Total RNA Isolation Protocol: A Comprehensive Guide
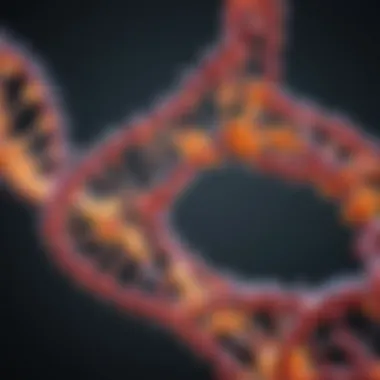
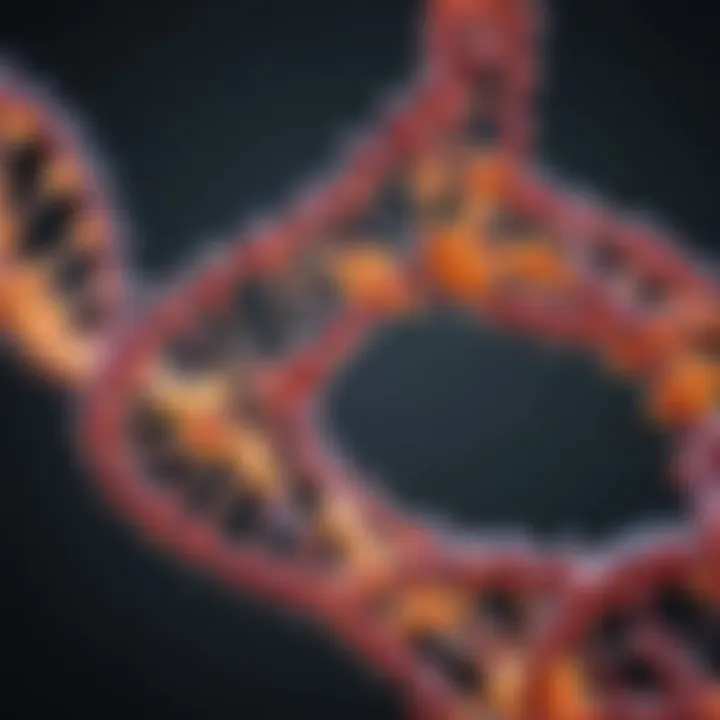
Intro
Total RNA isolation is a crucial process in molecular biology that enables researchers to study gene expression and the functional dynamics of RNA. RNA plays a central role in various cellular processes, and understanding its behavior can provide insights into gene regulation, cellular response, and even disease mechanisms. This guide aims to present a detailed methodology for isolating total RNA from diverse biological samples – offering both clarity and rigor.
This article will delve into key aspects of RNA isolation, including an overview of significant findings in the field, methodological approaches, essential reagents, and troubleshooting strategies. The goal is to furnish researchers with the tools necessary for successful RNA extraction, thus enhancing their research capabilities.
Research Overview
Summary of Key Findings
Isolating RNA effectively from various sources is paramount for accurate research outcomes. Over the years, methods and protocols have evolved, enabling improved yield and quality of total RNA. Studies have shown that the choice of isolation method can dramatically impact the integrity and purity of the RNA harvested. Techniques can range from organic extraction methods, like those using phenol and chloroform, to column-based purification methods.
"Underestimating the complexity of RNA isolation can lead to misinterpretation of downstream results."
Background and Context
The evolution of RNA isolation techniques can be traced back to the early days of molecular biology. As scientific inquiry has advanced, so too has the need for refined protocols that ensure reproducibility. Each biological sample presents unique challenges, whether it is tissue, cell cultures, or tricky substances like plant material. Understanding the context in which RNA is investigated lays the groundwork for selecting the appropriate extraction technique.
Different techniques may have various implications, such as yield differences or the presence of inhibitors that hinder downstream applications such as RT-PCR. By grasping the historical perspective and the technological advancements in RNA isolation, researchers can make informed decisions about their methodological strategies.
Methodology
Experimental Design
The design of an RNA isolation experiment should consider both the sample source and the downstream applications planned. Researchers must carefully select the extraction method based on factors such as:
- Type of sample: tissue, blood, or cultured cells
- Desired RNA quality and quantity
- Specific downstream applications (e.g., qPCR, sequencing)
Data Collection Techniques
Data collection in RNA experiments commonly necessitates stringent controls to quantify the quality of the isolated RNA. This may include:
- Spectrophotometry to assess RNA purity and concentration
- Agilent Bioanalyzer or similar devices for checking RNA integrity
- PCR or qPCR for functional verification of quality
Integrating these data collection techniques into the RNA extraction workflow is essential for ensuring reliable results.
Intro to Total RNA Isolation
Total RNA isolation is a methodically important procedure in molecular biology. By obtaining total RNA from various biological samples, researchers gain the ability to explore gene expression, unravel RNA structure, and evaluate functional profiles. Understanding how to properly isolate RNA is essential for advancing research in genetics, cell biology, and many other fields.
The significance of this article lies in its effort to demystify the process of total RNA isolation. RNA analysis serves a crucial role in comprehending cellular functions and diseases. Inaccurate isolation methods can lead to erroneous data, impacting the entire research project. This guide aims to present a clear overview of the protocols, offering clarity on the steps involved, along with the tools and reagents required.
Importance of RNA Analysis
RNA analysis is critical for various aspects of biological research. First, it opens pathways for understanding gene expression during different stages of development or in various environments. By examining RNA levels, scientists can infer which genes are active or silent, giving insights into cellular responses and processes.
Moreover, RNA serves as a marker for diseases. For example, altered expression patterns can signal the presence of cancer or other medical conditions, leading to potential diagnostic applications. With RNA's involvement in protein synthesis, studying its behavior can shed light on diseases caused by genetic mutations. Thus, understanding and analyzing RNA is not just significant for basic science; it extends to applied medical research as well.
Furthermore, by employing total RNA isolation, researchers can analyze diverse forms of RNA, leading to broader insights. With such analysis, RNA sequencing, microarray analysis, and even quantitative PCR can be facilitated.
Applications of Total RNA Isolation
Total RNA isolation finds its applications in multiple disciplines, ranging from basic research to advanced clinical studies. Here are some important areas where RNA isolation is utilized:
- Gene Expression Profiling: Researchers often analyze RNA to assess the levels of specific mRNAs. This can reveal how cells respond to treatments or environmental changes.
- Functional Genomics: Total RNA is essential for understanding gene function and the relationships between genes in complex networks.
- Disease Research: Isolated RNA enables the exploration of disease mechanisms and identification of biomarkers, potentially leading to more precise therapies.
- Drug Development: Analyzing how drugs affect gene expression through RNA analysis provides foundational knowledge that aids in developing new therapeutics.
- System Biology: Total RNA contributes significantly to understanding cellular systems, helping to create a holistic view of biological processes.
In summary, RNA isolation is pivotal not just to acquire data, but also to translate that data into meaningful biological insight.
Understanding RNA Structure and Types
Understanding the structure and types of RNA is crucial for anyone working in molecular biology and related fields. RNA plays a central role in various biological processes, especially in gene expression and protein synthesis. Grasping these concepts aids in the effective utilization of total RNA isolation protocols.
Different types of RNA serve specific functions in cellular activities. These include messenger RNA (mRNA), ribosomal RNA (rRNA), and transfer RNA (tRNA). Each type has unique characteristics that are essential for their role in the processes of transcription and translation. Additionally, knowledge of these RNA types helps in optimizing extraction protocols and analyzing RNA effectively.
Types of RNA
mRNA
Messenger RNA is a single-stranded molecule that carries genetic information from DNA to the ribosome, where proteins are synthesized. This unique role of mRNA makes it vital in the overall gene expression mechanism. A key characteristic of mRNA is its capability to be translated into proteins. This attribute makes it a popular choice for various molecular analyses, especially in studies seeking to understand gene expression levels.
The advantage of mRNA is its abundance and stability under appropriate conditions, making it relatively easier to isolate. However, it is also susceptible to degradation by nucleases, necessitating strict adherence to proper handling protocols during isolation to ensure integrity and reliability in downstream applications.
rRNA
Ribosomal RNA constitutes a key component of ribosomes, the cellular machinery responsible for protein synthesis. rRNA is prominent in the nucleolus and forms the structural framework for ribosomes. Its primary characteristic is its involvement in the catalytic function of ribosomes in the translation process.
For this guide, rRNA is a beneficial choice because it contributes to the overall measurement of total RNA, helping evaluate ribosomal integrity. However, one limitation is that rRNA makes up a large portion of total RNA, which can sometimes dilute the signals of other RNA types when analyzed, such as mRNA.
tRNA
Transfer RNA is vital for translating the mRNA sequence into a specific amino acid sequence during protein synthesis. The key characteristic of tRNA is its unique ability to carry and match the appropriate amino acids to the corresponding codons on the mRNA strand.
tRNA is a significant choice for studies involved with translation processes, facilitating the understanding of how proteins are synthesized based on mRNA templates. Its rapid turnover rates in cells can be both an advantage and a disadvantage, as it may not always be present in sufficient quantities for isolation purposes, potentially affecting analytical outcomes.
RNA vs. DNA
One of the fundamental distinctions in molecular biology is between RNA and DNA. Both nucleic acids are essential for life, but they differ in structure and function. RNA is usually single-stranded, whereas DNA is double-stranded. The sugar in RNA is ribose compared to deoxyribose in DNA, and RNA utilizes uracil instead of thymine.
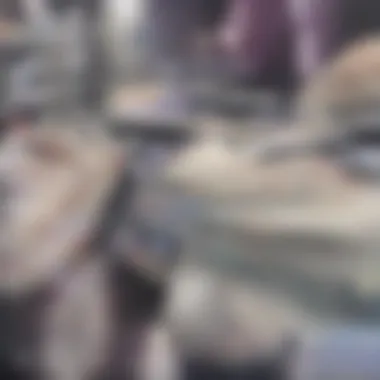
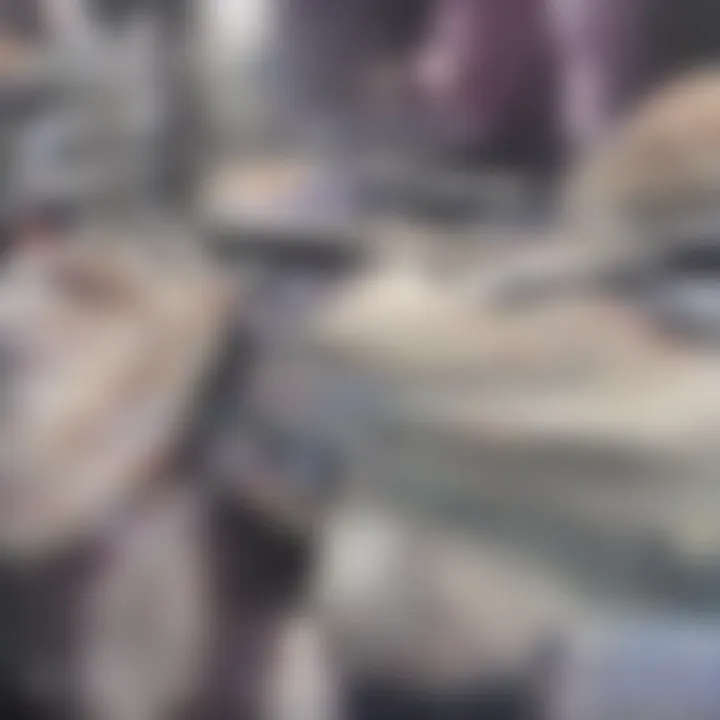
These differences impact stability and functionality. RNA is generally more prone to hydrolysis, making it less stable than DNA. Consequently, different protocols and strategies are necessary for the isolation and analysis of RNA compared to DNA, which is more robust and easier to extract. Understanding these differences is essential when developing effective RNA isolation techniques and interpreting results accurately.
"The isolation and analysis of RNA are integral to unraveling the complexities of gene expression and cellular function."
In summary, recognizing the various types of RNA and the differences between RNA and DNA is essential for effectively engaging with total RNA isolation protocols. This knowledge not only enhances understanding in practical applications but also informs better experimental design and data interpretation.
Overview of RNA Isolation Techniques
The isolation of total RNA is a crucial component of molecular biology research. Understanding the various techniques available for RNA isolation is essential for selecting the most effective method based on the sample type and downstream applications. Each technique has its distinct advantages and considerations, making it vital for researchers to grasp these differences. Techniques such as phenol-chloroform extraction, column-based methods, and magnetic bead-based extraction are among the most prevalent. Each method retains unique attributes, influencing factors like RNA yield, purity, and efficiency. Through this section, we will explore these techniques in detail, enabling informed choices for successful RNA isolation.
Phenol-Chloroform Extraction
Phenol-chloroform extraction is a time-honored technique for isolating total RNA. This method employs the use of phenol and chloroform to facilitate phase separation of RNA from proteins and other cellular debris. The principle is based on the differential solubility of biological molecules in organic solvents. When mixed, this solution undergoes centrifugation, resulting in distinct phases: an upper aqueous phase containing RNA and a lower phase containing proteins and lipids.
Advantages of phenol-chloroform extraction include:
- High purity of isolated RNA.
- Effective removal of contaminants such as proteins and DNA.
However, there are notable considerations.
- This method can be hazardous and requires careful handling of toxic chemicals.
- The process can be time-consuming and labor-intensive.
Despite these challenges, phenol-chloroform extraction remains a powerful tool for RNA isolation, especially when the focus is on obtaining high-quality RNA for downstream applications.
Column-Based Methods
Column-based methods are popular for their simplicity and efficiency. These techniques generally involve using silica membranes or polymer-based columns to bind RNA. After the sample is applied, washing steps remove contaminants, leaving purified RNA bound to the column. Subsequently, RNA is eluted using an appropriate buffer.
Benefits of column-based methods include:
- Quick and straightforward protocols suitable for high-throughput applications.
- Reliable yield and quality of RNA.
Nonetheless, there are restrictions to acknowledge:
- The cost associated with purchasing commercial RNA extraction kits.
- Possibility of incomplete elution, which can affect yield.
Column-based methods have established themselves as reliable RNA extraction protocols, particularly useful for routine applications where speed and convenience are paramount.
Magnetic Bead-Based Extraction
Magnetic bead-based extraction is increasingly utilized in RNA isolation due to its versatility and effectiveness. This technique employs magnetic beads, typically coated with oligo(dT) or other capture molecules, to bind RNA. The sample is mixed with the beads, and a magnet is used to separate the bound RNA from the solution. After a series of washes, RNA is eluted from the beads.
This method offers several advantages:
- Reduced risk of contamination, as the process is performed in a controlled setting.
- Flexibility in scale, enabling both small and large sample preparations.
On the downside:
- Requires access to specialized equipment like a magnetic separator.
- Potential for lower yield compared to traditional methods if not optimized.
Each technique carries its own balance of convenience, yield, and purity, making the right choice depending on the research goals and specific sample considerations.
Materials and Reagents Needed
The process of RNA isolation requires specific materials and reagents to ensure successful extraction and purification. Each item plays a vital role in maintaining the integrity and quality of RNA. Understanding the importance of each reagent and equipment is critical in the overall efficacy of the RNA isolation protocol. The selection of high-quality materials directly influences the yield and purity of the extracted RNA.
Reagents for RNA Extraction
RNA Extraction Kit
RNA extraction kits are designed to simplify the isolation process. These kits often include essential reagents such as lysis buffers, purification columns, and specific enzymes. A significant aspect of RNA extraction kits is their ability to enhance yield while minimizing contamination risk. Popular kits, like the Qiagen RNeasy Kit, are favored among researchers for their reproducibility and efficiency.
One key characteristic of these kits is the inclusion of a special binding buffer that favors RNA binding to a silica membrane. This unique feature allows for effective separation from DNA and proteins. However, while kits can streamline the process, they might also introduce variability if not used properly.
Detergents
Detergents are crucial in the cell lysis step. They help disrupt cellular membranes, facilitating the release of RNA. Commonly used detergents such as SDS (sodium dodecyl sulfate) are effective at breaking down lipid bilayers. The benefit of using detergents lies in their ability to provide consistent and thorough lysis, which is vital for maximizing RNA yield.
A notable limitation is that excessive amounts can lead to contamination or degradation of RNA, thus careful optimization of detergent concentration is needed. This balancing act is where experience and protocol familiarity come into play.
Buffers
Buffers play a significant role in maintaining the stability of RNA during isolation. They help maintain pH levels and provide an optimal environment for the enzymes involved in the extraction process. Tris-buffered saline is a common choice due to its neutrality and compatibility with RNA stability.
The specificity of buffers for certain applications can be a double-edged sword. While they optimize extraction, inappropriate buffer types can hinder downstream applications. This makes it essential to select buffers appropriate for the intended analysis or use of the isolated RNA.
Required Laboratory Equipment
Proper laboratory equipment is just as important as reagents in RNA isolation. This equipment ensures that the extraction process is efficient, safe, and reproducible.
Centrifuge
A centrifuge is instrumental in the separation of RNA from cellular debris. The force generated by centrifugation facilitates the partitioning of materials based on density, which is essential for RNA isolation. High-speed centrifuges are particularly useful for this purpose. A key characteristic is the rotor speed, which should be suited for the specific protocol requirements.
Using a reliable centrifuge minimizes variability in RNA recovery. However, using higher speeds than required might result in RNA fragmentation. Thus, following protocol specifications is crucial for ensuring RNA integrity.
Pipettes
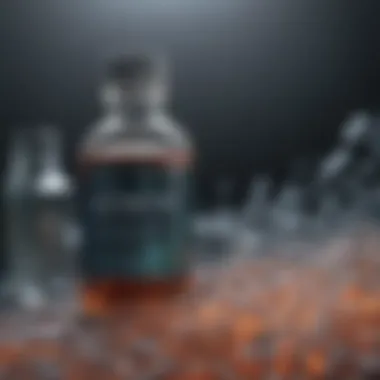
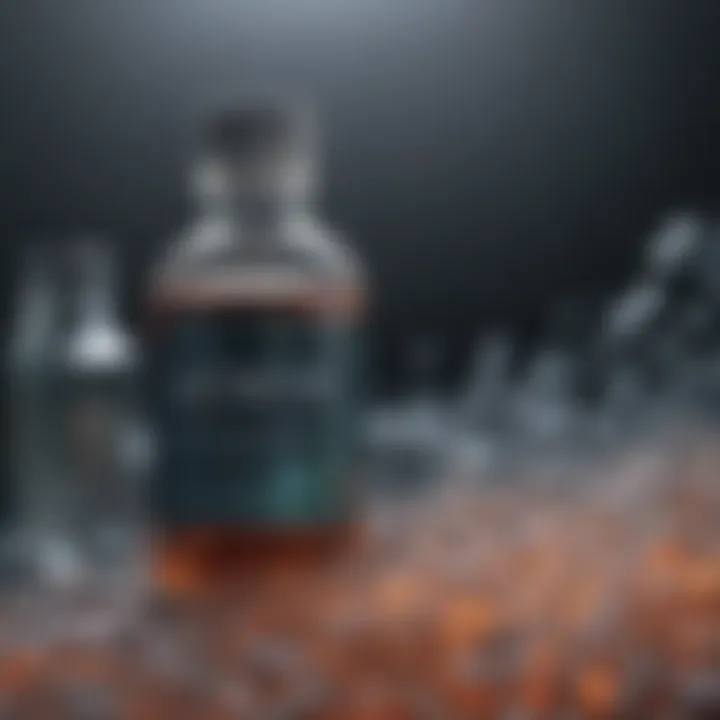
Accurate pipetting is essential in molecular biology labs, especially in RNA extraction. Pipettes allow the controlled transfer of reagents and samples, minimizing contamination risks. Choosing high-quality pipettes contributes to precision in every step.
The unique feature of adjustable pipettes is a notable advantage. They provide flexibility for using various volumes depending on the experimental needs. However, user technique can significantly affect results, emphasizing the importance of training in pipetting skills.
UV Spectrophotometer
A UV spectrophotometer is valuable for quantifying and assessing the purity of isolated RNA. It allows for the measurement of absorbance at specific wavelengths, which correlates with RNA concentration. The reliability of results from a UV spectrophotometer makes it a beneficial tool in the RNA isolation process.
This device often has the ability to measure purity ratios (like A260/A280), serving as a quality control step. However, care must be taken to ensure the cuvettes used are of high quality to avoid discrepancies.
RNA isolation is a delicate process, requiring attention to detail and appropriate materials for success.
Step-by-Step RNA Isolation Protocol
The process of isolating RNA from biological samples is integral to many molecular biology applications. Each step in the RNA isolation protocol is crucial to its success, ensuring the integrity and purity of the extracted RNA. This section provides a detailed look into the individual phases of RNA isolation, elucidating specific elements, benefits, and considerations that researchers must take into account.
Sample Preparation
The initial stage of RNA isolation involves sample preparation. Proper preparation ensures that cells or tissues are suitable for extraction, maximizing yield and quality. Samples can come from various sources like cultured cells, tissues, or blood.
Considerations should include:
- Sample size: Ensure the amount is sufficient to yield measurable RNA.
- Storage conditions: Samples should be fresh or stored at appropriate temperatures (typically -80°C) to prevent degradation.
- Handling: Use RNase-free tubes and reagents to minimize contamination.
By adhering to these guidelines, researchers can enhance the likelihood of successful RNA extraction later in the process.
Cell Lysis
Cell lysis is essential to release RNA into the solution. This process disrupts the cell membranes, allowing for the release of intracellular components, including RNA. Various lysis methods exist, including physical and chemical approaches.
Common considerations include:
- Choice of lysis buffer: The buffer should be designed to inhibit RNase activity, preserving RNA integrity. Common choices include TRIzol and RLT buffer.
- Duration: Enough time must be allowed for complete lysis. Insufficient lysis can result in low RNA yields.
- Method: Be mindful of the method of lysis; gentle methods such as freeze-thaw cycles may be appropriate for sensitive samples.
Effective cell lysis sets the stage for successful extraction in subsequent steps.
Phase Separation
Phase separation involves separating the RNA from proteins and other cellular debris. This step varies depending on the isolation method chosen. Typically, phenol-chloroform extraction is useful, but column-based and magnetic bead methods have become popular for their efficiency.
Key steps include:
- Incubation: Allow the mixture to sit to facilitate phase separation. Often, this is done by adding a chloroform and shaking gently.
- Centrifugation: This step helps separate the aqueous phase (containing RNA) from the organic phase (containing proteins). Careful pipetting is essential here to avoid contamination.
Understanding how to properly perform phase separation is critical for high-quality RNA extraction.
Precipitation of RNA
Once RNA is in the aqueous phase, precipitation is needed to isolate it further. This typically involves the addition of alcohols such as ethanol or isopropanol. These solvents help aggregate the RNA, making it more visible and easier to handle.
Specific points to consider include:
- Temperature: Usually, cold ethanol or isopropanol is used to enhance RNA precipitation efficiency.
- Incubation time: A longer incubation improves yields, typically overnight at -20°C.
- Centrifugation: After incubation, centrifuging helps collect the RNA pellet. Following this, the supernatant must be discarded carefully to avoid losing RNA.
This step is vital in ensuring that the RNA is correctly separated from other cellular components.
RNA Purification
The purity of RNA after isolation is critical for downstream applications. The purification process often involves washing steps to remove residual contaminants from the RNA pellet. This is where the choice of purification method comes into play. Common methods include:
- Column-based purification: Utilizing silica membranes to bind RNA selectively while washing away contaminants.
- Magnetic bead-based methods: Beads that specifically bind RNA can also be used, allowing for easy separation via a magnet.
Considerations for purification include:
- Washing buffers: Ensure that buffers are prepared correctly to adequately separate RNA from contaminants.
- Final resuspension: Choose the appropriate buffer or water to resuspend RNA, as this can affect downstream applications such as qPCR.
Ensuring thorough purification is essential for obtaining high-quality RNA suitable for various analyses.
Proper methodology throughout the RNA isolation process enhances the probability of obtaining reliable and reproducible data from subsequent experiments.
By focusing on each step in the RNA isolation protocol, researchers can enhance their understanding and application of this crucial technique, optimizing RNA yields and purity for further experimentation.
Evaluation of RNA Quality and Quantity
Evaluating the quality and quantity of isolated RNA is crucial in molecular biology. This step ensures that the RNA is suitable for downstream applications, such as quantitative PCR, microarray analysis, and RNA sequencing. Poor-quality RNA can lead to unreliable results, rendering experiments invalid and wasting precious time and resources.
The assessment of RNA quality typically involves checking for purity and integrity. High-quality RNA is free from contaminants like proteins and other nucleic acids. Additionally, the integrity of RNA can indicate whether the RNA extraction process was successful, which is vital for obtaining reproducible data.
By employing accurate evaluation techniques, researchers can confidently move forward with their analysis, knowing that their samples meet the necessary criteria for further experimentation. This evaluation not only aids in troubleshooting during RNA isolation but also prepares scientists for upcoming challenges in their research.
"Quality is more important than quantity."
Spectrophotometry
Spectrophotometry is a widely used method for measuring RNA concentration and purity. This technique utilizes UV absorbance at 260 nanometers (nm), where nucleic acids absorb light. The RNA concentration can be calculated using the absorbance readings, typically multiplied by a factor of 40 for RNA to convert the absorbance value into concentration (µg/mL).
In addition to concentration, the purity of RNA is assessed using the ratios of absorbance readings at 260 nm to 280 nm and 260 nm to 230 nm. A ratio of 1.8 is commonly accepted for pure RNA; deviations from this ratio can indicate contamination.
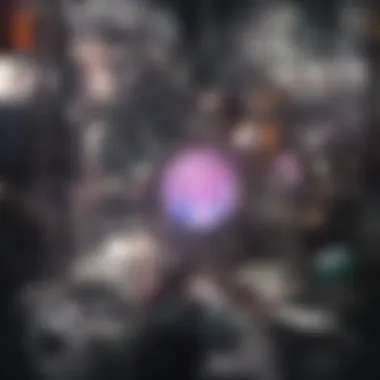
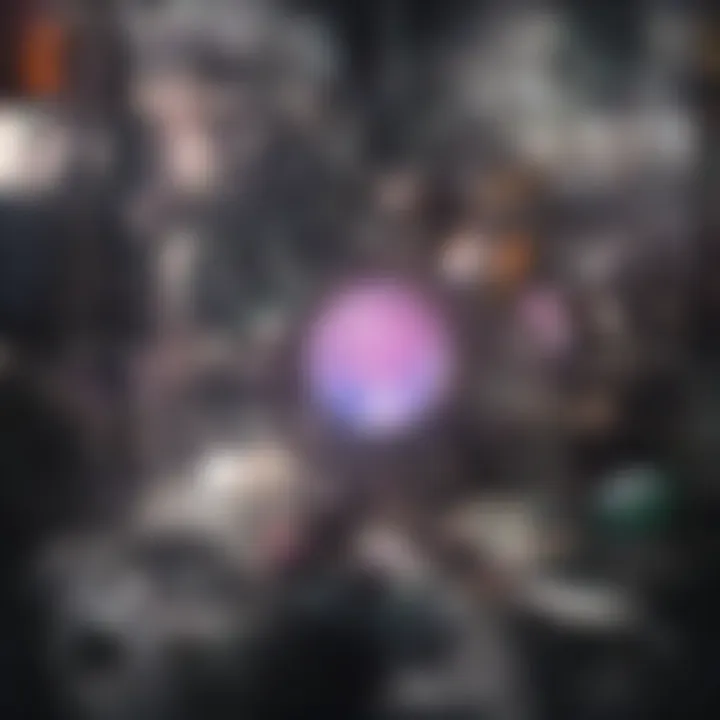
This method is fast and requires minimal sample volume, making it preferable for many laboratories. However, it is important to note that spectrophotometry does not provide information regarding the integrity of RNA.
Agarose Gel Electrophoresis
Agarose gel electrophoresis is another crucial approach to evaluate the integrity and size distribution of RNA samples. This technique allows researchers to visualize RNA bands and ascertain whether the RNA molecules are intact or degraded.
Typically, agarose gels are prepared and RNA samples are loaded. An electric current is then applied, causing the RNA to migrate through the gel matrix. Smaller RNA fragments travel faster than larger ones, leading to a separation based on size.
After electrophoresis, the gel is stained with ethidium bromide or a similar dye to visualize RNA under ultraviolet light. High-quality RNA appears as distinct bands, usually showing sharp rRNA bands for eukaryotic samples, while degraded RNA will appear as a smear. This visualization provides reliable information needed to confirm RNA quality before further applications.
Both methods of evaluation complement one another, providing comprehensive insight into RNA integrity and suitability for downstream applications.
Common Challenges in RNA Isolation
The process of isolating total RNA is crucial for various molecular biology experiments. However, several challenges can hinder the effectiveness of RNA extraction. Understanding these challenges is important for researchers. Recognizing potential pitfalls can significantly improve the RNA isolation process, facilitating better results in downstream applications. Common issues include contamination risks and RNA degradation, both of which can adversely affect sample quality and integrity.
Contamination Issues
Contamination is one of the primary concerns during RNA isolation. Contaminants such as DNA, proteins, or other RNAs can significantly interfere with results. These impurities can lead to inaccurate measurements of RNA quantity and quality, ultimately affecting analysis results. Additionally, RNA is especially sensitive to enzymes such as RNases, which degrade RNA. Therefore, it is essential to maintain a clean and sterile work environment.
Several strategies can mitigate contamination issues:
- Use RNase-free reagents and consumables. This includes using certified RNase-free water and tubes.
- Implement strict cleaning protocols. Proper cleaning of surfaces and equipment reduces the risk of contamination.
- Employ personal protective equipment (PPE). Gloves should be worn at all times to prevent degrading the sample with skin enzymes.
Following these best practices helps ensure that isolated RNA remains pure and suitable for subsequent experiments.
Degradation of RNA
RNA degradation is another significant hurdle during isolation. RNA is inherently unstable due to its structure, making it prone to degradation by endogenous RNases present in tissues and cells. Even minute exposure to RNases can result in substantial loss of RNA during the isolation process. Thus, timing and temperature play critical roles in minimizing degradation.
To prevent RNA degradation, researchers should consider the following:
- Use RNA stabilizing agents. These reagents help preserve RNA integrity during sample collection and handling.
- Perform the isolation as quickly as possible. Processing samples immediately after collection reduces the time RNA is exposed to degradative conditions.
- Consistently maintain samples at low temperatures. Keeping samples on ice during the extraction process can slow RNA degradation effectively.
By taking these precautions, the quality of isolated RNA can be preserved, ensuring reliable results in research.
Tips for Successful RNA Isolation
In the field of molecular biology, success in RNA isolation can greatly influence the quality of subsequent experiments. The process often presents various challenges; thus, applying effective tips can ensure reliability and reproducibility in results. With that said, understanding the nuances of RNA isolation will aid researchers in minimizing errors, optimizing yield, and enhancing the quality of RNA obtained from samples.
Best Practices
Implementing best practices during RNA isolation is critical. These practices can significantly impact the integrity and yield of isolated RNA. Here are some recommended approaches:
- Work Quickly and Maintain RNase-Free Conditions: RNA is susceptible to degradation by ribonucleases (RNases). It is advisable to work swiftly and use RNase-free reagents and consumables to minimize risk. Wearing gloves, using RNase-free tubes, and ensuring that all surfaces are decontaminated can help preserve RNA quality.
- Use Proper Sample Handling Techniques: Sample collection and storage can affect RNA quality. It is essential to immediately snap-freeze samples in liquid nitrogen or store them at very low temperatures until RNA extraction. Using appropriate stabilizing solutions can also enhance RNA preservation.
- Follow the Protocol Explicitly: Each RNA extraction method has specific steps that must be performed accurately. Whether using column-based techniques or magnetic bead approaches, adhering to the established protocol is crucial for optimal outcomes.
- Regularly Calibrate Equipment: Equipment like centrifuges and pipettes should regularly be calibrated to maintain precision. Inaccurate measurements can lead to unreliable results, so this is an important consideration in the RNA isolation process.
Optimizing Protocol Conditions
Optimizing the conditions under which RNA is isolated is equally important for ensuring high-quality results. Several factors can affect the efficiency and purity of the RNA:
- Adjusting pH Levels: The pH of the extraction buffers can markedly influence RNA stability. Typically, a pH around 7.5-8.0 is preferable for optimizing lysis and extraction processes.
- Temperature Control: RNA isolation should ideally occur at low temperatures to inhibit RNase activity. Keeping samples on ice or performing the extraction in a cold environment can significantly reduce RNA degradation.
- Volume Calculations: The volume of reagents should be optimized relative to the sample size. Under- or overestimating reagent volumes can result in lower yields or suboptimal purity levels. Ensuring that concentrations of reagents are suitable for the specific type of sample being processed is essential.
- Choosing the Right Kits and Reagents: Not all RNA isolation kits are created equal. Depending on the type of biological material, selecting the right kit can greatly improve the yield and quality. Researching and comparing various kits and their reviews can help in making an informed choice.
Applications of Isolated RNA
The isolation of total RNA opens numerous avenues for research, serving as a cornerstone in the study of gene expression and functionality. Each application derived from isolated RNA not only enhances our understanding of molecular biology but also contributes significantly to fields such as genetics, medicine, and biotechnology. This section provides an overview of the key applications of isolated RNA, emphasizing their importance and utility in modern research.
Quantitative PCR
Quantitative PCR, or qPCR, is a pivotal technique in the analysis of RNA expression levels. It allows researchers to quantify the amount of specific RNA in a sample, facilitating the understanding of gene regulation under various conditions. Through qPCR, it is possible to identify variations in gene expression, making it invaluable in studies related to disease states, developmental biology, and environmental responses.
The process is straightforward but requires high-quality RNA to achieve reliable results. This technique can detect low abundance transcripts with high sensitivity, making it possible to test samples taken from small quantities of material, such as biopsies or other precious samples. Moreover, the quantification enables comparisons between different samples, which is essential for understanding the dynamics of cellular processes.
Microarray Analysis
Microarray analysis represents a powerful method for examining the expression levels of numerous genes simultaneously. This high-throughput technology relies on isolated RNA, which is converted into labeled cDNA. The labeled cDNA is then hybridized to a microarray containing probes for thousands of genes.
Through microarray analysis, researchers can identify genes that are upregulated or downregulated in specific conditions. This is particularly useful in cancer research, where differential expression patterns can indicate potential biomarkers or therapeutic targets. The capability to analyze vast amounts of data allows for a comprehensive picture of gene interactions and regulatory networks.
RNA Sequencing
RNA sequencing, often referred to as RNA-seq, has transformed how gene expression studies are conducted. This method provides a detailed overview of the entire transcriptome, capturing both known and novel transcripts, including alternative splicing events. RNA-seq requires intact and pure RNA, thus underscoring the importance of effective RNA isolation protocols.
The insights gained from RNA sequencing are profound. It allows for quantifying transcript abundance and identifying transcript isoforms, all while harnessing the power of next-generation sequencing technology. This enables researchers to explore complex biological questions such as expression profiles in various tissues, differential gene expression, and the molecular mechanisms underlying diseases.
RNA-seq is revolutionizing transcriptomics, facilitating a shift from targeted analysis towards a more holistic view of gene expression.
Finale
One significant element discussed in this article is the impact of choosing the right isolation method. Different biological samples may require specific approaches or reagents, potentially affecting the extraction yield and purity. Researchers must consider their goals, the available equipment, and the nature of their samples when selecting methods.
Moreover, the benefits of thorough evaluation of RNA quality before proceeding with further analysis cannot be ignored. Employing techniques like spectrophotometry and agarose gel electrophoresis ensures that the RNA obtained is intact and pure enough for reliable results.
Ultimately, this comprehensive guide aims to arm both novice and experienced researchers with the knowledge needed to optimize their RNA extraction processes, leading to reproducible and reliable scientific outcomes.
Summary of Key Points
- RNA isolation is a fundamental technique in molecular biology with critical applications.
- The choice of extraction method can significantly affect the yield and purity of RNA.
- Evaluating RNA quality and quantity is crucial for accurate experimental outcomes.
- Addressing common challenges can enhance the reliability of RNA analysis.
Future Directions in RNA Research
Looking ahead, the field of RNA research is evolving rapidly. Innovations in RNA isolation techniques and advancements in sequencing technologies promise to open new frontiers.
- Automation of RNA Isolation: The integration of robotics and automated systems could streamline the RNA extraction process, reducing human error and increasing throughput.
- Novel Extraction Methods: Research into alternative extraction approaches may yield more efficient ways to isolate RNA from challenging samples, such as those with high levels of DNA or protein.
- Single-Cell Analyses: As technology advances, the ability to isolate RNA from individual cells will drive research into cellular heterogeneity and gene expression profiling.
- Integration with Bioinformatics: Enhanced computational tools can analyze RNA data at higher resolutions, making insights into gene regulation more accessible.
These prospects highlight the importance of ongoing research and education in RNA techniques, fostering a deeper understanding of RNA's complex roles in biology.