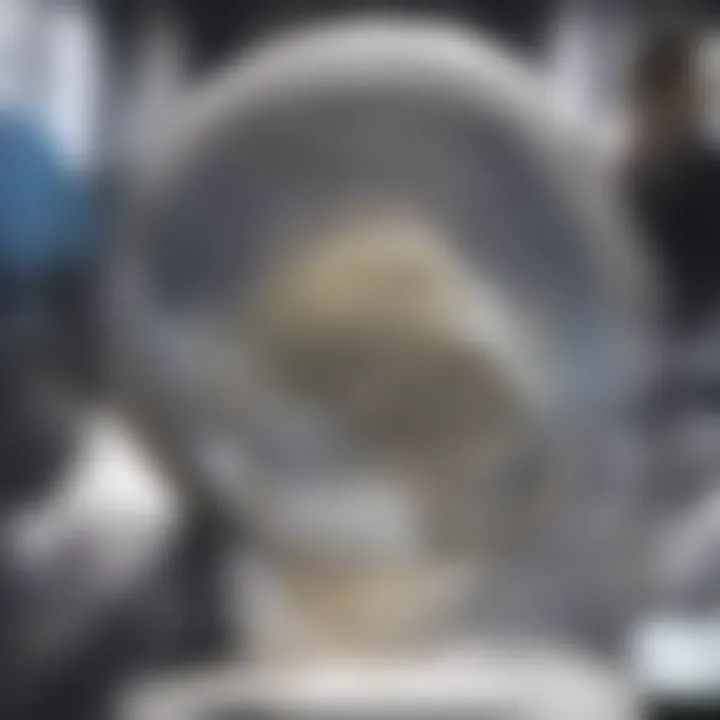
Genetic manipulation of murine embryonic fibroblasts provides a robust tool for uncovering the genetic underpinnings of cancer. For instance, researchers can use CRISPR-Cas9 technology to edit genes that are believed to play critical roles in cancer biology. This allows for studies that can elucidate the functions of specific genes in the context of tumorigenesis.
Key considerations in this area include:
- Gene Knockout Studies: Disabling specific genes in murine embryonic fibroblasts enables researchers to observe changes in behavior, which can shed light on tumor suppressor functions or oncogene activities.
- Overexpression Experiments: Conversely, increasing the expression of certain genes can also alter fibroblast behavior, revealing potential targets for therapeutic intervention.
- Modeling Gene Interactions: By manipulating multiple genes simultaneously, researchers can model the complex genetic landscape of cancer, informing the development of more accurate therapeutic models.
Overall, the genetic manipulation of murine embryonic fibroblasts is a powerful approach that enhances the understanding of cancer biology. By leveraging these experimental models, researchers can progress toward more effective treatments and intervention strategies.
Challenges in Research
Research on murine embryonic fibroblasts (MEFs) presents numerous challenges that can affect the quality and applicability of scientific findings. Addressing these challenges is crucial for researchers aiming to utilize these cells effectively in various applications, including cancer research and regenerative medicine. Understanding the complexities involved helps in optimizing methodologies and refining experiments.
Cell Line Variation and Standardization
Cell line variation is a recognized issue in the field. Many MEF cell lines can show significant differences in their properties, such as growth rates and gene expression profiles. This variability can stem from several factors, including the genetic background of the murine strain, the ways in which cells are cultured, and the duration of subculturing. Therefore, it is essential to establish standard operating procedures that promote consistency.
To counteract these variations, researchers often compare multiple cell lines to identify the most representative ones for their studies. Furthermore, employing strategies like genetic profiling can offer insights into the inherent differences between these lines. Standardization of culturing protocols—such as the use of specific media formulations and growth conditions—also aids in minimizing discrepancies. This ensures that findings are reliable and can be reproduced across different laboratories, which is a fundamental principle of scientific inquiry.
Ethical Considerations
Ethics plays a significant role in research involving murine models. Researchers must navigate a range of ethical considerations when using MEFs. The collection of embryonic fibroblasts involves complex ethical implications, especially as it relates to animal welfare and the use of embryos. Obtaining necessary approvals from institutional review boards and ensuring compliance with regulations is essential before initiating any study.
Moreover, as new techniques, such as genetic editing, evolve, researchers must continuously assess the ethical dimensions associated with such methods. For instance, the use of CRISPR/Cas9 technology raises questions about genetic integrity and potential long-term outcomes of genetic manipulation in experimental models. Engaging in ongoing ethical discourse helps to ensure that the research adheres to established norms while advancing scientific knowledge.
"Ethics must keep pace with scientific advancements to ensure responsible research."
By focusing on these challenges, researchers can develop robust protocols that promote the integrity of their work. Identifying and addressing the intricacies of cell line variation and ethical responsibilities will enhance the credibility, reproducibility, and societal acceptance of findings derived from studies using murine embryonic fibroblasts.
Future Directions in Murine Embryonic Fibroblast Research
The exploration of future directions in murine embryonic fibroblast research is critical for advancing current methodologies and applications. This topic holds immense importance as it can significantly impact areas like regenerative medicine and cancer research. Further development in this field promises to refine the techniques used and enhance the reliability of results.
Research on murine embryonic fibroblasts is not stagnant; instead, it is evolving with innovative techniques being introduced. Moreover, interdisciplinary approaches bring diverse perspectives and methodologies, enriching the study of these cells.
Innovative Techniques and Technologies
Innovative techniques play a pivotal role in studying murine embryonic fibroblasts. One significant advancement is the application of CRISPR-Cas9 gene-editing technology. This method allows precise modifications in the genome of these fibroblasts, which can lead to breakthroughs in understanding genetic disorders. Another emerging technology involves the use of three-dimensional (3D) cell culture systems. Unlike traditional two-dimensional cultures, 3D systems provide a more physiologically relevant environment, enabling better mimicry of in vivo conditions.
Moreover, single-cell RNA sequencing can offer detailed insights into gene expression at the cellular level. By accurately quantifying mRNA transcripts from individual cells, researchers can pinpoint cellular heterogeneity and identify specific subtypes of fibroblasts that may have distinct roles in development or disease.
"The application of advanced technologies is essential for the evolution of murine embryonic fibroblast research. Innovations in gene editing and culture techniques can redefine our understanding of cellular mechanisms."
Interdisciplinary Approaches
Interdisciplinary approaches are becoming increasingly vital in the study of murine embryonic fibroblasts. Collaborations between fields like bioinformatics, molecular biology, and systems biology can illuminate complex biological processes. For example, integrating high-throughput sequencing data with computational analysis helps in understanding cellular dynamics and pathways in fibroblasts.
Incorporating knowledge from engineering can also lead to novel bioreactor systems that provide precise control over the culture environment, leading to improved cell growth and function. Furthermore, partnerships with clinicians can ensure that research is aligned with real-world applications, enhancing the translational aspect of murine embryonic fibroblast studies.
Collaborative efforts are essential to address the multifaceted challenges presented in fibroblast research. Researchers must acknowledge the benefits derived from diverse input, paving the way for innovative solutions and better outcomes.
The End
The importance of the conclusion in this article lies in its ability to synthesize the complex information surrounding murine embryonic fibroblasts and to highlight their significance in various domains of biomedical research. Drawing together the key findings elucidates the multifaceted roles these cells play in cellular behavior, disease modeling, and therapeutic advancements.
Recapitulation of Key Findings
Murine embryonic fibroblasts have emerged as a foundational model for understanding numerous biological processes. Here are the key insights:
- Cellular Characteristics: Murine embryonic fibroblasts exhibit specific gene expression profiles that provide insights into their functionality. Their characteristics allow for extensive applicability in experimental settings.
- Research Applications: They are invaluable in in vitro studies, contributing to breakthroughs in cancer research, regenerative medicine, and stem cell technology. Their ease of manipulation aids in understanding disease mechanisms.
- Technological Integration: Emerging technologies have increased the efficiency of culturing and manipulating these cells. For instance, advanced CRISPR techniques open up new pathways for genetic research that were not previously available.
The synthesis of these findings emphasizes not just their roles in current research but also their potential to unlock new avenues in studying genetic disorders, cancer, and more.
Implications for Future Research
Future research involving murine embryonic fibroblasts is likely to expand significantly, as they offer unique advantages for various applications. Some implications for future studies include:
- Interdisciplinary Collaboration: Scientists from different fields, such as genetics, oncology, and developmental biology, could achieve more comprehensive insights by working together. These collaborations could foster innovative approaches to tackling complex biological questions.
- Enhanced Techniques: The continued refinement and development of culturing and manipulation techniques promise to unlock further applications in regenerative medicine. This could lead to more effective treatments for degenerative diseases.
- Ethical Considerations: As research progresses, ethical considerations surrounding the use of embryonic cells will need close attention. Developing ethical frameworks can ensure responsible research practices while harnessing the benefits of murine embryonic fibroblasts.
Overall, the future of murine embryonic fibroblast research holds great promise. By continuing to examine their properties and applications, researchers can contribute valuable knowledge that may lead to transformative discoveries in healthcare and disease management.