Differentiating SEM and TEM Microscopy Techniques
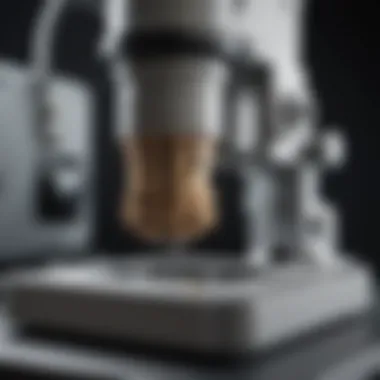
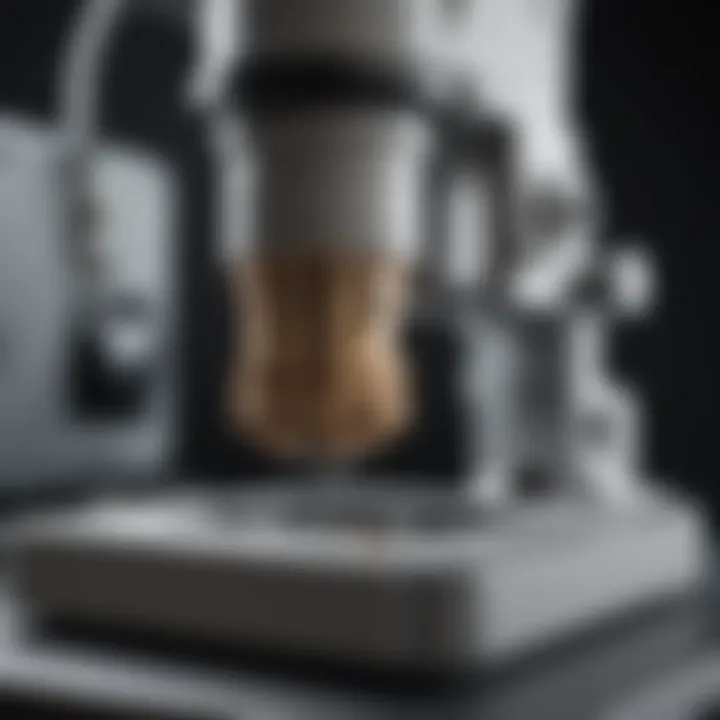
Intro
In the field of microscopy, scanning electron microscopy (SEM) and transmission electron microscopy (TEM) stand out as critical techniques for those studying materials at the nanoscale. Both methods are pivotal in fields such as materials science, biology, and nanotechnology, yet they operate on very different principles and serve distinct purposes. This article will delve into the nuanced distinctions between these two microscopy techniques, shedding light on how each contributes uniquely to scientific exploration and material characterization.
While SEM is primarily used for surface imaging, providing topographical and compositional information, TEM offers insights into the internal structure of samples at an atomic level. The differences between these methodologies can significantly affect experimental outcomes and interpretations in various research scenarios. Understanding these differences is paramount for researchers and students who seek to leverage these tools effectively in their work.
The aim here is to dissect these techniques not only in terms of their functioning principles but also in terms of their applications and inherent advantages. By the end of this article, readers will gain a comprehensive understanding of both SEM and TEM, as well as their roles in modern scientific research.
The forthcoming sections will present a structured discussion starting from foundational concepts through to applications, guiding the reader through the complexities of these micrsocopy methodologies.
Prelims to Electron Microscopy
Electron microscopy encompasses powerful techniques that utilize electron beams to visualize specimens at unprecedented resolutions. This article delves into the critical comparisons between scanning electron microscopy (SEM) and transmission electron microscopy (TEM), two methodologies that have revolutionized the field of materials characterization. Understanding the essentials of electron microscopy is vital for those engaged in advanced scientific research.
The importance of this introduction lies in its foundational role in preparing the reader to grasp more complex themes later in the article. By explaining basic concepts and principles, we aim to enhance the reader’s comprehension of how SEM and TEM operate and their differing applications. This insight is essential for students, researchers, educators, and professionals who seek to utilize these techniques effectively.
Furthermore, this section will address specific elements such as the evolution of electron microscopy, its technical requirements, and its integration into various scientific fields. As technology progresses, the importance of electron microscopy continues to grow, broadening its application in nanotechnology, biology, and materials science. These factors underscore the importance of this comprehensive analysis, which will detail the strengths and weaknesses of both SEM and TEM.
Overview of Electron Microscopy
Electron microscopy differs fundamentally from light microscopy by virtue of its utilization of electrons instead of photons to illuminate samples. The shorter wavelengths of electrons allow for much higher resolution imaging, making it possible to visualize small structures such as organelles within cells or nanoparticles in materials. SEM typically provides three-dimensional images of surfaces, while TEM allows for the investigation of internal structures at the atomic level.
Seminal advancements in instrumentation have contributed to the broad utility of these techniques. For instance, the development of field emission guns has improved the brightness of electron sources, thereby enhancing imaging capabilities. The types of electron microscopes available include benchtop models for SEM and more complex setups for TEM that require significant sample preparation. As such, the choice of technique often depends on the specific requirements of the investigation conducted.
Significance in Scientific Research
The significance of electron microscopy in scientific research cannot be overstated. This technology facilitates investigations at nanoscale dimensions, allowing researchers to uncover details that were previously unattainable. Its application extends across diverse fields, including material science, biology, and nanotechnology, driving innovation and discovery.
"Electron microscopy is not just about resolution. It’s about giving insights into the structure and function of materials at the atomic level."
In material science, electron microscopy enables the analysis of microstructural properties, which are crucial for developing new materials with superior qualities. In biological research, electron microscopy allows for the examination of cellular structures, giving insights into complex phenomena like virus-host interactions. In the realm of nanotechnology, it paves the way for the fabrication and characterization of nanoscale devices.
Moreover, as scientific inquiries evolve, the need for precise imaging techniques will likely remain paramount. By integrating electron microscopy with other analytical methods, researchers can obtain multidimensional data sets that enhance understanding and spark further innovation. The present analysis aims to lay the groundwork for such integrations by thoroughly delineating the roles and capabilities of SEM and TEM.
Principles of Scanning Electron Microscopy
The principles of scanning electron microscopy (SEM) constitute a vital component of the overall understanding of electron microscopy techniques. SEM allows researchers to visualize surface morphology and composition at the nanoscale. This section will clarify the mechanisms of image formation, outline the advantages of SEM, and discuss its limitations.
Mechanism of Image Formation
Scanning electron microscopy operates by directing a focused beam of electrons onto a sample. The interaction of the electron beam with the sample generates signals that are collected to form an image. When the high-energy electrons strike the surface of the specimen, they cause the emission of secondary electrons. The intensity of these emitted electrons varies with the topography of the surface.
A crucial aspect of SEM is how the images are created. A detector registers these signals, allowing for the construction of an image that reflects the surface characteristics of the sample. The images produced are typically in grayscale, as colors are not inherent to the electron signals, but they can be enhanced digitally during analysis.
Advantages of SEM
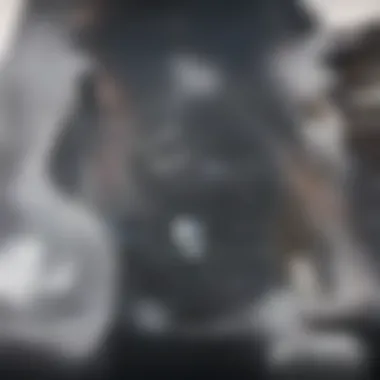
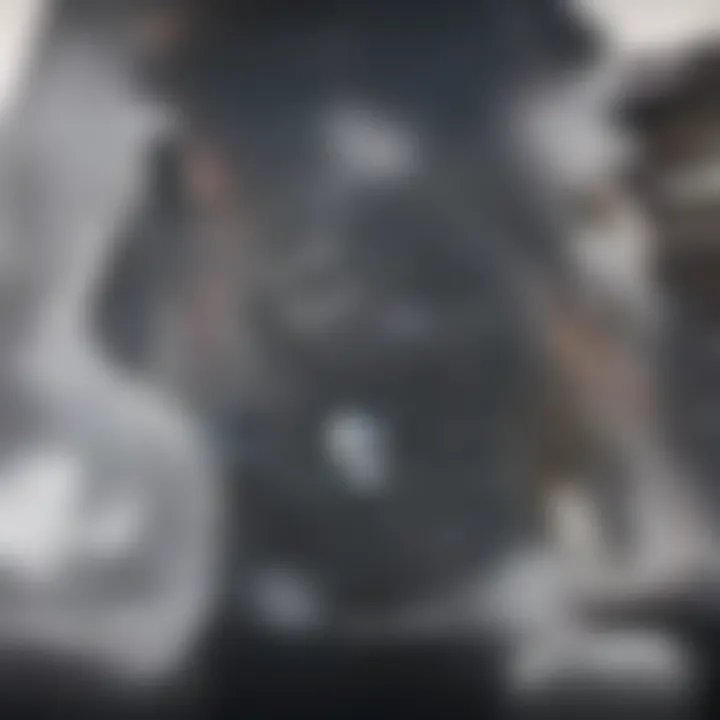
Scanning electron microscopy offers several benefits that make it preferable for certain applications:
- High Depth of Field: SEM provides a greatly enhanced depth of field compared to optical methods. This means that more of the sample can be in focus simultaneously, allowing for better 3D visualization.
- Surface Characterization: The ability to analyze surface structures and features makes SEM especially valuable in material science and semiconductor research.
- Elemental Analysis: With the integration of energy dispersive X-ray spectroscopy (EDS), SEM can characterize the elemental composition of materials. This function is critical for determining material properties and assessing quality control.
- Rapid Imaging: SEM can produce images quickly, facilitating fast assessments of sample characteristics without extensive preparation time.
These advantages highlight why SEM is frequently used in various scientific disciplines including materials science, biology, and nanotechnology.
Limitations of SEM
While SEM presents many benefits, it is not without its drawbacks:
- Sample Preparation: Samples often require extensive preparation to avoid damage and to ensure adequate conductivity. Non-conductive samples may need to be coated with a thin layer of conductive material.
- Penetration Depth: The information obtained from SEM is mainly confined to the surface of the material. Thus, sub-surface features may remain obscured.
- Vacuum Requirement: The need for a high vacuum during operation limits the types of samples that can be examined, especially biological specimens.
- Cost of Equipment: High-quality SEM machines can be expensive to procure and maintain, which can limit access for some researchers or institutions.
In summary, the principles of scanning electron microscopy are fundamental to its application and effectiveness in scientific research. Understanding the mechanism of image formation, recognizing its advantages, and acknowledging its limitations help contextualize SEM in the broader landscape of microscopy techniques.
Principles of Transmission Electron Microscopy
Transmission Electron Microscopy (TEM) is a critical technique in the realm of electron microscopy. Its significance cannot be understated, especially in fields that demand high-resolution imaging and detailed structural analysis at the nanoscale. TEM operates on a distinct set of principles that govern how images are formed and analyzed. Understanding these principles lays the foundation for appreciating the applications and implications of this technique. The following sections explore the mechanism of image formation, advantages, and limitations associated with TEM.
Mechanism of Image Formation
The mechanism by which TEM generates images is intricate yet fascinating. In TEM, a beam of electrons is transmitted through an ultra-thin sample. This beam passes through the material and interacts with it, leading to various scattering events. The electrons that pass through unscattered contribute to the formation of an image.
Key factors in this imaging process include:
- Electron Source: A focused beam originating from an electron gun.
- Sample Thickness: Samples must be sufficiently thin, typically less than 100 nm, to allow electrons to pass through without significant scattering.
- Condenser and Objective Lenses: These lenses focus the electron beam onto the sample and form magnified images from transmitted electrons.
- Detection System: A phosphorescent screen or a digital camera captures the resultant images.
This process results in high-resolution images that can reveal fine details about the material's internal structure, such as defects or crystallographic orientations. The extraordinary resolution of TEM can reach up to 0.1 nm, allowing researchers to visualize atomic arrangements.
Advantages of TEM
The advantages of Transmission Electron Microscopy make it an invaluable tool in scientific research. Here are some of the most noteworthy benefits:
- High Resolution: TEM provides some of the highest spatial resolutions among imaging techniques, which is essential for nanoscale investigation.
- Material Characterization: It offers detailed insights into the morphological and structural attributes of materials.
- Chemical Analysis: Through techniques like Energy Dispersive X-ray Spectroscopy (EDX), TEM can provide elemental composition data of the sample.
- Dynamic Studies: Some advanced TEM models allow for real-time observation of dynamic processes, such as phase transitions.
These advantages illustrate TEM's position as a versatile instrument in various fields, including materials science, biology, and nanotechnology.
Limitations of TEM
Despite its benefits, TEM also has limitations that researchers must consider:
- Sample Preparation: The necessity for ultra-thin samples can pose significant challenges. Improper sample preparation may result in artifacts that compromise the image quality.
- High Vacuum Requirement: The operational requirement for a vacuum environment may limit the types of samples that can be analyzed. Samples that outgas, such as certain polymers, can interfere with imaging.
- Cost and Complexity: The cost of TEM instruments and their operation is relatively high. Training personnel to operate these devices proficiently is also necessary, making it less accessible.
- Limited Field of View: TEM typically has a smaller field of view compared to other imaging techniques, which may hinder the analysis of large area samples.
Comparative Analysis of SEM and TEM
The comparative analysis of scanning electron microscopy (SEM) and transmission electron microscopy (TEM) is crucial for understanding their respective roles in scientific research. By examining the nuances between these methods, readers can appreciate which technique best serves specific types of investigations. This analysis aids researchers in selecting the appropriate method based on the demands of their work, whether it be in material science, biological investigations, or nanotechnology.
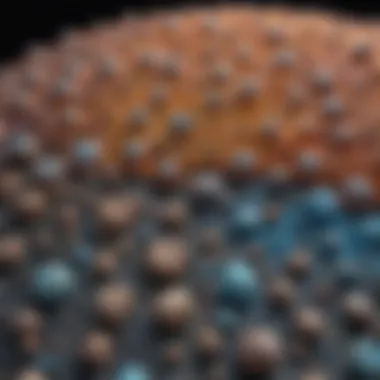
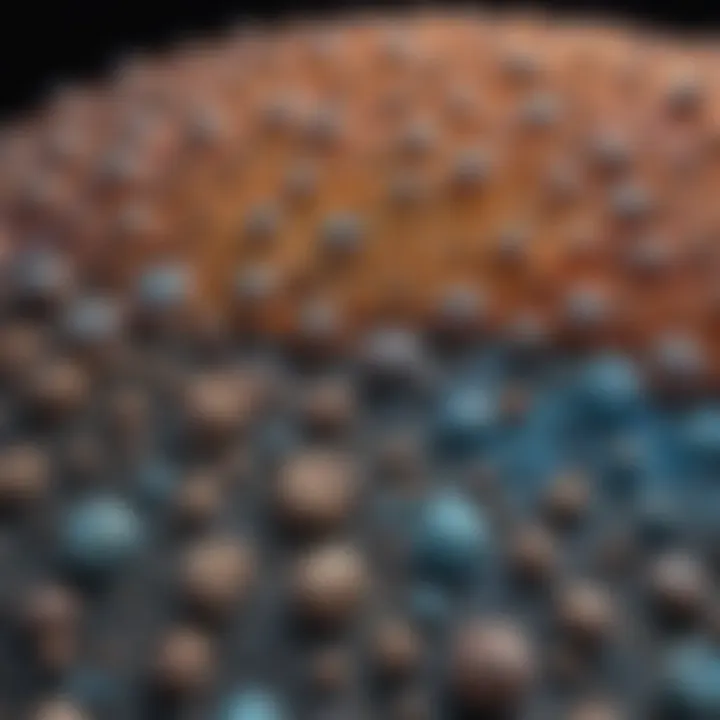
Resolution and Magnification Capabilities
Resolution is a defining factor when choosing between SEM and TEM. SEM typically offers lower resolution than TEM, as SEM generates images from electron interactions on the surface of samples. In contrast, TEM provides much higher resolution, allowing detailed observations at the atomic level. The ability of TEM to visualize finer features in specimens makes it an essential tool in fields like crystallography and nanotechnology.
Magnification capabilities also differ markedly between the two methods. SEM can achieve a maximum magnification of around 1,000,000 times, which is formidable for surface imaging. However, TEM can reach magnifications upwards of 10,000,000 times. This stark contrast showcases TEM’s superiority in applications where atomic structures or nanoparticle distributions must be discerned with great precision.
Sample Preparation Techniques
The procedures involved in preparing samples for SEM and TEM significantly affect the results obtained. For SEM, samples can often be analyzed in their natural state. This reduces the complexity of sample preparation. Nevertheless, some conductive coating may be necessary for non-conductive samples to prevent charging effects during imaging.
Conversely, TEM necessitates a more intensive preparation process. Specimens must be incredibly thin, typically less than 100 nanometers, to allow electrons to transmit through them. This requirement means the preparation of samples is labor-intensive and time-consuming. Techniques like ultra-microtomy or ion milling are commonly used to achieve these thin sections. Thus, the preparation process contributes directly to the application suitability of each microscopy method.
Imaging Modes and Contrast Mechanisms
Imaging modes in SEM and TEM also depict their essential differences. SEM generally operates in secondary electron mode, highlighting topographical features and yielding three-dimensional perspective images. This mode enhances the visualization of surface texture and morphology.
In contrast, TEM can function in multiple modes, including bright field, dark field, and high-resolution imaging. The contrast mechanisms in TEM involve differences in electron scattering. For instance, bright field imaging relies on transmitted electrons, while dark field imaging utilizes scattered electrons to provide contrast. These diverse imaging modes enable TEM to analyze materials on various levels, from surface morphology to internal structure, making it adaptable to diverse research scenarios.
"The selection between SEM and TEM can fundamentally change the insights obtained from material studies. Understanding their comparative strengths is key to advancing scientific discovery."
In summary, the comparative analysis of SEM and TEM reveals distinct advantages and limitations of each. Understanding resolution, magnification, sample preparation, and imaging modes equips researchers with the knowledge necessary to choose the appropriate microscopy technique for their needs.
Applications in Various Scientific Fields
The applications of scanning electron microscopy (SEM) and transmission electron microscopy (TEM) are extensive across many scientific disciplines. Understanding how these microscopy techniques are utilized is crucial for researchers and professionals seeking to enhance their studies and findings. Both methods provide distinct benefits that cater to specific needs in scientific investigations. Research in fields like material science, biological research, and nanotechnology greatly benefits from the unique capabilities of SEM and TEM.
Material Science
In material science, SEM and TEM serve as invaluable tools for analyzing the microstructure of materials. SEM is often preferred for its ability to provide detailed three-dimensional representations of surface morphology. Researchers can observe features such as grain structure, defects, and surface topography with high resolution.
On the other hand, TEM is essential for understanding the internal structure of materials at the atomic level. Applications include:
- Characterizing new materials: Researchers can assess the crystallinity and phase of materials, integral for developing new high-performance materials.
- Studying composites: The interaction between different phases in composite materials can be effectively analyzed with TEM.
Both techniques complement each other, providing holistic insights into materials' properties.
Biological Research
Biological research utilizes SEM and TEM to investigate the intricate details of biological specimens. SEM reveals surface structures of cells and tissues, providing a comprehensive view without requiring extensive sample preparation. This technique is useful for examining:
- Cell morphology: SEM allows for the observation of cell shape, surface features, and interactions between cells.
- Tissue architecture: The three-dimensional structure of tissues can be assessed, leading to better understanding in fields like histopathology.
TEM, however, is critical for examining the internal cellular structures, such as organelles. Key applications include:
- Ultrastructure analysis: Researchers can explore the arrangement and function of internal cellular components using high-resolution images.
- Virus and microbial studies: TEM enables visualization of viruses and bacteria, facilitating insights into their structures and potential interactions.
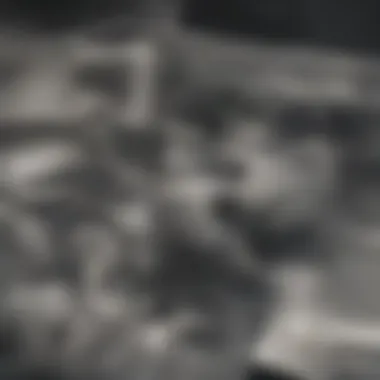
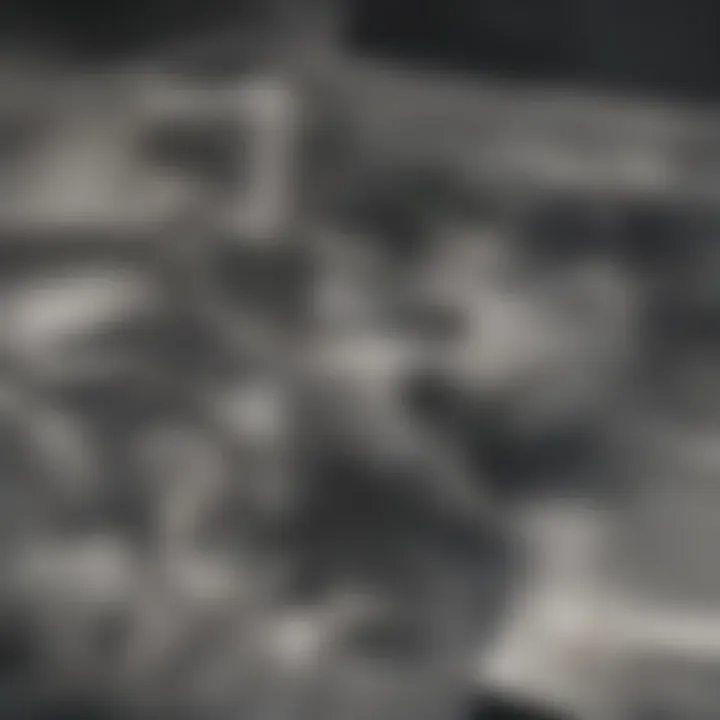
Both SEM and TEM play crucial roles in advancing knowledge in biological sciences.
Nanotechnology
Nanotechnology is another field where SEM and TEM excel. Their unique capabilities allow scientists to manipulate and analyze materials at the nanoscale.
- Nanoparticle characterization: SEM provides information on size, shape, and surface properties of nanoparticles, which is essential for applications in drug delivery and nanomedicine.
- Nanostructure fabrication: The ability to observe defects and make corrections at the nanoscale supports the design of innovative nanomaterials.
In terms of TEM, it excels in:
- Structural analysis of nanomaterials: Understanding the arrangement of atoms in nanomaterials is crucial for their performance and stability.
- Research on quantum dots and nanowires: TEM is used to examine these materials' properties, aiming to develop advanced electronic components.
The integration of SEM and TEM in nanotechnology research fosters the development of new technologies with promising applications.
Future Trends in Electron Microscopy
The domain of electron microscopy is rapidly evolving. Future trends promise to enhance its capabilities, addressing many limitations experienced in current practices. This section explores notable advancements and the integration of techniques that are shaping the landscape of microscopy.
Advancements in Instrumentation
Recent developments in instrumentation have dramatically changed the field of electron microscopy. The push for higher resolution and improved imaging speed is evident. Modern scanning electron microscopes now feature advanced detectors and improved electron optics. These improvements lead to clearer images with higher contrast.
Furthermore, innovations in sample holders enable in situ experiments. Researchers can observe dynamic processes in real-time. Examples include studying chemical reactions or observing material behaviors under varying conditions. The introduction of field-emission guns provides a smaller beam size, allowing for finer detail capture.
In addition, automation and AI are transforming data acquisition and analysis. Instruments now can process vast datasets faster, improving throughput significantly. Techniques like machine learning are aiding in the interpretation of complex images. This ensures that the valuable data obtained can be utilized efficiently and effectively.
Integration with Other Techniques
The future of electron microscopy is not just about advancements in its standalone capabilities. There is a significant trend toward integrating it with other imaging and analytical techniques. Combining techniques allows for a more comprehensive understanding of samples.
For example, integrating atomic force microscopy (AFM) with SEM or TEM provides valuable insights. This combination allows researchers to gather topographical and electrical data alongside electron imaging. Similarly, coupling electron microscopy with spectroscopic techniques can reveal compositional information at the nanoscale.
Moreover, this integration often leads to multimodal imaging systems. Such systems provide a complete characterization of materials, addressing issues of resolution and information loss associated with single-technique approaches. This trend ultimately enhances both research capabilities and outcomes across various fields, ranging from nanotechnology to biological sciences.
"The integration of electron microscopy with other techniques expands our capabilities, providing richer datasets for analysis and understanding."
Finale
In this article, we explored the intricate differences between scanning electron microscopy (SEM) and transmission electron microscopy (TEM). Understanding these differences is crucial not only for researchers and practitioners in the field but also for anyone looking to employ these imaging techniques effectively. The conclusion serves as the synthesis of the insights gathered throughout our analysis, emphasizing the strengths and weaknesses inherent to both SEM and TEM.
Summary of Key Differences
Both SEM and TEM possess distinct characteristics that define their applicability in scientific research. Here are some key differences:
- Image Formation: SEM produces three-dimensional images by scanning the surface of a sample, while TEM provides two-dimensional images that capture internal structures using transmitted electrons.
- Sample Preparation: The preparation requirements for SEM are generally less stringent than those for TEM. SEM can accommodate bulk samples, whereas TEM necessitates ultrathin specimen sections to allow electron transmission.
- Resolution: While SEM typically reaches resolutions of about 1 nanometer, TEM often achieves resolutions well below that threshold, making it a superior technique for observing fine structural details.
- Information Provided: SEM excels in surface morphology and topography, while TEM is preferred for its capacity to reveal crystallographic information and defects within materials.
This summary encapsulates the crucial aspects to consider when choosing between these microscopy types. Understanding these differences aids in making informed decisions that best serve specific research objectives.
The Role of Electron Microscopy in Future Research
The role of electron microscopy—both SEM and TEM—in future research cannot be overstated. As technology progresses, these methodologies are expected to evolve, integrating with new techniques and enhancing their capabilities.
- Advancements in Instrumentation: Innovations in electron sources and detectors will likely improve the resolution and imaging speed of both SEM and TEM, facilitating the analysis of more complex materials at increasingly smaller scales.
- Integration with Other Techniques: Future research may increasingly focus on combining electron microscopy with complementary techniques, such as atomic force microscopy (AFM) or X-ray diffraction. This integration promises more comprehensive characterizations, enriching the interpretive power of observations made through electron microscopy.
- Emerging Applications: Fields such as nanotechnology, materials science, and biological research will continue to expand their reliance on SEM and TEM techniques. As new materials and biological samples present unique challenges, the adaptation and refinement of electron microscopy will play a critical role.