Seismic Isolators in Earthquake Engineering Explained
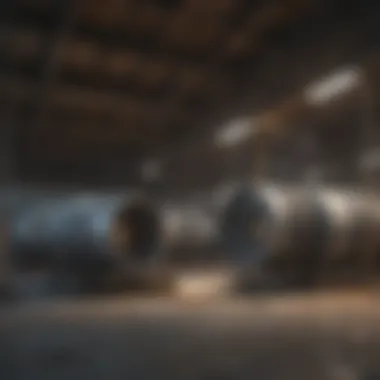
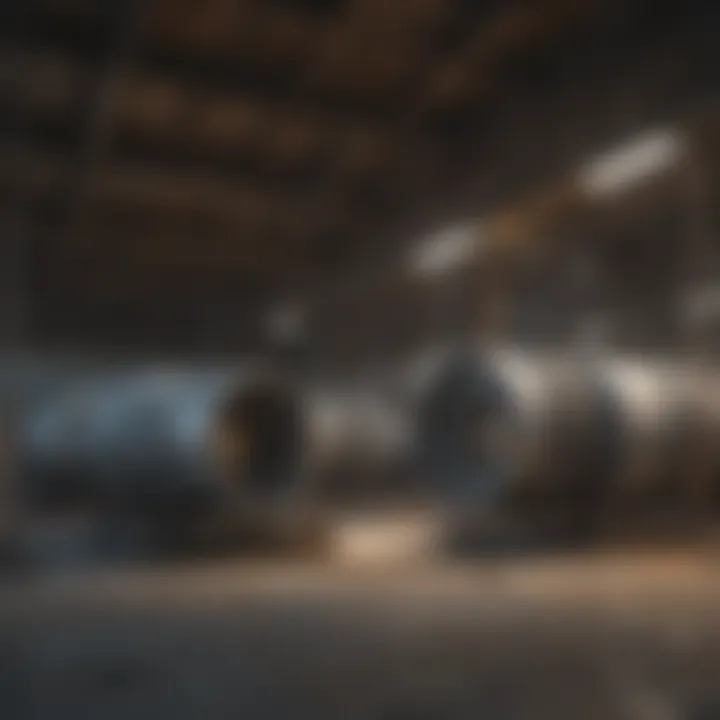
Intro
The necessity for resilient structures in seismically active regions can't be overstated. As our cities grow denser, the potential for significant earthquake damage increases, making effective engineering solutions paramount. This is where seismic isolators come into play, serving as a fundamental component in the design of earthquake-resistant buildings. In essence, they absorb and mitigate the energy released during an earthquake, thus protecting the integrity of the structure and ensuring the safety of its occupants.
By understanding their mechanics and typologies, designers and engineers can enhance the safety of infrastructures. This section will examine how seismic isolators function, offering a lucid overview of their historical evolution, current applications, and future challenges in the field of earthquake engineering.
Foreword to Seismic Isolators
Understanding seismic isolators is like holding a map to navigate the treacherous terrain of earthquake engineering. They play a pivotal role in safeguarding structures in seismically active regions, acting as a buffer between the ground motion and the buildings they support. As the world grapples with the increasing frequency of earthquakes, the significance of these devices becomes more pronounced, making them a cornerstone in modern structural engineering.
When designing structures to withstand earthquakes, the sheer force exerted during such events can be catastrophic. Seismic isolators function by absorbing and dissipating this energy, ensuring that a building remains intact even as the earth shakes beneath it. They allow structures to move independently of ground motion, which can significantly mitigate damage.
Key Elements and Benefits
- Protection: First and foremost, the reduction of seismic forces helps to protect both lives and property.
- Flexibility: Their design can be tailored to accommodate specific site conditions and building requirements.
- Cost-Effectiveness: Although the initial costs may seem steep, the long-term savings in repairs and loss prevention often outweigh the investment.
- Innovative Designs: With advancements in materials and technology, seismic isolators offer more efficient and effective solutions today than ever before.
In exploring the definition and purpose of seismic isolators, one begins to appreciate their material and functional diversity. This leads us to reflect on the historical context of such technologies, which offers insight into why they have become integral to earthquake engineering.
Definition and Purpose
A seismic isolator is a specialized device designed to prevent or reduce the transmission of seismic waves from the ground to a building or structure. These devices achieve this through various mechanisms, which primarily involve decoupling the structure from ground motion.
Simply put, the core purpose of seismic isolators is to enhance the resilience of buildings during earthquakes. By absorbing and dissipating harmful energy, they turn what could be a tragic scenario into a manageable event. The introduction of seismic isolators marks a shift from traditional strategies that relied heavily on rigidity, aiming instead for a dynamic response to seismic challenges.
This dynamic approach allows for a much needed flexibility in design and performance, making buildings safer and more reliable against the forces of nature.
Historical Context
The journey of seismic isolators began in the mid-twentieth century, amid increasing awareness of the destructive potential of earthquakes. Early systems primarily focused on reinforcement and rigidity, but the catastrophic results of major earthquakes in places like San Francisco in 1906 and Tokyo in 1923 underscored the need for more innovative solutions.
By the 1970s, engineering researchers started to explore the concept of base isolation—essentially the bedrock of seismic isolator technology. During these years, various materials were tested, ranging from rubber to bearings, leading to the creation of elastomeric bearings that became widely adopted.
It's noteworthy that even with the advancements, the global acceptance of seismic isolators has varied. Some regions now take these technologies for granted, integrating them seamlessly into their building codes, while others are still catching up. Today, seismic isolators not only represent engineering ingenuity but also a burgeoning field that has evolved with the understanding of seismic risks—a reminder of the constant dance between man-made structures and the natural world.
Types of Seismic Isolators
Understanding the different types of seismic isolators is crucial for engineers and architects engaged in earthquake-resistant design. Each type offers distinct benefits and functionalities, making them suitable for various scenarios. These isolators act as a barrier between the ground motion and the structure, allowing for greater flexibility and safety. In this section, we will delve into three primary types of seismic isolators: elastomeric bearings, sliding bearings, and hybrid systems.
Elastomeric Bearings
Elastomeric bearings are one of the most widely used types of seismic isolators due to their simplicity and effectiveness. These bearings are constructed from layers of rubber typically combined with steel plates. This design enables them to absorb and dissipate energy during an earthquake, significantly reducing the forces transmitted to the structure above.
- Flexibility: The rubber layers allow these bearings to deform, making them highly responsive to seismic movements.
- Durability: Made from advanced materials, elastomeric bearings endure harsh weather conditions and have a long lifespan.
- Cost-Effective: Their manufacturing process is relatively straightforward, which can lower overall project costs.
These bearings are often used in bridges, buildings, and critical infrastructure, where maintaining stability during seismic events is vital. Engineers must consider parameters such as load-bearing capacity and shear resistance when selecting elastomeric bearings for a particular project.
Sliding Bearings
Sliding bearings operate on a different principle compared to elastomeric bearings. These systems utilize a sliding interface to allow the structure to move horizontally without transmitting substantial forces from ground motion. The main component of a sliding bearing is a low-friction surface which facilitates this lateral movement.
- Reduced Base Shear: The ability to slide significantly decreases the base shear forces acting on the building or structure.
- Adaptability: These systems can be engineered for different types of movements, making them suitable for various structural designs.
- Maintenance and Adjustability: Sliding bearings may require regular adjustments and maintenance to ensure optimum performance, particularly in high-use applications.
These isolators are commonly found in high-rise buildings and bridges located in seismically active zones. The design and materials chosen for sliding bearings can vastly affect their performance in a seismic event, making detailed engineering assessments essential.
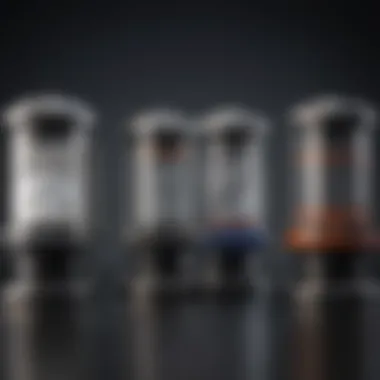
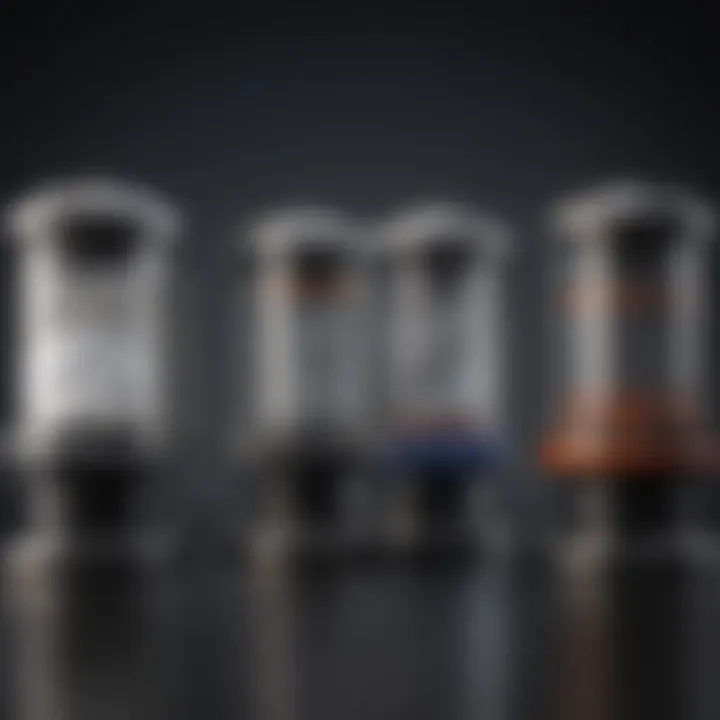
Hybrid Systems
Hybrid systems combine elements from both elastomeric and sliding bearings. This innovative approach aims to leverage the advantages of each type while mitigating their individual drawbacks. Hybrid systems utilize components that allow for both sliding and elastic deformation, thus offering a versatile solution for seismic isolation.
- Enhanced Performance: By integrating the flexibility of elastomeric and the sliding capabilities, these systems can adapt to a wide range of seismic scenarios.
- Complex Design and Engineering: While they provide superior performance, hybrid systems can be more complex to design and implement. Engineers must consider the interactions between components to achieve desired results.
- Potential for Customization: These systems can be tailored according to specific project requirements, offering unique solutions that standard isolators may not provide.
In summary, hybrid systems are gaining traction in modern earthquake engineering for their multifaceted capabilities. As technology advances, the development of hybrid systems may pave the way for new standards in seismic isolations.
"The goal of seismic isolation is to increase the survival rate of both structures and their inhabitants during an earthquake."
Mechanisms of Seismic Isolation
Understanding the mechanisms of seismic isolation is crucial for grasping how these systems safeguard structures during earthquakes. These innovations do not just resemble ordinary components; they function as primary defenders against the violent forces of nature. By absorbing and redirecting seismic energy, seismic isolators play a pivotal role in maintaining the integrity of buildings, bridges, and various infrastructures.
One of the main aspects to consider is how these mechanisms can significantly enhance a structure's resilience. Seismic isolators serve to reduce the amount of energy that travels through a building's framework during an earthquake. They achieve this through various sophisticated designs, primarily focusing on dampening vibrations and decoupling structural responses.
This section will explore two key mechanisms: dampening and energy dissipation, alongside the concept of decoupling structural responses. Each method offers unique advantages, highlighting the intricacies of earthquake-resistant design.
Dampening and Energy Dissipation
Dampening and energy dissipation are two intertwined processes at the core of seismic isolators. When an earthquake strikes, the seismic waves generate vibrations that resonate through a structure, threatening its stability. As these waves approach a building, seismic isolators mitigate their impact by absorbing a portion of the energy and converting it into heat.
Several types of technology come into play here:
- Elastomeric bearings use rubber or polymer materials that deform in response to seismic forces. This deformation dissipates energy, reducing the overall movements within the structure.
- Viscous dampers incorporate fluid-filled cylinders that slow down the vibrations as they move through. By slowing down the oscillations, these dampers prevent excessive deformation of the structural components.
- Friction dampers rely on the friction generated between surfaces to absorb energy and reduce movement.
The benefits of dampening and energy dissipation mechanisms are clear. They enhance safety by:
- Reducing the risk of structural damage
- Protecting lives during seismic events
- Ensuring that buildings can remain operational even after an earthquake
In other words, effectively implemented systems can be the difference between a building that stands tall and one that crumbles under pressure.
Decoupling Structural Responses
Decoupling structural responses refers to the strategy of isolating the building's superstructure from the ground motion. This separation allows the structure to move independently of the seismic forces acting upon it, significantly limiting the transmission of these forces.
Through various designs like base isolators or bearing pads, buildings can effectively skirt around the principal waves that cause the most damage. This mechanism provides a few noteworthy advantages:
- Enhanced flexibility: Structures can sway in response to forces without rigid connections that force them to move together, which can weaken integrity.
- Maintenance of functionality: Many critical facilities, such as hospitals or emergency response centers, can remain operational during and after an earthquake thanks to this technique.
- Vital for taller buildings: In skyscrapers, where lateral movements can cause significant tilt or sway, decoupling is essential for maintaining overall stability.
"Seismic isolators turn the ground's fury into mere ripples, allowing structures to ride the storm with resilience."
Decoupling not only embodies a protective measure but also showcases how engineering can allow buildings to dance with the earth rather than yield to its chaotic rhythms.
In summary, the mechanisms of seismic isolation combine dampening, energy dissipation, and decoupling techniques. These components are not mere theoretical concepts; they play an active role in achieving a safer built environment. By mastering these mechanisms, engineers ensure structures can withstand the unpredictable forces of nature.
Design Considerations
In the realm of earthquake engineering, design considerations serve as the backbone of effective seismic isolation techniques. When engineers embark on designing structures that can withstand the wrath of seismic forces, they must weigh various elements that directly influence a building's stability and resilience to earthquakes. Understanding these considerations is crucial not only for the integrity of the structures but for the safety of their occupants.
Load Constraints
One prominent aspect to consider in seismic isolation design is load constraints. Buildings bear not only their own weight but are also subject to live loads, wind pressures, and lateral forces during events like earthquakes. The isolators are tasked with managing these loads efficiently.
Engineers must calculate the maximum load an isolator can carry while being mindful that the seismic activity could double or even triple the load in certain scenarios. Failure to account for these conditions often leads to catastrophic failures. Using Finite Element Analysis (FEA) tools can significantly help in predicting how various load scenarios will impact the performance of seismic isolators.
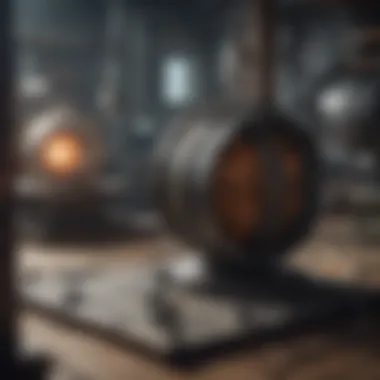
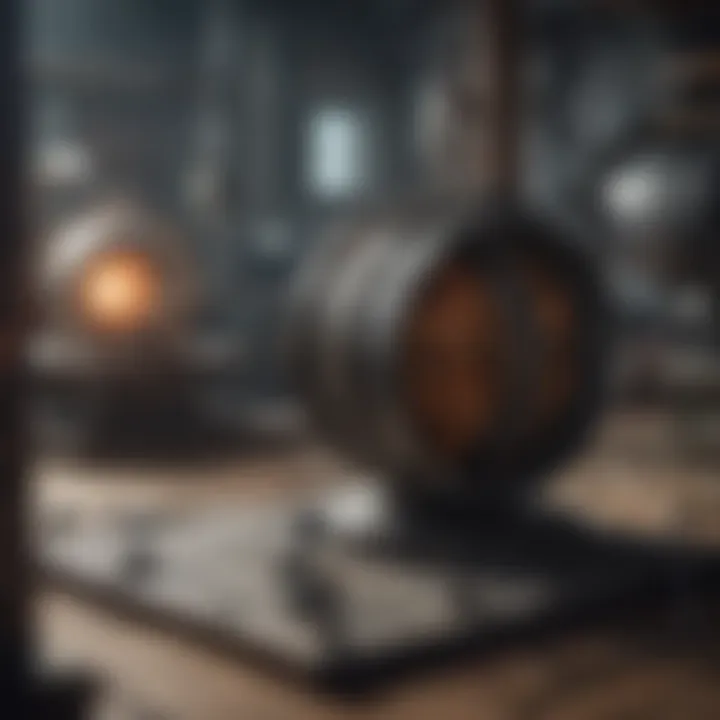
Properly assessing load constraints ensures that seismic isolators function effectively, mitigating potentially devastating structural damage during seismic events.
Geotechnical Factors
Geotechnical considerations relate to the soil and site conditions where a building is to be erected. The soil's properties can dramatically affect how seismic forces impact a structure, thus influencing the selection and design of isolators.
For instance, loose or saturated soils can amplify seismic waves, increasing the forces on a building's foundation. Engineers often engage in detailed soil analyses, testing parameters like shear strength, compressibility, and drainage characteristics. These tests help determine how much settlement or, in some cases, liquefaction may occur during an earthquake, affecting the effectiveness of the isolators. In regions prone to earthquakes, understanding these geotechnical conditions is not just recommended; it’s integral to the design process.
Compliance with Building Codes
Last but certainly not least: compliance with building codes. Building codes exist to set the baseline requirements for structures to ensure safety and durability. In many regions, these codes mandate certain elements of seismic design, including the use and performance of seismic isolation systems.
These codes vary by location and are influenced by factors like historical seismic activity and local government regulations. Engineers must adhere to these codes not only to ensure safety but also to avoid facing legal repercussions or nullified insurance policies. Incorporating current knowledge and best practices into the design process is essential for achieving compliance.
Engineers often participate in continual education and collaboration with regulatory bodies to stay updated on changes in building codes. This engagement fosters innovation and ultimately leads to more resilient structures that can better protect against the forces of nature.
Performance Evaluation
Evaluating the performance of seismic isolators is paramount in understanding their effectiveness within earthquake engineering. This section discusses not only why performance evaluation matters but also how it informs design and implementation processes, ensuring structures can withstand seismic events. By establishing performance benchmarks, engineers can delve deeper into identifying potential weaknesses in the design and execution of seismic isolation systems.
One key benefit of rigorous performance evaluation is the establishment of safety parameters for buildings equipped with seismic isolators. This assessment involves various testing protocols and methodologies that help engineers ascertain how the isolators will behave under different stress conditions. As more data is collected, trends form, allowing for adjustments in design and materials used in future projects.
"The accuracy of performance evaluations lays the groundwork for innovative advances in the earthquake-resistant structures of tomorrow."
Testing Protocols
Testing protocols are the backbone of performance evaluation in seismic isolation systems. These protocols encompass a variety of approaches that simulate real-world seismic conditions, ensuring the isolators will behave as expected during an earthquake.
Dynamic Testing
Dynamic testing uses accelerated simulations to evaluate the response of isolators. Tests often mimic seismic waves, providing immediate feedback about how the isolators will perform. Here are some common methods employed:
- Shake Table Tests: These are widely recognized and involve putting structures on a moveable platform that simulates seismic activity.
- Response Spectrum Analysis: This evaluates how specific frequencies affect the isolator's ability to dampen potential vibrations.
- Cyclic Load Testing: This method applies repeated loads to isolators to determine their durability and endurance under stress.
These testing protocols not only ensure that the isolators meet design criteria but also push the boundaries of what is possible within seismic design. This innovation can lead to enhanced methods for protecting lives and property.
Field Performance Assessments
Field performance assessments provide a practical evaluation of how seismic isolators hold up in real-world conditions, post-installation. This on-site evaluation is just as critical, if not more so, than testing completed in laboratories.
Post-Earthquake Investigations
After an earthquake, engineers often conduct assessments to determine how well the seismic isolators performed. This includes:
- Observation of Damage: Checking both the isolators and the superstructure to identify signs of stress or failure.
- Performance Metrics: Analyzing data to measure how much energy was absorbed and how effectively it mitigated movements.
- Feedback for Design Improvements: This aspect allows engineers to refine their designs and engineering equations based on real-world performance.
Collectively, these assessments bridge the gap between theory and practice. Not only do they reaffirm the reliability of existing systems, they also open the door for future advancements in earthquake-resistant technology. Coupling extensive testing with ongoing field evaluations contributes substantially to optimizing seismic isolators and increases overall safety in seismic-prone areas.
Applications in Earthquake Engineering
In the realm of earthquake engineering, the deployment of seismic isolators plays a pivotal role. These devices, designed to absorb and mitigate the lateral forces generated by seismic events, are essential in protecting structures from the destructive impacts of earthquakes. The application of seismic isolators is not solely about resilience; it also concerns the operational continuity of key infrastructures, economic viability, and the safety of inhabitants. By decoupling a building's structure from ground motion, these isolators ensure that even during tremors, the integrity of the structure remains intact.
The importance of understanding where and how to apply seismic isolators cannot be overstated. Their strategic positioning can significantly enhance a structure’s performance during seismic activities, minimizing damage and ensuring that it can function post-event. An investor's perspective on safety and durability can become a selling point in regions prone to earthquakes. As such, these applications merit thorough exploration and analysis.
Strategic Locations for Use
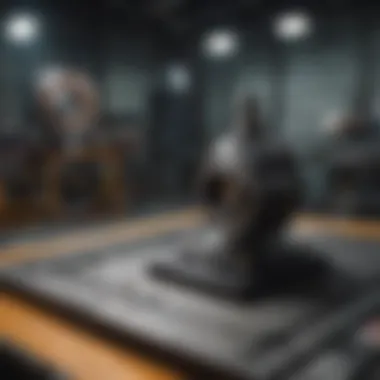
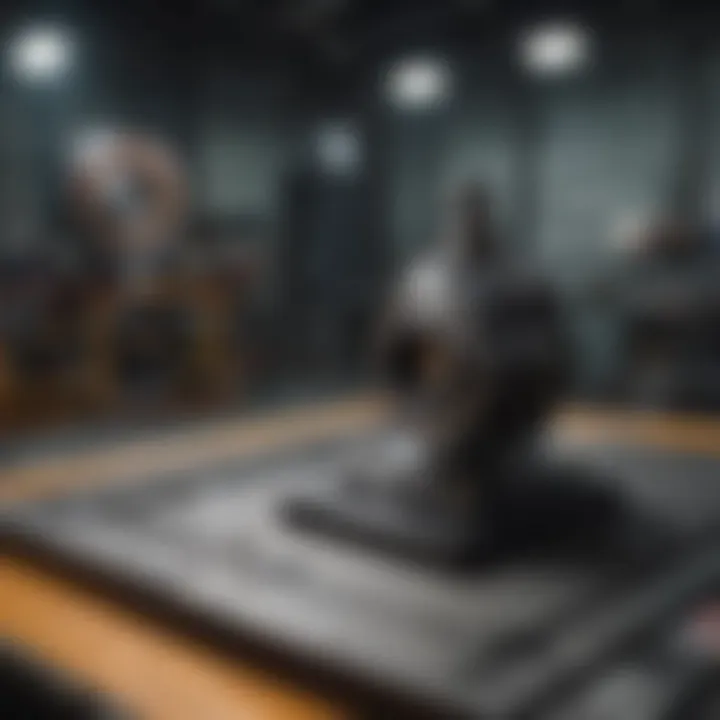
Identifying optimal locations for the implementation of seismic isolators is crucial. Numerous factors inform these decisions, ensuring efficacy in real-world scenarios. Here are some considerations that influence strategic placement:
- Urban Areas: In densely populated regions, the importance of minimizing damage is vital. High-rise buildings and critical infrastructures like hospitals and emergency response centers often integrate seismic isolators. Their placement reduces not only structural damage but also the risk to human lives, ensuring that these crucial facilities remain operational during and after an earthquake.
- Critical Infrastructure: Bridges, railways, and dams are a few examples of structures where seismic isolators can make a marked impact. These facilities must continue to operate flawlessly even in the aftermath of a seismic event, and isolators help achieve this by reducing vibrations that can cause failures.
- Historical Buildings: Preservation is key when it comes to cultural heritage. Integrating seismic isolators into older buildings allows them to withstand tremors, minimizing damage while maintaining their architectural integrity. This application reflects a harmonious blend of modern technology and historical preservation.
In summary, the strategic application of seismic isolators is not just a technical decision; it's an essential aspect of urban planning that directly addresses safety and functionality in seismic-prone areas.
Case Studies of Successful Implementations
Learning from real-world applications provides invaluable insights into the effectiveness of seismic isolators. Here are a few compelling case studies:
- The San Francisco Transbay Transit Center: This facility features state-of-the-art seismic isolators designed to protect it against the devastating effects of earthquakes. The center has successfully withstood seismic tests, reinforcing the importance of isolators in modern infrastructure.
- Tokyo Sky Tree: One of the tallest structures in the world, the Tokyo Sky Tree, employs innovative seismic isolation technologies. Located in a high-risk seismic zone, its design incorporates multiple layers of dampening systems, including base isolators. The structure's resilience showcases the potential of seismic isolators in tall buildings, ensuring safety and stability.
- Christchurch Town Hall: After the 2011 earthquake in New Zealand, the Christchurch Town Hall underwent extensive retrofitting, including the installation of seismic isolators. This project demonstrated how existing historical buildings could be retrofitted effectively, allowing them to be safeguarded against future seismic events without sacrificing their historical value.
These case studies illustrate the vital role that seismic isolators play in both modern constructions and redevelopment of historical sites. They highlight how successful implementation can ensure the longevity and safety of structures in earthquake-prone areas, reaffirming the importance of integrating such systems into engineering practices.
Limitations and Challenges
Understanding the limitations and challenges of seismic isolators is fundamental to grasping their role in earthquake engineering. While these devices are indispensable for reducing the impact of seismic forces on structures, they do come with specific restrictions that can affect their performance and deployment.
Technical Restrictions
Technical restrictions surrounding seismic isolators often stem from material limitations and design constraints. For instance, elastomeric bearings, while effective, might experience degradation over prolonged periods or under extreme loading conditions. Factors such as temperature, humidity, and exposure to corrosive agents can significantly affect the lifespan and functionality of these isolators.
Further, the performance of sliding bearings can be compromised if not carefully calibrated. The friction behavior between sliding surfaces might change over time, leading to unpredictable outcomes in real-world seismic events.
- Material Degradation: Different materials exhibit varied resistance to environmental effects. For example, rubber can deteriorate due to UV rays, affecting its elasticity and, in turn, its isolating ability.
- Calibration Challenges: Proper installation and maintenance are critical. Any misalignment or improper setup might cause these isolators not to perform effectively during an earthquake.
- Dynamic Response Variation: The dynamic behavior of structures equipped with seismic isolators can exhibit complexities that aren’t thoroughly understood. This means engineers must constantly reassess how these isolators effectively mitigate seismic energy.
"An effective seismic isolator is only as good as its least optimum component. Choices made in the design and materials heavily influence overall performance."
Economic Considerations
The economic considerations involved in using seismic isolators are multifaceted. While the initial investment in advanced seismic isolation systems might be considerable, the long-term benefits often overshadow these costs. However, challenges arise in budgeting, especially for projects in regions where earthquakes are less frequent, leading some decision-makers to question the necessity of these systems.
- Initial Costs: The upfront costs can deter stakeholders, particularly in regions where seismic activity is rare. The perception that these isolators are non-essential leads many to compromise on safety measures.
- Maintenance and Replacement Costs: Regular inspection and potential replacement of seismic isolators can add to ongoing expenses. The need for specialized knowledge in maintaining these systems can further elevate costs.
- Cost-Benefit Analysis: Understanding the true value of seismic isolation technology requires a broader perspective on loss prevention. Structures without adequate protection can incur massive financial losses if an earthquake compromises them.
In essence, the limitations and challenges of seismic isolators, both technical and economic, warrant careful consideration. Addressing these challenges enhances the effectiveness of seismic isolation technologies and contributes positively to overall structural integrity in the face of natural disasters.
Future Directions in Seismic Isolation Technology
As we move deeper into the 21st century, the field of earthquake engineering continues to evolve in response to the increasing demands of urbanization and the growing awareness of seismic risks. The importance of exploring future directions in seismic isolation technology cannot be overstated. This exploration is critical not only for enhancing the performance of structures during seismic events but also for incorporating innovations that could make these systems more efficient and accessible.
Seismic isolators have significantly advanced over the decades, but as technology transforms, so too do the opportunities for improvement. This section focuses on two key areas: the innovation in materials and design, as well as the integration with smart technologies. These aspects are pivotal for advancing seismic isolation systems.
Innovation in Materials and Design
Innovative materials play a vital role in the performance and longevity of seismic isolators. New material developments are transforming how these devices are designed and function. For example, researchers are experimenting with high-damping rubbers, which can absorb more energy than traditional materials, leading to better overall performance during earthquakes. Lightweight composite materials are also coming into play, allowing for highly effective isolators that are easier to install and maintain.
Moreover, the design of isolators is also evolving. New methodologies are being employed to create adaptable systems that can be customized based on specific site conditions and building needs. This adaptability ensures that structures can withstand various levels of seismic activity.
Furthermore, the use of computational simulations and advanced modeling techniques is enabling engineers to fine-tune designs before they are even built, thereby reducing the risk of failure during real occurrences. The integration of sustainable practices into the design processes is another trend. Eco-friendly materials and solutions not only help mitigate the carbon footprint but also ensure resilience against future demands.
"The future of seismic isolators hinges on creative material applications and innovative design frameworks that prioritize both performance and sustainability."
Integration with Smart Technologies
The emergence of smart technologies presents another frontier for the evolution of seismic isolation. By integrating sensors and monitoring systems directly into isolators, engineers can collect real-time data on the performance of these systems during seismic events. This data is invaluable, as it allows for immediate assessments of structural integrity and can inform future design improvements.
Additionally, these smart systems can be linked to building management systems, which can automatically respond to seismic activity. For instance, upon detecting tremors, a building’s systems might engage emergency protocols, such as shutting off gas lines or adjusting the settings of elevators to prevent them from becoming hazards during an earthquake.
Moreover, artificial intelligence can be leveraged to analyze collected data and predict potential failure patterns or necessary maintenance needs. This proactive approach could enhance safety and save costs over time, allowing for timely interventions and repairs before issues escalate.
In summary, the future of seismic isolation technology appears promising, driven by innovations in materials and the exciting integration of smart technologies. These advancements hold the potential to significantly enhance the effectiveness of seismic isolators and, consequently, the resilience of our built environments against the ever-present threat of earthquakes.