Tantalum Oxide: Properties, Uses, and Future Outlook
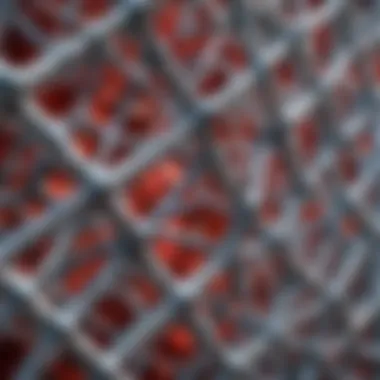
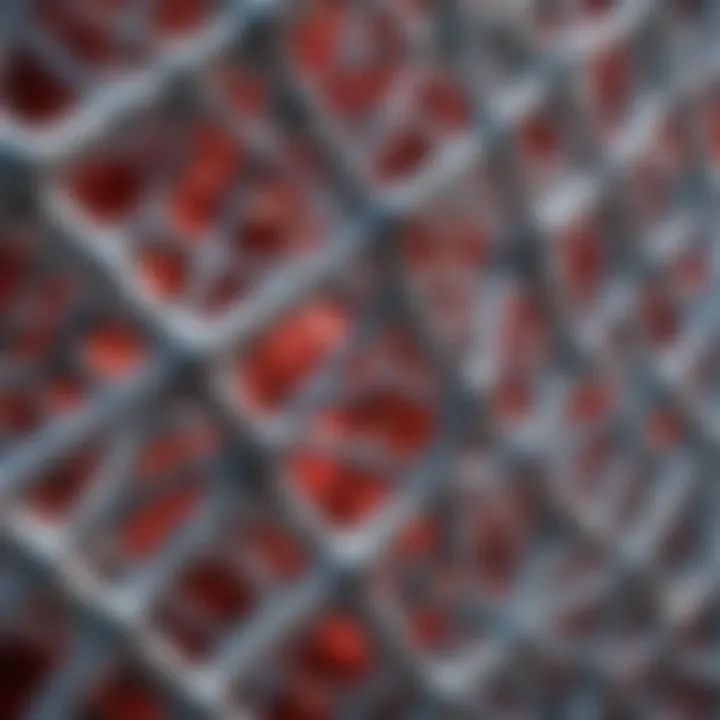
Intro
Tantalum oxide, noted for its chemical formula Ta2O5, stands out in the realm of advanced materials. It's like an underdog, quietly making waves across various scientific and industrial fields thanks to its remarkable properties. This article aims to delve into the intricacies of tantalum oxide, exploring its multifaceted applications that span from electronics to environmental disciplines. As a compound, tantalum oxide plays pivotal roles, enhancing technologies and addressing challenges, primarily due to its unique physical and chemical attributes.
TA2O5 isn't merely a compound for laboratory debate. It holds practical implications in electronics, optics, and even catalysis. Imagine a material that can function in harsh environments, exhibiting high dielectric constant, and serving as a barrier layer in capacitors. Its story of synthesis and application is thick with significance, promising a bright future as new methodologies surface.
The journey into tantalum oxide is replete with insights, shedding light on its synthesis methods and the diverse techniques employed to characterize it. One could say it’s a treasure trove for researchers and engineers alike. Given the evolving landscape of technology, understanding tantalum oxide’s role becomes even more critical.
Potential future prospects for tantalum oxide highlight its relevance in emerging applications, amplifying the excitement surrounding its study.
Let’s embark on this exploration.
Preface to Tantalum Oxide
Tantalum oxide, commonly represented as Ta2O5, has made quite the name for itself in the scientific community due to its impressive physical and chemical properties. From electronics to biomedical applications, this compound bridges multiple fields and showcases its versatility in technology. It stands out not merely for its functional characteristics, but for its significance in advancing various industrial processes. In this section, we’ll uncover its historical significance and delve into its intricate chemical composition and structure, setting the stage for a comprehensive understanding of tantalum oxide and its broad spectrum of applications.
Historical Context
The journey of tantalum oxide is as fascinating as the compound itself. Discovered in the early 19th century, tantalum was named after Tantalus, a figure from mythology who was eternally punished by unquenchable thirst and unattainable food. This nomenclature reflects the challenging extraction of tantalum from its ore, which took considerable effort due to its scarcity and the difficulty of purifying it. Over the decades, as technology evolved, so did the methods for synthesizing this valuable oxide.
In the 1940s, tantalum oxide began to catch the interest of researchers. It was primarily used in electronics, especially in capacitors, where its dielectric properties proved vital. Today, tantalum’s presence is ubiquitous in many high-tech applications, highlighting a remarkable shift from its humble beginnings in mineral discovery to its current status as a fundamental material in modern technologies. The evolution of tantalum oxide mirrors the strides in material science, resonating with how innovation fuels progress in various sectors.
Chemical Composition and Structure
Understanding the chemical composition and structure of tantalum oxide is crucial. The compound consists of tantalum and oxygen atoms arranged in a complex lattice. The empirical formula, Ta2O5, indicates that two tantalum atoms bond with five oxygen atoms. This unique arrangement gives tantalum oxide its distinctive characteristics, such as high stability and excellent electrical insulating properties.
Tantalum oxide crystallizes in a tetragonal structure, which contributes to its versatility in applications. This stability is often exploited in the fabrication of electronic devices, where a reliable insulator is a must.
"Tantalum oxide’s ability to maintain structural integrity under high temperatures makes it ideal for applications in harsh environments."
In summary, tantalum oxide's historical significance and its intricate chemical structure are foundational to its role in advancing technology. As we explore further, the multifaceted applications and the challenges faced by tantalum oxide in its synthesis and usage will become clear.
Synthesis of Tantalum Oxide
The synthesis of tantalum oxide (Ta2O5) is a fundamental topic that holds sway over its eventual applications and performance in various fields. Without the right synthesis methods, achieving desired properties and structures becomes a tall order. The importance of this section lies in understanding how the synthesis techniques shape the material's characteristics. More than just a science project, synthesizing tantalum oxide plays a critical role in the vast realms of electronics, optoelectronics, and even environmental solutions.
During synthesis, one must consider several elements: the purity of starting materials, the method used, and the environmental conditions maintained throughout the process. Each of these factors can significantly influence the end product’s quality and functionality.
Chemical Methods
When it comes to synthesizing tantalum oxide, chemical methods are often at the forefront. Among the most common techniques is the sol-gel process. This method allows for precise control over the particle size and distribution, which is crucial for various applications. In the sol-gel process, tantalum alkoxides—such as tantalum ethoxide—are typically used as precursors. Upon hydrolysis and subsequent gel formation, the tantalum oxide emerges as a fine powder upon drying and calcination.
Another noteworthy approach is the hydrothermal method. This method employs high temperature and pressure in an aqueous solution, yielding tantalum oxide with superior crystalline quality and homogeneity. The hydrothermal method has garnered attention due to its scalability and the ability to produce larger amounts of Ta2O5 efficiently. However, it requires careful management of temperature and reagent concentrations to avoid unwanted by-products.
- Pros of Chemical Methods:
- Cons to Keep in Mind:
- High purity of synthesized products
- Greater control over particle size and morphology
- Scalability in production
- Environmental concerns related to precursors
- The complexity of process optimization
Physical Methods
Physical methods bring a different flavor to the table when discussing tantalum oxide synthesis. One popular technique is sputtering, commonly utilized in producing thin films for electronic components. In sputtering, a target of tantalum is bombarded with argon ions, causing atoms to dislodge and deposit onto a substrate as tantalum oxide. The control over the deposition parameters—like pressure and temperature—can drastically influence the film's electrical properties and thickness.
Another intriguing physical method is plasma spraying, which provides an even more robust solution for applications requiring high durability. By injecting a tantalum feedstock into a plasma jet, the material rapidly vaporizes and solidifies upon contact with a substrate. This creates a dense, protective coating of tantalum oxide, reacting favorably in environments where thermal or chemical resistance is paramount.
- Advantages of Physical Methods:
- Challenges:
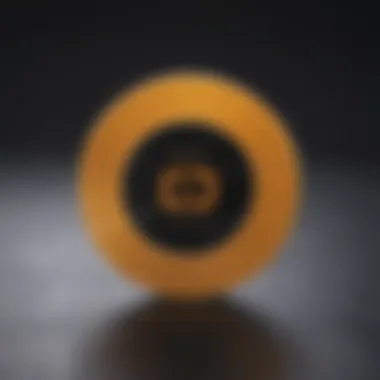
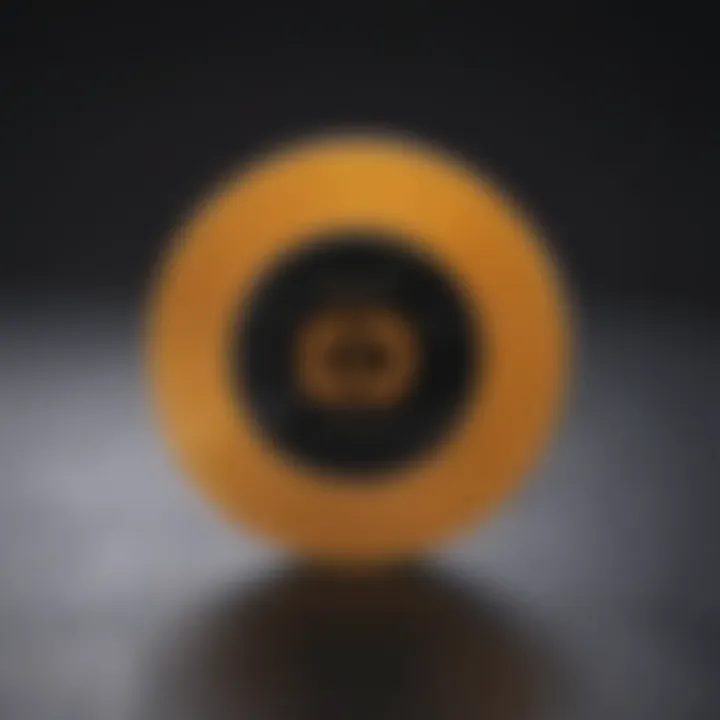
- Ability to create coatings with tailored thickness
- More straightforward material integration in devices
- Can achieve high deposition rates
- Potential high costs involved with equipment
- Process monitoring can be complex
The synthesis technique chosen significantly governs the performance characteristics of tantalum oxide. Thinking about your end application can help in deciding the best route for synthesis.
In summary, the synthesis of tantalum oxide involves a balance between chemical and physical methods, each with their benefits and challenges. Recognizing how each approach influences the material quality is vital for researchers and professionals looking to utilize tantalum oxide in advanced applications.
Characterization Techniques
When diving into the world of materials science, especially with compounds like tantalum oxide (Ta2O5), understanding characterization techniques is crucial. These methods bring out the intrinsic nature of the material, shedding light on its properties and enabling researchers to harness its potential in varied applications. Each technique offers unique benefits and insights, allowing for a thorough evaluation of tantalum oxide's capabilities.
Spectroscopic Methods
Spectroscopic techniques play a vital role in the characterization of tantalum oxide. They help determine the material's electronic structure, bonding characteristics, and phonon modes, among other attributes. One prominent example is Fourier Transform Infrared (FTIR) spectroscopy. This method examines how tantalum oxide absorbs infrared light, providing valuable information about its molecular vibrations and chemical bonds.
- Advantages of FTIR include:
- Non-destructive analysis, preserving the integrity of the sample.
- Quick data acquisition, facilitating timely investigations.
- Versatility across different materials, not just tantalum oxide.
Another key technique is Raman spectroscopy, which provides insights into the vibrational modes of the material. This is especially helpful in identifying phase transitions or structural changes in tantalum oxide as it undergoes certain conditions. The resonant Raman effect can enhance specific spectral features, making analyses even more precise.
In summary, employing spectroscopic methods is essential for unlocking the secrets of tantalum oxide, ensuring accurate interpretations for its applications in technology and beyond.
Microscopy Techniques
Microscopy techniques enhance our ability to visualize tantalum oxide down to the nanoscale. Techniques such as Scanning Electron Microscopy (SEM) and Transmission Electron Microscopy (TEM) are among the most utilized variants. SEM offers a surface-level view, revealing the morphology and topography of tantalum oxide samples across various scales.
- Key Benefits of SEM include:
- High-resolution imaging, allowing for detailed analysis of surface features.
- Capability to analyze elemental composition via Energy Dispersive X-ray Spectroscopy (EDS).
On the other hand, TEM provides insights into the internal structure of tantalum oxide. Through this method, researchers can gain high-resolution images that reveal crystallographic information, dislocation structures, and even defects within the material.
Both SEM and TEM are critical for researchers who seek to understand how the microstructural characteristics relate to the overall properties of tantalum oxide, guiding improvements in its synthesis and applications.
Electrical Property Measurements
The electrical properties of tantalum oxide are fundamental for its application in electronics and optoelectronics. Characterizing these properties helps establish how the material behaves under varying electrical conditions. Techniques such as impedance spectroscopy are often employed to explore the dielectric properties of tantalum oxide.
Impedance spectroscopy analyzes how an applied voltage influences the current in tantalum oxide, offering insights into its conductivity, permittivity, and loss mechanisms.
- Considerations when measuring electrical properties include:
- Sample preparation is crucial for accurate results.
- Environmental factors like temperature and humidity can affect measurements.
- Understanding the testing frequency range helps define performance characterization.
Additionally, capacitance-voltage profiling can be useful in determining the material's behavior in various electrical devices, like capacitors and transistors. Through these electrical property measurements, researchers gain a deeper insight into the feasibility and optimization of tantalum oxide in future technologies.
"Understanding the electrical properties of a material is as essential as recognizing its physical makeup; both dictate its applications and innovations in technology."
Properties of Tantalum Oxide
Tantalum oxide, known chemically as Ta2O5, is not just a mundane compound. Its properties set it apart in various fields, from electronics to environmental applications. Understanding these properties is pivotal, as they lay the groundwork for the exploration of tantalum oxide's capabilities and its future prospects. With a multitude of applications on the horizon, the characteristics of tantalum oxide compel researchers and industry professionals to examine this material in depth.
Physical Properties
Diving into the physical properties of tantalum oxide reveals a substance that's both versatile and robust. It primarily appears as a white powder, with a melting point around 1872 °C. Notably, its density is about 8.2 g/cm³, making it relatively heavy compared to many other oxides. But, it’s not just about its weight; the hardness of tantalum oxide is impressive as well—measured on the Mohs scale at around 6.5, it can withstand substantial wear and tear.
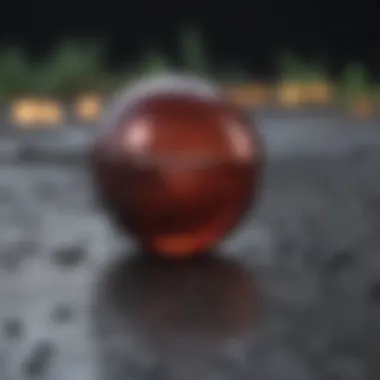
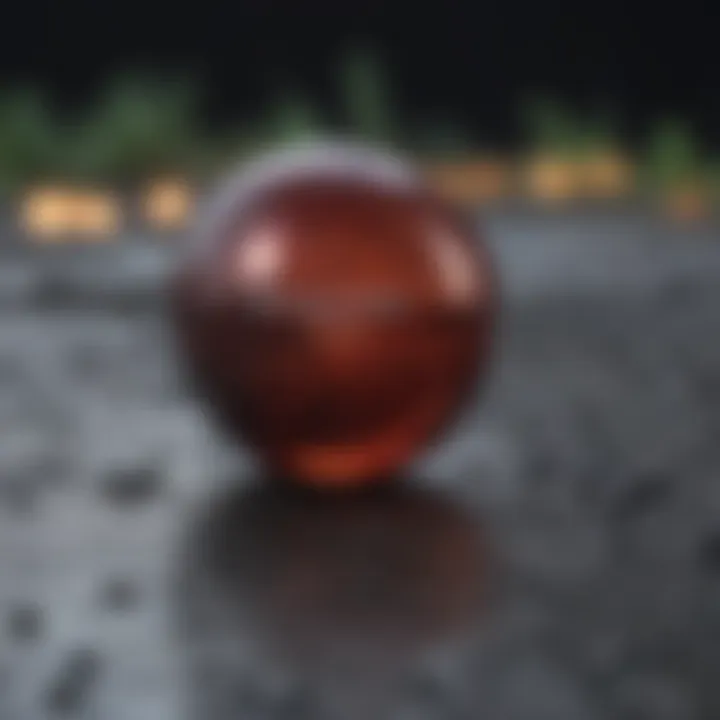
One fascinating aspect is the thermal stability it exhibits. Even at high temperatures, tantalum oxide maintains its integrity, which is a key consideration for applications in environments that are subject to thermal stress. Its good dielectric properties make it a prime candidate for use in capacitors, allowing it to store significant amounts of electric charge effectively.
What’s more? Tantalum oxide's molecular structure contributes to its performance. The crystalline form of Ta2O5 allows for varied applications in electronics, serving as a dielectric material in capacitors and integrated circuits. In sum, the physical properties of tantalum oxide present it as a formidable player in various high-tech applications.
Chemical Stability
When it comes to chemical stability, tantalum oxide proves its mettle. This compound is remarkably inert, resisting corrosion and reacting minimally with most acids, except for the highly aggressive hydrofluoric acid. Such stability is essential in applications where reactive environments may corrode or degrade lesser materials. Its robustness ensures extended lifespan and maintenance of performance in critical applications, especially in electronics and chemical processing.
The material also provides a considerable advantage in biomedical applications, where it does not interact negatively with bodily fluids. This property allows tantalum oxide to be used in devices like implants without significant worry about immune reactions.
Moreover, tantalum oxide forms a protective layer when exposed to certain chemicals, sealing its surface and preventing further degradation. This ability to form a stable, protective film adds another layer of utility, reiterating its position as a reliable compound in a wide array of environments.
Optical Properties
Turning to the optical properties of tantalum oxide, we encounter characteristics that are equally compelling. Notably, it exhibits high transparency in the infrared region while also showing notable refractive index, which ranges around 1.9 to 2.2. This makes it an appealing choice for various optoelectronic devices, such as lenses and coatings. The ability to manipulate light efficiently opens doors for innovation in fields such as telecommunications and imaging.
Another critical aspect of its optical performance is its high photocatalytic activity which has prompted research into its use in environmental applications, particularly in self-cleaning surfaces and pollution remediation techniques. The propensity for tantalum oxide to absorb and utilize sunlight is being harnessed in novel solutions aimed at tackling today’s environmental challenges.
In essence, the optical properties of tantalum oxide enhance its relevance across diverse applications, paving the way for further advancements in technology and sustainability.
Tantalum oxide stands as a testament to the potential of materials science. Its unique properties not only fulfill today’s technological needs but also hint at innovative future uses.
Applications of Tantalum Oxide
Tantalum oxide, or Ta2O5, is not just a lab curiosity; it has found routes into various essential applications across numerous industries. With its unique properties, tantalum oxide stands as a vital compound in the ever-evolving technological and industrial landscape. In this section, we’ll dissect the multi-faceted uses of tantalum oxide, spotlighting its significance and weighing the advantages and challenges that come with it.
Electronics and Semiconductors
In the realm of electronics, tantalum oxide shines particularly bright. Its high dielectric constant and excellent insulating properties make it a favored choice in the manufacturing of capacitors and thin film transistors. These capacitors are pivotal in microelectronic circuits, allowing for storing electrical energy in a compact size, which is crucial for mobile devices. The ability of tantalum oxide to form a stable oxide layer is what makes it indispensable for these applications. Not to mention, it's resistant to high voltages, making it fit for a variety of environments.
Moreover, recent advancements indicate a growing trend to explore even more intricate uses in semiconductor technology. As devices become smaller and more powerful, there’s a continual push for materials that can keep up without compromising performance.
Optoelectronic Devices
Tantalum oxide isn’t just confined to purely electronic applications; its role extends into the world of optoelectronics. This sector combines optics and electronics, involving devices that either emit, modulate, transmit, or detect light. Tantalum oxide serves here primarily as an optical coating material. It can be used in the production of anti-reflective coatings and mirrors for lasers, improving performance metrics in telecommunications and imaging systems.
The transparency of tantalum oxide in a wide range of wavelengths further bolsters its utility in photonic devices. Advances in research suggest that its optical properties can be fine-tuned, offering exciting possibilities for future applications in high-tech imaging and sensing technologies.
Biomedical Applications
In the biomedical field, tantalum oxide's strong biocompatibility sets it apart. It is often utilized in coatings for implants and medical devices. The remarkable quality of forming a strong bond with biological tissues allows for better integration when used in implants like dental fixtures or orthopedic screws. The properties of tantalum oxide not only enhance the longevity of these devices but also minimize the risk of rejection by the body.
In addition, studies have recently highlighted tantalum oxide for its potential role in drug delivery systems. Its nanoscale forms could act as carriers in targeted drug delivery, where precision in medication release can lead to more effective treatments with fewer side effects.
Environmental Applications
Tantalum oxide’s applications are not limited to technology and health; it also sees promising future prospects in environmental science. As the world grapples with the pressing need for sustainable resources, tantalum oxide emerges as a candidate in water treatment processes. Its ability to adsorb pollutants makes it a viable option for purifying water, especially heavy metals.
Additionally, its role as a catalyst in various chemical reactions adds another dimension to its utility. As researchers seek cleaner processes for energy production, tantalum oxide can potentially streamline methanol conversion into fuel, supporting sustainability goals.
"Tantalum oxide is not merely a compound; it’s a bridge to a cleaner, more advanced future across multiple sectors."
As we tread further into the exploration of tantalum oxide, it’s clear that its applications form a tapestry that interconnects technology, health, and environmental sustainability. With potential applications expanding, the importance of furthering research in this area cannot be understated.
Challenges in Tantalum Oxide Research
Tantalum oxide, while known for its remarkable properties and diverse applications, is not without its hurdles in research. Addressing these challenges is crucial for optimizing its use in various industries, which depend heavily on fine-tuning specific aspects of tantalum oxide’s production and application. Researchers, engineers, and industry professionals must navigate several obstacles to harness the full capabilities of tantalum oxide. The following subsections will elaborate on significant challenges faced in the realm of tantalum oxide research, namely synthesis limitations, characterization difficulties, and scalability issues.
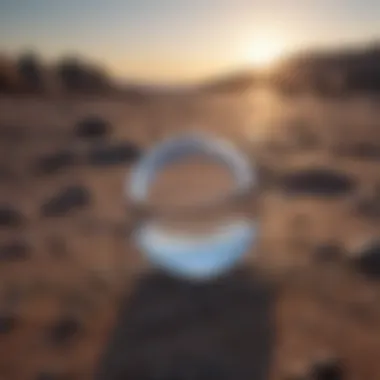
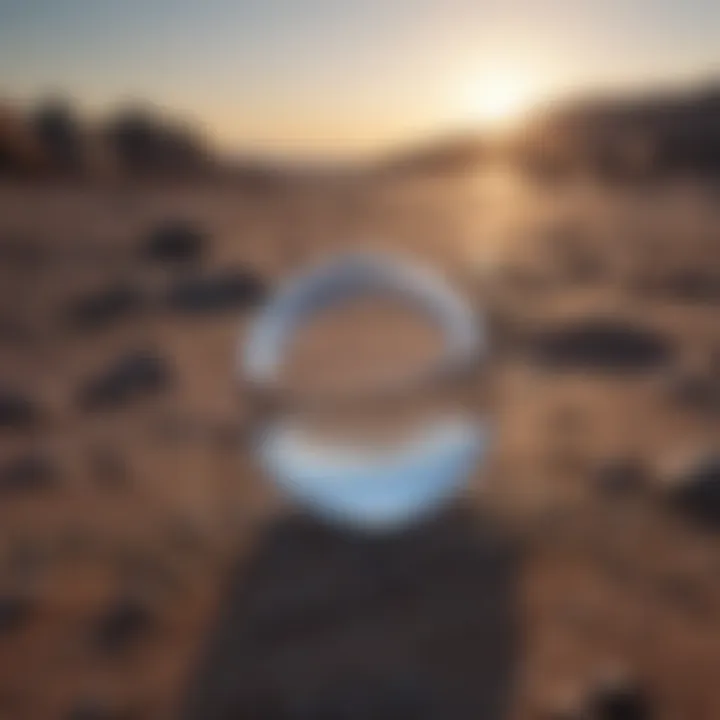
Synthesis Limitations
The synthesis of tantalum oxide is laden with complexities that often impede the production of high-purity and consistent material. One of the most prominent issues is the array of methods available that yield diverse results based on the starting materials and conditions. For instance, techniques like sol-gel and hydrothermal synthesis can vary greatly in terms of temperature, time, and environmental atmosphere. These variations can drastically influence the resulting structure and properties.
Moreover, purifying sources of tantalum is often a double-edged sword. The raw materials can contain impurities that not only affect performance but can also result in by-products that complicate the material's application. These impurities often present themselves as a persistent thorn in the side, as researchers strive for the pristine characteristics required for advanced applications, especially in electronics and optoelectronic devices. Furthermore, much time and effort must be spent optimizing the synthesis route, which can be a resource drain for researchers.
Characterization Difficulties
Characterizing tantalum oxide is akin to painting a picture without a clear palette; the challenge lies in discerning the properties and variations of the material at a molecular level. The methods employed for characterization—like X-ray diffraction, infrared spectroscopy, and electron microscopy—each have their unique limitations. For instance, while X-ray diffraction is excellent for providing information about crystal structure, it may not yield adequate data on non-crystalline or amorphous forms of tantalum oxide.
Additionally, optical characterization methods often demand specific conditions to obtain accurate results. Factors such as surface defects and variances in composition can skew findings, leading to an incomplete understanding of the material's behavior under different conditions. As most applications demand precise engineering, overlooking these minute details can result in products that do not perform as expected.
"A lack of comprehensive understanding can lead to unforeseen complications, stalling advancements in technology that rely on tantalum oxide."
Scalability Issues
Another significant barrier in tantulum oxide research is scalability. While a method may prove successful in a laboratory setting, transitioning to larger scales poses additional challenges. Factors such as cost, time, and resource availability weigh heavily on the viability of large-scale production.
For example, while chemical vapor deposition can yield high-quality tantalum oxide films on a small scale, scaling this process to industrial levels often results in diminished quality and increased risk of contamination. Additionally, the economic implications of sourcing high-purity tantalum compounds can become overwhelming, pushing project budgets to the limits.
In summary, the quest to further explore tantalum oxide is fraught with significant challenges that researchers must carefully navigate. By tackling synthesis limitations, characterizing the material effectively, and addressing scalability issues, the field can progress considerably, unlocking the true potential of tantalum oxide in various high-tech applications.
Future Prospects of Tantalum Oxide
The future for tantalum oxide is both intriguing and critical, holding promise across a multitude of sectors. Understanding its potential isn't merely an academic exercise; it can influence commercial applications and spur innovations in technology. This section focuses on specific elements such as innovative applications and prevailing research trends that outline tantalum oxide's trajectory.
Innovative Applications
Tantalum oxide isn't just a lab curiosity; it's positioning itself firmly in the practical world. Its high dielectric constant and excellent insulating properties make it a prime candidate for next-generation electronics. For instance, as manufacturers delve deeper into miniaturization, tantalum oxide could become essential in the production of smaller capacitors.
Furthermore, tantalum oxide's role in optoelectronic devices—those that combine light and electronics—is seeing significant advancements. Researchers are examining its usability in light-emitting diodes (LEDs) and laser technologies. By leveraging its unique optical properties, it may be possible to create devices that are not only more efficient but also capable of operating at wavelengths that traditional materials cannot handle.
In the biomedical arena, tantalum oxide is making inroads, especially in implants and drug delivery systems. Due to its biocompatibility and ability to form a protective layer, it’s an ideal material for devices that need to coexist with biological tissue without adverse effects. As the healthcare industry leans toward more advanced and integrated solutions, tantalum oxide's applications could be pivotal in enhancing patient outcomes.
"The continual refinement of tantalum oxide application could lead breakthroughs that change medical devices fundamentally."
Moreover, environmental applications are unfurling as tantalum oxide’s properties lend themselves to pollution control mechanisms and energy storage solutions. For example, in photocatalytic applications, engineers are exploring how tantalum oxide can help in breaking down environmental pollutants when exposed to light, potentially leading to cleaner technologies.
Research Trends
As we navigate the landscape of tantalum oxide, research trends reveal significant areas of focus. One notable trend is the synthesis of nanostructured tantalum oxide materials. Researchers are investigating how altering the morphology of tantalum oxide at the nanoscale can affect its properties. With smaller particle sizes, the surface area increases, which may enhance its reactivity and performance in various applications.
Another area attracting attention is the incorporation of tantalum oxide into hybrid materials. These composites could harness the strengths of various materials to push the limits of current technology. For example, blending tantalum oxide with other metal oxides could yield products that exhibit even better electrical or thermal properties, offering pathways to innovate devices, particularly in the semiconductor field.
Additionally, computational modeling is increasingly playing a role in this field. By employing advanced simulation techniques, scientists can predict how tantalum oxide will behave in different environments and applications. It streamlines the research process, potentially shortening development cycles and enabling quicker, data-driven decisions about application methods.
The integration of these research trends, combined with the exploration of tantalum oxide's vast potential, paints a promising picture for the future. As its applications expand into electronics, biomedicine, and environmental technology, tantalum oxide's role in modern advancements is set to grow, offering benefits that could ripple throughout industries. With ongoing exploration in these areas, the future of tantalum oxide is not only critical but also transformational.
End
Summary of Key Points
Throughout this exploration, several pivotal aspects have come to light:
- Properties: The compelling physical and chemical properties of tantalum oxide, including its commendable resistance to corrosion and high thermal stability, make it suitable for use across various domains.
- Versatile Applications: Tantalum oxide finds its application not just in electronics, where it enhances semiconductor performance, but also in other fields like optoelectronics, biomedicine, and environmental conservation.
- Challenges: Despite its vast potential, challenges persist in synthesis, characterization, and scalability in manufacturing this vital compound. Addressing these issues will pave the way for broader adoption and innovation.
- Future Prospects: Emerging technologies and research trends are hinting at innovative applications for tantalum oxide. This includes its role in energy storage solutions and advanced biomedical devices, which could significantly alter current technological frameworks.
Implications for Future Research
The implications for future research surrounding tantalum oxide reveal a promising avenue for scientists and engineers alike. There’s a pressing need to delve into:
- Improved Synthesis Techniques: Enhancing existing methods or developing new approaches could optimize the properties of tantalum oxide, making it more accessible for various applications.
- Emerging Applications: Exploring uncharted territories like its integration in quantum computing or advanced renewable energy systems might unlock further potential.
- Environmental Impact Studies: Given the increasing emphasis on sustainability, research into the environmental impacts of tantalum oxide production and application should be prioritized. Understanding these factors will ensure responsible innovation moving forward.
In summary, tantalum oxide stands as a beacon of promise, intertwining its past with future advancements. As we push the boundaries of science, keeping a close eye on this compound could unveil many more facets yet to be discovered. The journey of unraveling its potential is just beginning. Whether in enhancing technology or contributing to environmental efforts, tantalum oxide is set to play a pivotal role in shaping various fields for years to come.