Exploring RP PCR: Techniques and Applications in Science
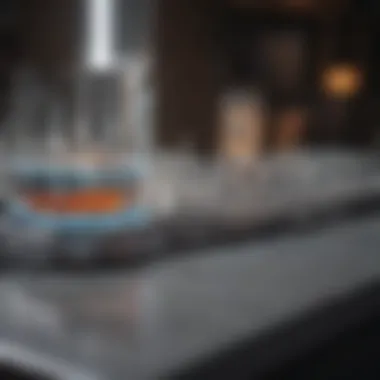
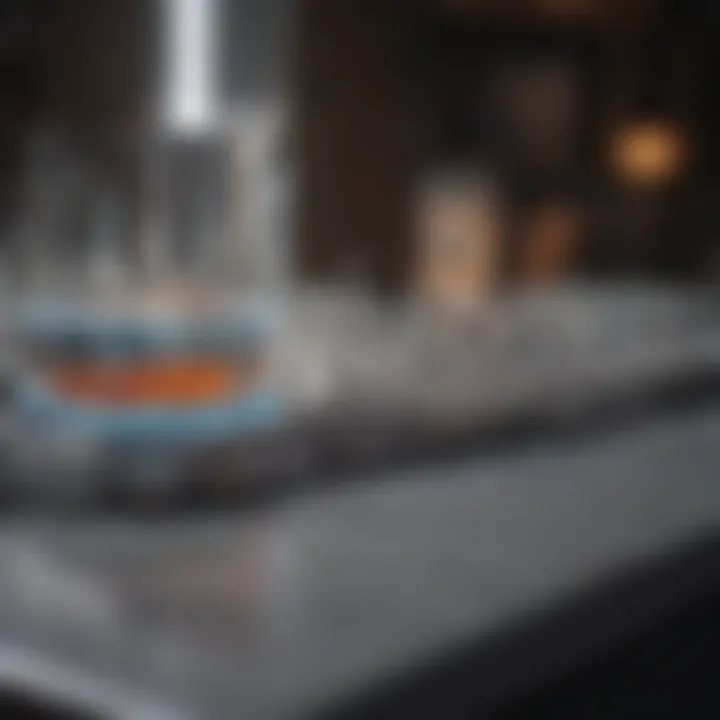
Intro
Reverse transcription polymerase chain reaction (RP PCR) stands as a linchpin in the toolkit of molecular biology. It’s a technique that melds the powers of reverse transcription with polymerase chain reaction, enabling researchers to delve into the nuances of RNA. In essence, it transforms RNA into complementary DNA (cDNA), which can then be amplified. This transformation is not simply a laboratory trick; it lays the groundwork for countless applications in research and diagnostics.
Why RP PCR Matters
The drive to understand genetic expression has pushed RP PCR into the limelight. Traditional PCR primarily focuses on DNA, but since many critical biological processes hinge on RNA, RP PCR fills in the gaps. Its ability to quantify RNA levels opens doors to understanding diseases, developmental biology, and evolutionary studies. As the science pushes forward, so does the tech itself–there are always emerging modalities and methods that promise even greater precision and utility.
A Glimpse into Key Applications
In the realm of diagnostics, RP PCR has made its mark particularly in the detection of viral infections. For instance, the rapid testing of SARS-CoV-2 relied heavily on RP PCR techniques. This application didn't just showcase its clinical relevance but also its adaptability in facing global emergencies. Additionally, the technique finds prominence in research settings, notably in the study of gene expression.
"'Without the integration of RP PCR, many modern molecular biology applications would be severely hindered.'"
As we embark on a detailed exploration of RP PCR, we’ll uncover how specific methodologies and the latest innovations will shape molecular biology's future. Let's delve deeper.
Prologue to RP PCR
Reverse transcription polymerase chain reaction (RP PCR) stands as a cornerstone in modern molecular biology, merging the principles of transcription and amplification. This innovative technique is not merely a variation of traditional PCR; it has evolved to address specific needs in research and diagnostics, particularly in those domains where understanding the transcriptome becomes crucial. The importance of RP PCR can’t be overstated, as it offers a bridge between RNA and DNA analysis, which allows researchers to glean insights that were previously difficult, if not impossible, to acquire.
In recent years, the relevance of RP PCR has surged, driven by its need in gene expression studies, investigations into infectious diseases, and cancer research. By converting RNA into complementary DNA (cDNA), it facilitates the amplification of genetic material that is inherently labile, making the process both powerful and necessary. This section sets the stage for a deeper exploration of RP PCR by establishing fundamental concepts and historical milestones, providing context and clarity behind this essential technique.
Defining RP PCR
Reverse transcription polymerase chain reaction, simply put, is a variation of the polymerase chain reaction (PCR). While standard PCR focuses on amplifying DNA, RP PCR addresses RNA targets. The crux of the technique lies in the reverse transcription step, where RNA strands are transcribed into cDNA using reverse transcriptase. This initial step is pivotal as RNA is not stable like DNA; it degrades easily under various conditions.
Key components of RP PCR include:
- Reverse Transcriptase Enzyme: This enzyme synthesizes complementary DNA from RNA templates, crucial for the process.
- Primers: Short sequences that initiate the amplification of specific regions of the cDNA.
- PCR Cycle: This consists of repeated cycles of denaturation, annealing, and extension, allowing for exponential amplification of the target cDNA.
RP PCR enables researchers to conduct detailed analyses of gene expression, providing a means to understand cellular processes, disease mechanisms, and other biological functions. With the increasing emphasis on transcriptome studies, defining RP PCR has never been more significant for students and professionals in the field.
Historical Context and Development
The inception of RP PCR can be traced back to the early 1990s when scientists began to realize the need for methodologies that could specifically amplify RNA. Until then, conventional PCR was predominantly employed for DNA, leaving the complex world of RNA largely untouched.
One of the groundbreaking developments came with the introduction of reverse transcriptase in the late 1970s. Researchers, including Howard Temin and David Baltimore, were pivotal in showcasing how retroviruses use this enzyme to replicate their RNA genomes into DNA, laying the groundwork for future methodologies.
As research progressed, the first applications of RP PCR started appearing in scientific literature around 1993, focusing primarily on gene expression analysis in various organisms. From there, it quickly branched out into diverse fields such as virology, cancer research, and beyond. The evolution of this technique has shaped our understanding of many biological processes, illustrating how a method born from examining retroviruses burgeoned into a universal tool that aids in numerous scientific inquiries.
Through understanding both RP PCR's definition and its historical trajectory, we can better appreciate its significance in today’s research landscape. The development journey helps highlight how needs in biology and medicine fuel innovations and advancements, making each step forward equally essential.
Principles of RP PCR
Understanding the principles behind reverse transcription polymerase chain reaction (RP PCR) is crucial for grasping how this technique has become a cornerstone in modern molecular biology. At its heart, RP PCR not only amplifies RNA but also provides insights into gene expression levels, making it indispensable for a myriad of applications ranging from academic research to clinical diagnostics. The significance of these principles lies in their ability to bridge the gap between simple DNA analysis and complex RNA profiling, ultimately aiding researchers in unraveling the intricacies of cellular functions.
Molecular Mechanisms Involved
At its core, RP PCR rests upon a set of molecular mechanisms that intertwine to execute the transcription and amplification of RNA. Firstly, it involves the conversion of RNA into complementary DNA (cDNA) using an enzyme called reverse transcriptase. This enzyme synthesizes cDNA from an RNA template through a process reminiscent of traditional DNA synthesis but runs in reverse. Why is this essential? Well, it provides the foundation for understanding how genes are expressed and regulated within cells.
Following this, the cDNA undergoes amplification through conventional PCR methods. This involves cycles of denaturation, annealing, and extension, allowing for exponential growth of the cDNA, which is crucial for detecting low-abundance transcripts.
The specificity of primer design and the particular settings of temperature and timing during the amplification phase can heavily influence the outcome. Any misstep in these parameters could result in non-specific products that muddle interpretation. Thus, a deep understanding of these mechanisms can enhance the reliability and efficacy of the results obtained from RP PCR experiments.
"The precision of RP PCR ensures that even fleeting RNA signals can be interpreted, illuminating previously opaque biological pathways."
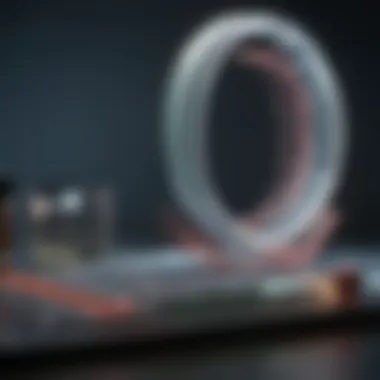
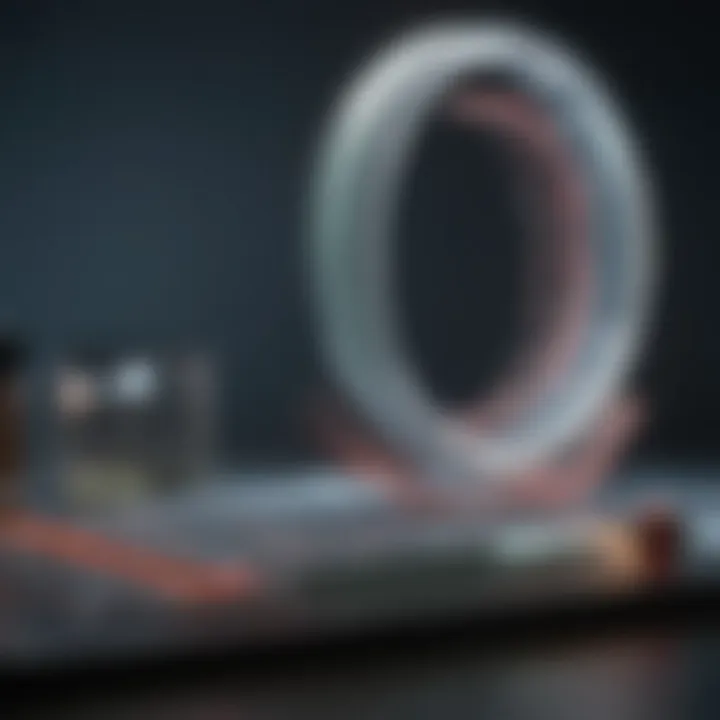
Through these mechanisms, RP PCR dishes out a powerful tool for dissecting the dynamics of gene expression, allowing scientists to explore gene function with unparalleled depth.
Comparison with Traditional PCR
When it comes to comparing RP PCR to traditional PCR, several distinctive factors come into play that underscore why RP PCR has carved out its niche in molecular biology. Traditional PCR primarily amplifies DNA, working from a template of pre-existing genetic material. In contrast, RP PCR uniquely focuses on RNA, necessitating an additional step of reverse transcription before amplification can even begin.
- Starting Material:
- Applications:
- End Products:
- Traditional PCR: DNA
- RP PCR: RNA (transformed into cDNA)
- Traditional PCR: Primarily used for genotyping, cloning, and forensic analysis.
- RP PCR: Essential for investigating gene expression, viral load, and detecting non-coding RNAs.
- Traditional PCR: Amplified DNA fragments for various downstream applications.
- RP PCR: Provides cDNA that can be analyzed for expression patterns, offering insights into temporary gene activities.
Such stark differences highlight why researchers might choose RP PCR over traditional methods when their aim is to illuminate the nuances of RNA biology. Additionally, while PCR often requires longer DNA to amplify specific sequences, RP PCR shines when working with limited or degraded RNA samples, demonstrating its utility in less-than-ideal conditions.
In summary, the principles underpinning RP PCR not only elucidate its mechanisms but also showcase its indispensable role in advancing our understanding of gene expression and regulation.
Methodological Approach to RP PCR
The methodological approach to reverse transcription polymerase chain reaction (RP PCR) is critical to its utility in both research and clinical settings. This section delves into the essential components of the RP PCR process, illustrating how each step contributes to the overall efficacy of this powerful technique. By understanding the nuances of sample preparation, the reverse transcription process, and amplification phase, researchers and professionals can enhance their applications of RP PCR in various domains.
Sample Preparation
Sample preparation forms the bedrock of any successful RP PCR experiment. It involves the careful extraction and purification of RNA from biological specimens such as tissues, blood, or cultured cells. This stage is crucial because the quality and integrity of the RNA determine the accuracy of subsequent analyses.
To begin with, the samples must be handled in a manner that preserves RNA integrity. Researchers often utilize specialized kits designed for RNA extraction, such as the Qiagen RNeasy Mini Kit. Methods typically include mechanical disruption of the samples followed by the use of lysis buffer, which aids in breaking down cell membranes and releasing RNA. Additionally, it's essential to use RNase-free reagents and conditions, as RNases can degrade RNA quickly.
The purification process may include column-based or magnetic bead methods, which help in eliminating contaminants that could inhibit the subsequent reverse transcription. Proper quantification and assessment of RNA quality can be performed using spectrophotometry or gel electrophoresis, ensuring that the sample is suitable for the next steps in RP PCR.
Reverse Transcription Process
Once the RNA is isolated, the next step is the reverse transcription process. This phase converts the extracted RNA into complementary DNA (cDNA), which serves as the template for amplification in the following steps. The enzyme reverse transcriptase plays a pivotal role here—its ability to synthesize cDNA from an RNA template is what sets RP PCR apart from traditional PCR methods.
During this process, researchers typically use a specific oligo(dT) primer or random hexamers to initiate cDNA synthesis. The choice of primer can influence the RNA populations represented in the cDNA, allowing for either selective or broad capture of transcripts. Heating, followed by cooling cycles, optimizes the conditions for primer annealing and cDNA synthesis. This stage usually runs for about 30-60 minutes at 37°C, followed by inactivation of the reverse transcriptase through a brief heat treatment, ensuring a clean transition to the amplification phase.
Amplification Phase
The amplification phase marks the final leg of the RP PCR approach, where the cDNA is exponentially amplified through controlled cycles of denaturation, annealing, and extension. This phase essentially magnifies the cDNA, making it detectable and quantifiable, which is essential for subsequent applications.
In this step, typical PCR reagents come into play: DNA polymerase, primers specific to the target sequence, and nucleotides. The temperature cycles involve raising the temperature to separate the strands of cDNA, lowering it to allow primer binding, and again raising it for extension.
Amplification can be monitored through real-time PCR methods, allowing researchers to quantify gene expression levels effectively. It's essential to maintain optimal conditions during this phase—too high or too low temperatures can lead to inefficient amplification or non-specific products. Researchers often include a standard curve for comparison to ensure accurate quantification of target nucleic acids.
Effective methodological approaches in RP PCR are not merely about the individual steps but also how each interacts to produce robust and reproducible results.
In summary, each segment of the methodological approach to RP PCR plays a pivotal role in the overall success of the technique. From sample preparation to the final amplification phase, understanding these processes helps in overcoming potential pitfalls and achieving accurate, meaningful results in research and clinical diagnostics.
Applications of RP PCR in Research
The significance of RP PCR in the realm of molecular biology cannot be overstated. This technique has transformed the landscape of biological research by facilitating precise detection and quantification of RNA. With its versatility and sensitivity, RP PCR serves as a cornerstone method for many researchers. It helps uncover gene expressions, monitor viral load during infections, and investigate the genetic foundations of various diseases, especially cancer. This section explores its diverse applications, shedding light on why it holds such an esteemed place in research methodologies.
Gene Expression Analysis
Gene expression analysis is one of the most prominent applications of RP PCR. This method allows scientists to measure the amount of specific RNA produced by a gene, offering insights into gene activity under various conditions. By knowing the RNA levels, researchers can ascertain how environmental factors or treatments affect gene expression.
Compared to more traditional methods, RP PCR provides a higher degree of sensitivity and specificity. For instance, if a researcher is looking to analyze cytokine genes that respond to a particular immune challenge, RP PCR can offer quantitative data that helps in understanding the magnitude of the immune response. Furthermore, it enables the comparison of gene expression between different samples, revealing information about differences in tumor and normal tissue.
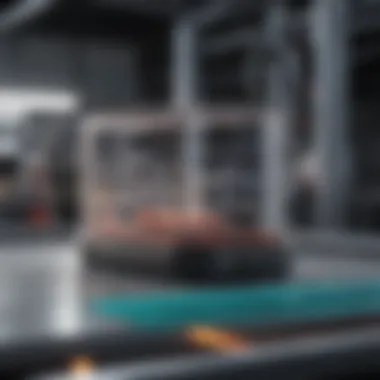
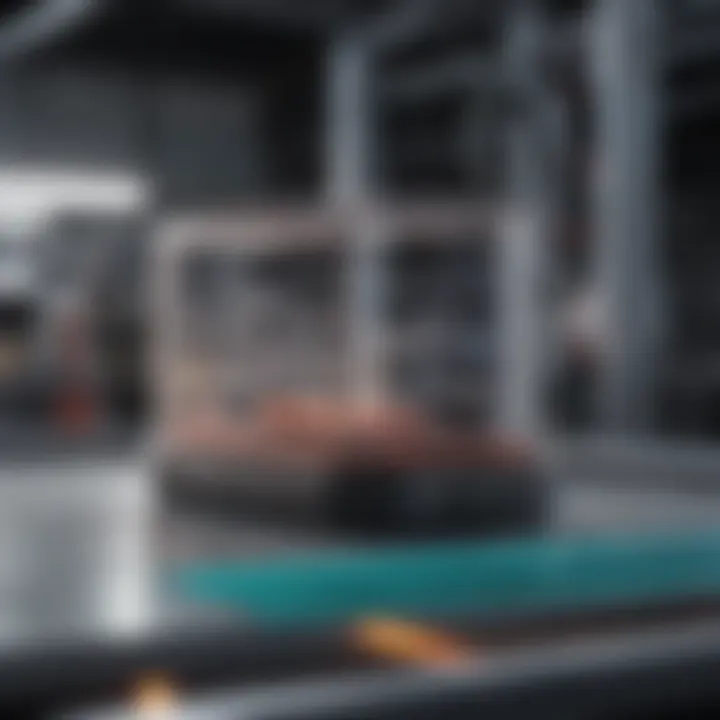
"Gene expression analysis through RP PCR can be likened to tuning into a clear radio station amidst static, allowing researchers to hear the vital signals of cellular behavior."
Detection of Viral Infections
Another critical application of RP PCR lies in the rapid detection of viral infections. This has become exceptionally relevant given the challenges posed by emerging infectious diseases. With the ability to directly amplify RNA from viral pathogens, RP PCR facilitates early diagnosis and helps to initiate timely treatment protocols.
For instance, in the case of HIV, RP PCR is crucial in monitoring the viral load in patients. By enabling detection of low levels of viral RNA, clinicians can assess the efficacy of antiviral therapies and make necessary adjustments to treatment. Moreover, in cases such as COVID-19, detecting the viral RNA in samples took precedence for public health responses, showcasing how pivotal this application of RP PCR is in modern medicine.
Research in Cancer Biology
In the realm of cancer biology, RP PCR emerges as a powerful tool for exploring the molecular underpinnings of tumors. By analyzing the expression levels of oncogenes and tumor suppressor genes, researchers can gather crucial information about the biological mechanisms driving cancer progression.
For example, specific gene patterns can indicate a patient’s prognosis or responsiveness to particular therapies. There are cases where elevated expression of a certain gene correlates with a poorer outcome, prompting the pursuit of targeted therapies aimed at inhibiting that gene's activity. This makes RP PCR not just a method for understanding cancer biology, but also a potential guide for personalized treatment strategies.
The interplay of RP PCR with other innovations, such as next-generation sequencing, brings a depth of analysis that can isolate novel biomarkers for cancer. This can pave the way for breakthroughs in diagnostics and treatment methodologies.
Clinical Applications of RP PCR
The clinical applications of Reverse Transcription Polymerase Chain Reaction (RP PCR) are significant, so they contribute immensely to modern medicine. RP PCR not only aids in diagnosing diseases but also plays a pivotal role in tailoring treatments to meet individual patient needs. Understanding its usage in clinical settings can illuminate how vital this technique is in addressing a variety of health challenges.
Diagnostics in Pathogen Detection
A primary application of RP PCR is its use in diagnosing pathogenic infections. This process is essential because it enables healthcare professionals to detect the presence of viral or bacterial RNA in biological samples swiftly. For example, during the COVID-19 pandemic, RP PCR became the gold standard for confirming SARS-CoV-2 infection. Compared to traditional methods that depend on culturing organisms, RP PCR provides a faster and more accurate diagnostic approach.
- Sensitivity and Specificity: The methods used in RP PCR enhance sensitivity and specificity, allowing for the detection of low levels of viral load that might be overlooked by other techniques.
- Speed: The rapid turnaround time often significantly improves patient management and reduces the risk of transmission, particularly in infectious disease outbreaks.
Moreover, this technique can be tailored to identify emerging pathogens that are not yet well characterized, making it a crucial tool in the realm of personalized medicine. RP PCR's adaptability ensures that healthcare providers have the resources to respond effectively to new threats.
"RP PCR is not just a technique; it's a vital lifeline in diagnosing infectious diseases that can save countless lives."
Role in Personalized Medicine
Another noteworthy role of RP PCR lies within personalized medicine, which emphasizes tailoring medical treatment to the individual characteristics of each patient. By utilizing this technology, clinicians can assess gene expression profiles from RNA samples, which provides deep insight into how a patient’s body responds to specific treatments.
- Targeted Therapies: The analysis of gene expression can guide the use of targeted therapies, particularly in oncology. For instance, in cancer patients, RP PCR can reveal the presence of specific biomarkers that dictate the aggressiveness of the tumor, thereby influencing treatment options.
- Pharmacogenomics: RP PCR also plays a role in pharmacogenomics, where it helps predict how patients will respond to certain medications, reducing adverse effects and enhancing therapeutic effectiveness.
In summary, the clinical applications of RP PCR profoundly impact diagnostics, allowing for speedy detection of pathogens and contributing significantly to personalized medicine. This approach not only provides detailed insights needed for treatment decisions but also aligns with the push towards a more individualized healthcare model.
Challenges and Limitations of RP PCR
Reverse transcription polymerase chain reaction (RP PCR) is a powerful mechanism facilitating insights in molecular biology. However, it’s far from perfect. Understanding its challenges and limitations is crucial for researchers, educators, and students who aim to leverage its applications in meaningful ways. This section examines key technical and interpretational hurdles inherent in RP PCR, offering a transparent view of its limitations as well as potential strategies to navigate them.
Technical Challenges
One fundamental challenge of RP PCR lies in the complexities involved in its technical execution. Here are several critical technical issues encountered in this methodology:
- Efficiency of Reverse Transcription: The reverse transcription step involves converting RNA into complementary DNA (cDNA). Variations in enzyme efficiency, ambient temperature, and RNA quality can lead to inconsistent cDNA yields. If the RNA is degraded or of low quality, it can affect subsequent amplification, leading to not-so-reliable results.
- Contamination Risks: Contamination poses a serious threat in any PCR-based procedure, but in RP PCR, it can severely skew results. Cross-contamination from other samples or the environment could produce false positives, thereby undermining the accuracy of analyses.
- Quantification Challenges: It's not just about getting results. It's about getting them accurately. RP PCR quantification methods can be affected by amplification efficiency, leading to discrepancies in quantifying the original RNA template. This makes it difficult to draw conclusions from the results unless strict controls are implemented.
- Primer Design: The success of RP PCR heavily relies on effective primer design. Poorly designed primers can lead to non-specific binding or failure to anneal at the designated temperature, impacting the overall sensitivity and specificity of the assay.
"Technical rigor is not merely a recommendation, it's a necessity for reliable outcomes."
Interpretational Challenges
Even when the technical challenges are addressed, interpretational challenges still linger, complicating data analysis and conclusions. Here are some salient interpretational hurdles:
- Complex Gene Expression Profiles: RP PCR often evaluates complex gene expression patterns. Analyzing these results requires a sophisticated understanding of molecular pathways and interaction dynamics, which can be convoluted due to the multifaceted nature of gene regulation. Ignoring these complexities might lead readers down the wrong path in their conclusions.
- Basal Expression Levels: Variations in baseline expression levels can obscure meaningful comparisons. Determining what constitutes a significant change versus normal variation can be tricky, particularly in studies addressing subtle expression changes.
- Data Overinterpretation: There’s always a temptation to jump to conclusions, especially when results seem to support a hypothesis. However, the risk of misinterpretation exists, exceeding solid evidence supported by data. It’s important to remember that correlation does not equal causation.
- Statistical Analysis: Understanding results of RP PCR assays often calls for robust statistical analysis. Inadequate statistical treatment of data might yield misleading insights, emphasizing the need for thorough statistical training and understanding among researchers.
By recognizing these challenges, researchers can better prepare for the hurdles they may encounter along the way. Identification of both technical and interpretational obstacles provides a pathway for learning and adaptation, fostering greater confidence in RP PCR as an indispensable tool in modern genetics and molecular diagnostics.
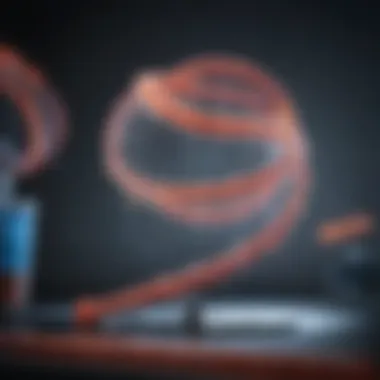
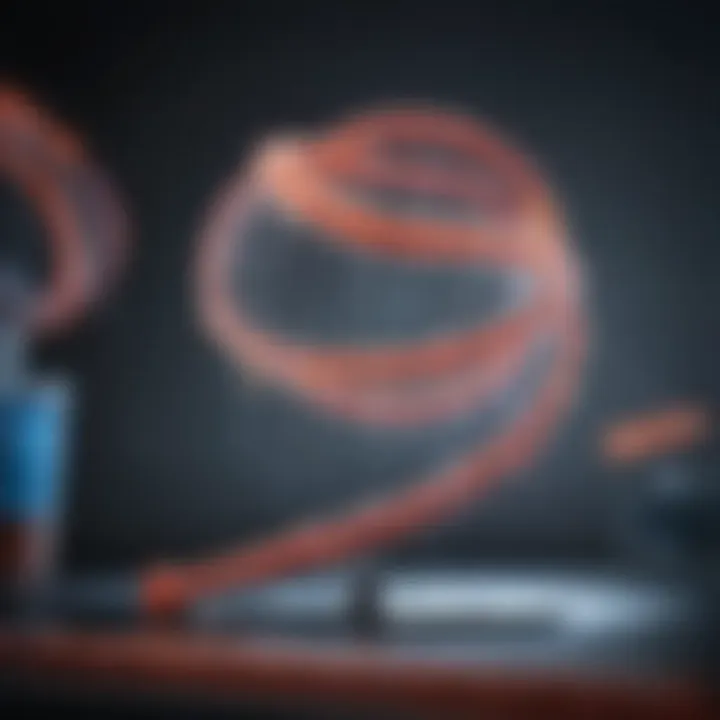
Emerging Techniques and Innovations
The landscape of molecular biology is constantly evolving, and the emergence of innovative techniques in reverse transcription polymerase chain reaction (RP PCR) is at the forefront of this change. These new advancements are essential as they promise to enhance accuracy, efficiency, and overall usability of RP PCR, offering researchers better tools for analysis and application.
As scientific inquiries deepen, the need for sophisticated methodologies grows. Integrating such cutting-edge techniques can lead to significant breakthroughs in gene analysis, diagnostics, and therapeutic strategies. In this section, we will delve into two prominent innovations transforming RP PCR today: Next-Generation Sequencing Integration and Digital PCR Advances.
Next-Generation Sequencing Integration
Next-Generation Sequencing (NGS) is a game-changer in the world of genomics and transcriptomics. Its integration with RP PCR is particularly noteworthy, creating synergistic methodologies that prolong and amplify the benefits of each individual technique. This combination allows for a more detailed understanding of RNA populations, as well as improved quantification of gene expression levels.
One of the crucial advantages of merging NGS with RP PCR is the ability to conduct multiplexing effectively, which significantly reduces the time and cost involved in traditional sequencing approaches. Additionally, this integration enhances sensitivity to detect rare transcripts, which can be pivotal in studying diseases like cancer where early detection is key.
In practical terms, this technique operates by first utilizing RP PCR to convert RNA into complementary DNA (cDNA). Following this, NGS performs a high-throughput sequence analysis of the cDNA. Researchers have reported that this combination not only increases throughput but also improves data reliability, paving the way for more informed decision-making in clinical settings.
"The ability to analyze thousands of genes in a single experiment can unveil insights that were previously unattainable."
Digital PCR Advances
Digital PCR (dPCR) is bringing about another dimension to RP PCR capabilities. Unlike conventional quantitative PCR that relies on a standard curve for quantification, dPCR operates on a partitioning principle. In essence, a sample is divided into thousands of smaller reactions, and positive/negative results are counted digitally. This method provides absolute quantification without the need for external calibration, which offers a level of precision that is increasingly important in research and diagnostic contexts.
The relevance of dPCR in RP PCR applications is clear; it can detect low-abundance nucleic acids with much higher specificity and sensitivity compared to traditional methods. For instance, when studying low-frequency variants in cancer biopsies, a digital approach can significantly improve detection rates, which are critical for accurate diagnosis and subsequent treatment plans.
Furthermore, with the increasing emphasis on personalized medicine, dPCR can assist in determining the precise quantities of therapeutic targets in patients, allowing for tailored therapies that align with individual genetic profiles. This transition from qualitative to quantitative measures can reshape the way treatments are administered, facilitating a more proactive approach to patient care.
Future Directions in RP PCR Research
In the sprawling field of molecular biology, reverse transcription polymerase chain reaction (RP PCR) stands as a crucial methodology. Predominantly, it’s deployed for analyzing RNA, thus playing a vital role in gene expression studies, diagnostics, and even in therapeutic developments. Looking ahead, it’s pivotal to consider where RP PCR might head next.
Potential New Applications
The potential of RP PCR is only just beginning to blossom. The continual advancements in technology and improvements in techniques hint at numerous new applications on the horizon. For instance, one area on the verge of significant exploration is the use of RP PCR in environmental monitoring. Imagine detecting the RNA of pathogens or even microbial communities in natural settings, such as waterways or soil samples. This could revolutionize how we monitor ecosystems and detect changes brought on by climate change or pollution.
In addition to environmental science, there’s an emerging interest in utilizing RP PCR for personalized medicine strategies. The ability to assess an individual's unique RNA profile presents opportunities in customizing treatment protocols for various diseases, including cancer and genetic disorders. Specific biomarkers identified through RP PCR could guide the selection of therapeutic approaches tailored to the patient's unique biological makeup.
"The integration of RP PCR into these novel areas shows that it is not just a technique but a bridge to understanding more about our world and ourselves."
Yet another promising realm is its use in developing advanced vaccines. With the rise of mRNA vaccine technology, RP PCR can serve not only in vaccine validation but also in gauging immune responses in clinical trials. A quicker and more accurate understanding of immune response would be paramount in vaccine development, particularly in the wake of global health crises.
Regulatory and Ethical Considerations
As with any advanced technological approach, one must tread carefully with RP PCR's applications. Regulatory and ethical considerations loom large as this technique expands into new sectors. For example, the deployment of RP PCR in personalized medicine brings forth questions of privacy. The handling of genetic data, especially when linked to individuals’ RNA profiles, demands stringent safeguards to prevent misuse. Who owns this data? How can patients ensure that their information remains secure? Such inquiries must be addressed to maintain trust between the medical community and patients.
Moreover, as researchers explore environmental applications, ethical implications concerning natural ecosystems come into play. Careful consideration must be given to how data gathered from RP PCR is interpreted and utilized. A thorough understanding of ecological balance is critical, ensuring that the pursuit of knowledge does not inadvertently lead to harm.
End
The conclusion of this article encapsulates the profound insights gained from understanding reverse transcription polymerase chain reaction (RP PCR) and its significance in both research and clinical applications. By dissecting the principles, methodologies, and challenges associated with RP PCR, we've shed light on how this technique underpins advancements in molecular biology. Its invaluable role in gene expression analysis and pathogen detection reveals a breadth of possibilities that can influence future scientific endeavours.
Summary of Key Insights
Throughout our exploration, key insights regarding RP PCR have emerged:
- Robust Methodology: The distinct phases of RP PCR, from reverse transcription to amplification, provide a systematic approach to studying RNA. This transformation of RNA to complementary DNA (cDNA) is pivotal for a multitude of applications.
- Diverse Applications: The capability of RP PCR extends beyond basic research to critical clinical uses, including the detection of viral infections and the customization of treatment plans in personalized medicine.
- Advancements in Technology: Integration with technologies like next-generation sequencing has amplified the effectiveness of RP PCR, propelling it to the forefront of molecular diagnostics and research innovations.
- Ongoing Challenges: Despite its benefits, RP PCR does face technical and interpretational hurdles. Addressing these challenges is crucial for standardizing procedures and improving reliability in results.
In short, the amalgamation of theoretical knowledge and practical application emphasizes RP PCR’s role as a cornerstone of modern molecular biology.
The Ongoing Evolution of RP PCR
As we look forward, the ongoing evolution of RP PCR hints at exciting new horizons. Continuous improvements in protocols and technologies will allow for:
- More Accurate Diagnoses: Enhanced sensitivity and specificity in detecting diseases, particularly viral infections, promises to improve patient outcomes.
- Expanded Research Horizons: Novel applications in cancer biology and other fields will likely emerge as scientists develop refined RP PCR methods.
- Ethical Considerations: The growth of this technology brings along regulatory and ethical questions. Ensuring responsible use and maintaining high ethical standards will be paramount in navigating its future.
The transformative potential of RP PCR is undeniable. As we march ahead in the field of molecular biology, this technique will likely open new avenues that could lead to breakthroughs in our understanding of life at the molecular level. With constant innovation on the horizon, RP PCR promises to remain a vital element of biological research for years to come.