Transforming Plasmids into Bacteria: A Deep Dive

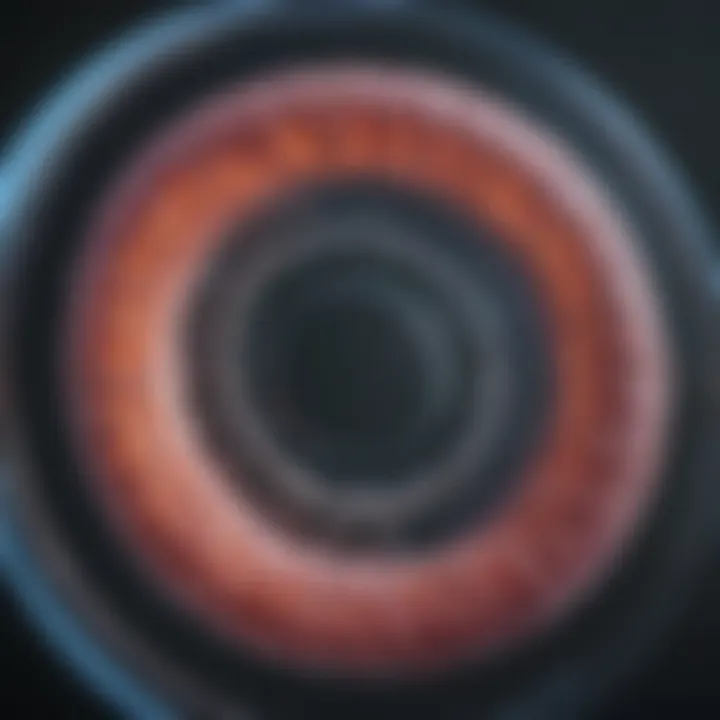
Research Overview
In the realm of genetic engineering, the method of transforming plasmids into bacteria stands as a cornerstone technique. This section provides a closer perspective on the importance of this process, shedding light on the intricate details that lay the foundation for numerous biotechnological applications.
Summary of Key Findings
The transformation of plasmids into bacteria enables researchers to manipulate the genetic material of bacterial cells. This manipulation is paramount for creating organisms that can produce valuable proteins, enzymes, or even pharmaceuticals. Significant advancements in this field have been observed, particularly with the advent of novel plasmids that enhance transformation efficiency and stability. In addition, understanding bacterial resistance mechanisms aids in improving the overall efficacy of these transformations.
Background and Context
The transformation process requires a comprehensive understanding of both plasmids and the bacterial cell structure. Plasmids are small, circular DNA molecules that exist independently of chromosomal DNA and are often used as vectors in genetic engineering. The microscopic world of bacteria plays host to a variety of transformation techniques, some naturally occurring and others artificially induced.
Research has shown that techniques such as heat shock, electroporation, and chemical transformation each offer unique advantages in differing contexts. These methodologies have grown more sophisticated, leading to enhanced transformation rates and fewer unintended consequences in the modified bacteria.
Such work has practical implications in diverse fields, from agriculture to medicine, where modified bacteria can be harnessed for pesticide production or the synthesis of vaccines. Exploring these applications lends insight into how transformation techniques can drive innovation in biotechnology, making it crucial for both students and professionals in the field to grasp the underlying concepts and methodologies.
"Understanding plasmid transformation is pivotal for advancing genetic engineering and unlocking new biotechnological possibilities."
Methodology
Examining the transformation of plasmids into bacteria involves precise planning and execution. The methodology must be robust to account for various biological variables and maintain scientific rigor.
Experimental Design
An effective experimental design typically includes a control group alongside transformed samples, facilitating clarity in data interpretation. Different strains of bacteriaβsuch as Escherichia coli or Bacillus subtilisβmight be selected based on the goals of the experiment. When setting up the design, researchers must consider:
- Type of plasmid utilized
- Method of transformation
- Selection markers for successful transformation
Data Collection Techniques
Robust data collection techniques are essential to evaluate the effectiveness of the transformation process. Strategies may involve:
- Growth observations through culture media
- PCR analysis for confirming plasmid presence
- Protein assays to assess expression levels
By combining these methodologies with a clear understanding of bacterial genetics, researchers can offer meaningful contributions to the field of genetic engineering.
Intro to Bacterial Transformation
Bacterial transformation is a vital process in molecular biology and genetic engineering. It allows for the introduction of plasmids into bacterial cells, which can lead to the production of proteins, the expression of genes, and the ability to confer new traits to the host organism. Understanding bacterial transformation is essential for various applications, including recombinant DNA technology, synthetic biology, and biotechnology. This article aims to delineate the intricate steps involved in the transformation process, providing a thorough understanding to students, researchers, and professionals alike.
Definition and Importance
Bacterial transformation is defined as the genetic alteration of a bacterial cell resulting from the uptake and expression of foreign DNA. This process can occur naturally in some bacteria or can be induced artificially in laboratory settings. The significance of this technique stretches beyond its immediate biological impact: it plays a crucial role in advancing our knowledge in genetics and its applications in medicine, agriculture, and environmental science. By enabling the manipulation of genetic material, bacterial transformation facilitates critical research that underpins innovations such as gene therapy and genetically modified organisms (GMOs).
Historical Background
The concept of bacterial transformation was first described in 1928 by Frederick Griffith, who demonstrated that non-virulent strains of Streptococcus pneumoniae could be transformed into virulent forms when mixed with heat-killed virulent strains. This foundational work set the stage for future research into the mechanics of DNA uptake in bacteria. The study evolved over decades, culminating in significant advancements during the late 20th century with the advent of recombinant DNA technology.
As research unfolded, many methods were developed to enhance transformation efficiency. An important development came in the 1970s with the advent of artificial transformation methods. Methods like the calcium chloride treatment and electroporation improved the ability to introduce plasmids into various bacterial strains. Such innovations heralded a new era in biotechnology, leading to the creation of genetically engineered microorganisms that have had substantial impacts across multiple industries.
"Bacterial transformation has opened pathways that were previously considered impossible in genetic engineering and synthetic biology."
Through these historical milestones, the process has evolved into a robust tool in laboratories worldwide. Today, bacterial transformation is a cornerstone in cloning, protein expression, and research on gene function, showcasing its profound relevance in modern scientific inquiry.
Plasmids: An Overview
Plasmids are essential components in genetic engineering and biotechnology. They are small, circular DNA molecules that replicate independently of the bacterial chromosome. Understanding their structure, function, and types is crucial for successful plasmid transformation into bacteria. This section delves into these aspects, emphasizing their significance in research and practical applications. Their varied properties and capabilities make plasmids a valuable tool in modern biology.
Structure and Function
Plasmids typically range from a few thousand to several hundred thousand base pairs in size. They comprise a double-stranded DNA structure, often containing genes that can offer a selective advantage to the bacteria, such as antibiotic resistance or metabolic functions. Plasmids can replicate independently, which allows them to increase in number within a host cell, thereby facilitating the spread of advantageous traits among bacterial populations.
The function of plasmids is diverse. They can carry genes that encode for proteins, regulatory elements, or even RNA molecules. Some plasmids are designed to act as vectors for gene cloning, while others may play a role in horizontal gene transfer, allowing bacteria to exchange genes directly through conjugation. Overall, plasmids serve as vital instruments for manipulating genetic material, enabling advancements in various fields, including medicine, agriculture, and bioengineering.
Types of Plasmids
Plasmids can be classified into several categories based on their characteristics and functions. Three major types are conjugative, non-conjugative, and cryptic plasmids.
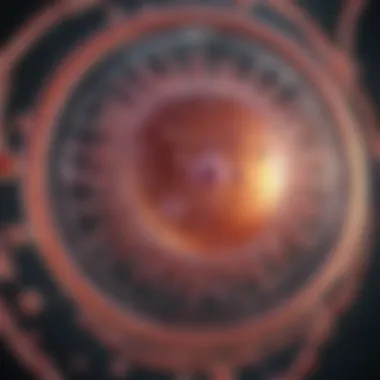
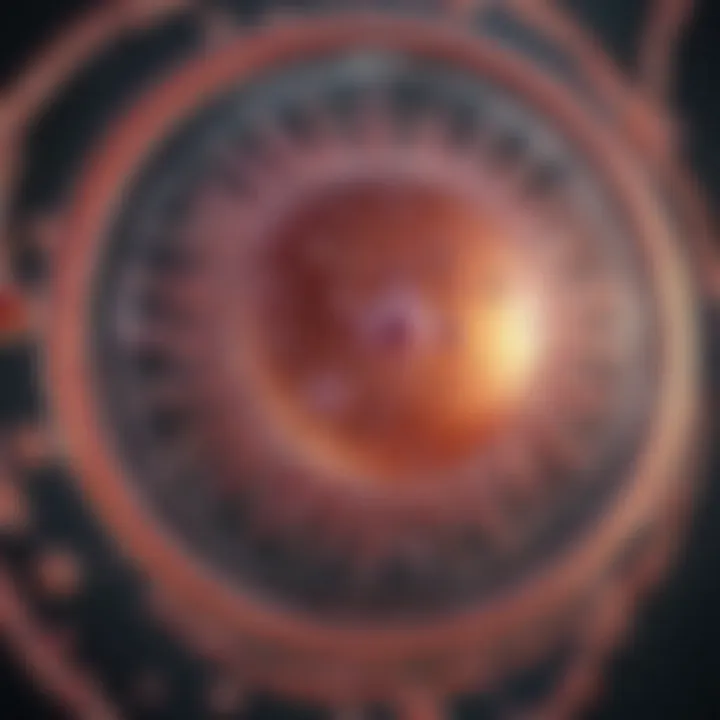
Conjugative Plasmids
Conjugative plasmids are notable for their ability to facilitate the transfer of genetic material between bacteria through a process known as conjugation. This characteristic is primarily due to the presence of specific genes termed tra genes, which enable the formation of a mating bridge between compatible bacteria.
The primary advantage of conjugative plasmids is their capacity for horizontal gene transfer, allowing beneficial traits, such as antibiotic resistance, to spread rapidly through bacterial populations. The critical feature is their mobility, making them an effective tool for genetic engineering efforts that aim to introduce new traits into microbial communities. However, their propensity for uncontrolled transfer can also result in unintended consequences, such as the rapid spread of resistance traits among pathogens.
Non-conjugative Plasmids
Non-conjugative plasmids lack the necessary genes for direct transfer between bacteria. Instead, they rely on other mechanisms for dissemination, such as transformation or being packaged in bacteriophages. Despite this limitation, non-conjugative plasmids are still invaluable in genetic research and biotechnology.
Their compact structure often contains essential genes that can be manipulated for various applications without the complications associated with conjugation. They offer researchers the benefit of stabilizing certain genetic traits within a single host. However, the restriction on mobility may reduce their efficacy in spreading desired traits across different bacterial species.
Cryptic Plasmids
Cryptic plasmids do not show any observable phenotype associated with their presence in a host. They may carry DNA that does not confer any known advantages to the bacteria or might be involved in poorly understood biological processes. The significance of cryptic plasmids lies in their potential role in bacterial evolution and adaptation.
Their primary characteristic is that they are often overlooked in studies, yet they contribute to the genetic diversity found within microbial populations. Understanding their function could reveal new insights into bacterial behavior and adaptability. While currently viewed as enigmatic, the exploration of cryptic plasmids could lead to breakthroughs in genetic manipulation and ecological studies.
"Plasmids represent a remarkable adaptability tool in nature, enabling bacteria to evolve and survive in changing environments."
Mechanisms of Transformation
Understanding the mechanisms of transformation is crucial in genetic engineering and the application of biotechnology. These mechanisms inform the processes by which plasmids are introduced into bacterial cells, enabling researchers to manipulate genetic material, produce proteins, and create genetically modified organisms. In this context, it is essential to differentiate between two primary methods: natural transformation and artificial transformation techniques. Both approaches have strengths and weaknesses that researchers must consider when planning experiments or applications.
Natural Transformation Processes
Natural transformation is a biological process observed in various bacterial species. It allows bacteria to uptake naked DNA from their environment and incorporate it into their genome. This process is essential for horizontal gene transfer, contributing to genetic diversity and evolution in bacterial populations. Natural transformation typically requires that bacteria enter a specific physiological state known as "competence." This state is often induced by environmental factors such as nutrient limitations or stress conditions.
Key benefits of natural transformation include its inherent efficiency and ability to maintain genetic stability. However, its reliance on specific conditions makes it less predictable in laboratory settings.
Artificial Transformation Techniques
Artificial transformation techniques enhance the ability to introduce plasmids into bacteria artificially. These methods overcome the limitations of natural transformation, allowing for a more controlled and efficient process. Below are three widely used artificial transformation techniques:
Calcium Chloride Method
The Calcium Chloride Method is a well-established approach based on treating bacterial cells with calcium ions. This treatment makes the cell membrane more permeable, facilitating the uptake of plasmid DNA. One major advantage of this method is its simplicity and cost-effectiveness, making it a popular choice in many laboratories.
Nonetheless, the method has limitations. The competence of cells can vary significantly, affecting transformation efficiency. The efficiency is also notably lower than some other techniques.
Electroporation
Electroporation involves the application of an electric field to create temporary pores in the bacterial cell membrane. This method allows for higher transformation efficiencies compared to the Calcium Chloride Method. Electroporation is highly versatile, as it can be used with various bacterial species and different types of plasmids.
However, the technique requires specialized equipment and parameter optimization to achieve desired results. Furthermore, cells may be damaged by the electric pulses if not properly calibrated.
Microinjection
Microinjection is a precise technique where plasmid DNA is directly injected into bacterial cells using a fine glass needle. This method allows researchers to bypass the barriers posed by the cell membrane, ensuring that a high percentage of cells receive the DNA. One of the key characteristics of microinjection is its ability to deliver relatively large DNA molecules, making it advantageous for specific applications.
On the downside, microinjection is labor-intensive and requires skilled personnel, making it less suitable for high-throughput applications. The potential for cellular damage during the process also poses a challenge.
Preparation for Transformation
Preparation for transformation is a crucial phase in the process of introducing plasmids into bacterial cells. This stage sets the foundation for the success of transformation by ensuring that both bacterial cells and plasmids are in optimal conditions for uptake. Proper preparation directly impacts transformation efficiency, which refers to the percentage of cells that successfully incorporate the plasmid DNA. High transformation efficiency is essential for maximizing the effectiveness of genetic engineering applications, including recombinant protein production and gene therapy research.
Culturing Bacterial Cells
Culturing bacterial cells involves growing bacterial strains in controlled environments. It is essential to select an appropriate medium that supports robust growth. Common media used include LB (Luria-Bertani) broth or more selective media depending on the strain in use.
- Inoculation: A starter culture is typically prepared by inoculating a small volume of fresh medium with a single bacterial colony. This allows the population to grow exponentially, promoting a healthy cell density suitable for transformation.
- Incubation Conditions: Maintaining optimal conditions is key. Generally, incubating at 37Β°C on a shaking incubator enhances aeration, thus supporting vigorous growth. Monitoring the optical density (OD) can help determine the right moment for harvesting the cells, with OD600 values around 0.4 to 0.6 often preferred for transformation protocols.
- Harvesting Cells: Once the desired cell density is reached, cells are collected by centrifugation. This step is critical, as it concentrates the cells, making them more amenable to the uptake process.
Effective culturing not only primes the bacterial cells for transformation but also plays a vital role in minimizing contamination risks, ensuring the integrity of the transformation process.
Isolating Plasmids
Isolating plasmids from a source organism is the next step in the preparation process. The quality and quantity of plasmid DNA impact the success of the transformation. The process generally involves the following key steps:
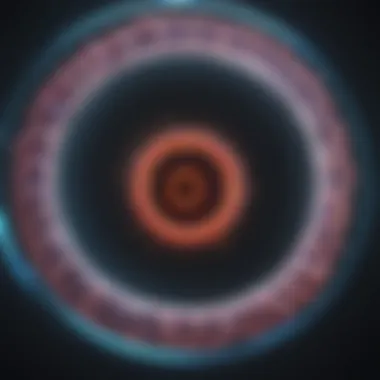
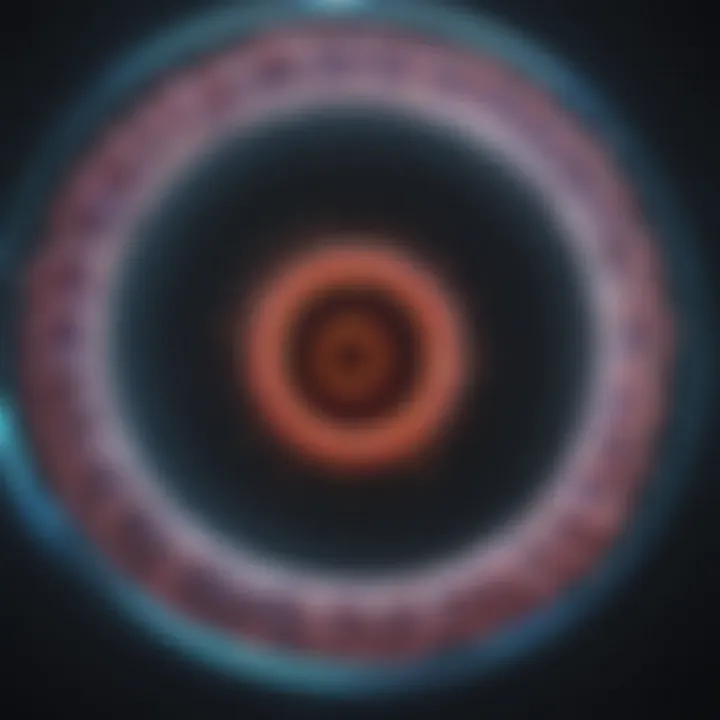
- Plasmid Extraction: Common methods for plasmid extraction include alkaline lysis followed by purification using spin columns that bind plasmid DNA. This allows for the separation of plasmid DNA from cellular debris, genomic DNA, and proteins.
- Purity Assessment: After extraction, assessing the purity of the plasmid is crucial. Spectrophotometry methods, often utilizing absorbance ratios (A260/A280), help evaluate nucleic acid purity. A ratio around 1.8 is typically indicative of pure plasmid DNA.
- Quantification: Accurate quantification of plasmid DNA is achieved using fluorometric assays or spectrophotometry. This information is vital to determine the appropriate amount of DNA to use in the transformation protocol.
Overall, isolating plasmids effectively prepares them for efficient introduction into bacterial cells, ensuring they carry the intended genetic information to achieve the desired outcomes.
Transformation Protocols
In the realm of genetic engineering, transformation protocols play a critical role. These protocols are the bridge connecting plasmids to bacterial cells. They define the methods utilized for the introduction of foreign DNA, explaining how the bacterial cells can incorporate and express this genetic material. Understanding these protocols can enhance transformation efficiency and optimize the desired outcomes in research and applied biotechnology.
Step-by-Step Procedures
The step-by-step procedures in transformation are essential for achieving successful uptake of plasmids by bacterial cells. Each step has distinct importance and contributes to the overall efficiency of the procedure.
Preparation of Competent Cells
Preparation of competent cells is a foundational step in transformation protocols. Competent cells are bacterial cells that have been treated to make their membranes permeable to DNA. This is crucial because natural bacterial cells do not readily take up DNA. One key characteristic of competent cells is their ability to facilitate high rates of transformation.
In this article, we discuss a popular choice: chemically competent cells. These cells are treated with calcium chloride, which enhances their ability to uptake plasmids. The unique feature of these cells is their reliability in various transformation settings. While they are simple to prepare and generally yield good results, the disadvantage is that they may not perform well with larger plasmids or those requiring high efficiency.
Mixing Plasmid with Cells
Mixing plasmid with competent cells is another critical aspect of the transformation process. This step allows the foreign plasmid to encounter the competent cells effectively. The key characteristic here lies in the conditions used during mixing, such as temperature and time. These factors influence the likelihood of successful uptake of the plasmid.
This article emphasizes the significance of gentle mixing. Gentle mixing ensures that the cells do not undergo stress, which could lead to cell damage. The unique feature of this gentle approach is that it maximizes the chances of successful transformation without compromising cell integrity. However, a potential disadvantage might be lower efficiency when compared to more aggressive mixing methods, which could lead to greater cell disruption.
Heat Shock or Electroporation Process
Heat shock and electroporation processes are two widely used techniques to facilitate DNA uptake. Both methods aim to alter the cell membrane's permeability temporarily, allowing plasmid entry. The heat shock method involves exposing cells to a sudden increase in temperature after they have been mixed with plasmids. This process is beneficial because it is simple and cost-effective, making it a popular choice for many labs.
In this article, we outline the advantages of heat shock: it typically provides good transformation efficiency for plasmids of reasonable size. However, one must consider that the efficiency may vary depending on the bacterial strain and plasmid used.
Electroporation, in contrast, uses an electric field to create temporary pores in the cell membrane. This method is characterized by its ability to efficiently transform a wide range of cell types, making it a robust option in transformation protocols. The unique benefit is that it allows the introduction of larger plasmids, which heat shock might not support as effectively. One drawback is the need for specialized equipment, which may limit accessibility for some researchers.
Post-Transformation Recovery
Following the transformation process, post-transformation recovery is critical for allowing bacteria to express the newly acquired plasmid. During this phase, cells need time to recover from the transformation shock, promoting cellular growth and replication of the plasmid. This step is often overlooked, but it is integral to ensure the highest yield of transformants. Proper recovery conditions can significantly enhance the success rate of experiments involving transformed bacteria.
Selection and Screening of Transformants
In the field of genetic engineering, selection and screening of transformants are crucial processes. They help identify bacterial cells that have successfully incorporated plasmids. This step is essential not only for confirming transformation but also for advancing research in various applications such as recombinant protein production and gene therapy. Without proper selection techniques, the efficiency and accuracy of experiments can significantly decrease. Therefore, understanding the principles behind these methods is vital for any researcher or practitioner working in the field.
Antibiotic Selection
The practice of antibiotic selection is a fundamental method used to identify transformed bacteria. After the transformation process, only the bacterial cells that have taken up the plasmid containing the antibiotic resistance gene can survive in the presence of that antibiotic. This selectivity is beneficial because it simplifies the identification of successful transformants. For instance, if a plasmid carries resistance to ampicillin, only the bacteria that contain this plasmid will thrive on ampicillin-containing media. However, reliance on this method has its limitations. The presence of antibiotic pressure can sometimes lead to unexpected mutations or the loss of the plasmid over time. Therefore, while antibiotic selection is effective, researchers must remain vigilant about potential long-term consequences.
Screening Methods
To further refine the identification of transformants, researchers employ various screening methods. These can complement antibiotic selection, providing a deeper insight into which cells have successfully taken up plasmids and expressed the desired genes.
Blue-White Screening
Blue-White Screening is a popular technique based on the use of beta-galactosidase, an enzyme that breaks down a chromogenic substrate called X-gal. When plasmids are designed with a lacZ gene, transformed cells will express the lacZ product. Cells that successfully incorporate the plasmid will appear blue due to the enzyme activity, while those without the plasmid will appear white. The key characteristic of this method is its simplicity and effectiveness. It allows researchers to quickly distinguish successful transformants from non-transformants. This is particularly beneficial because it can be integrated into routine workflows without extensive modifications.
However, one disadvantage of Blue-White Screening is that it requires the lacZ insertion site to be within the plasmid's multiple cloning site. Thus, not all plasmids can be used in this way.
Colony PCR
Colony PCR is another method frequently used for the screening of transformants. This technique involves directly picking a bacterial colony and performing PCR to amplify the target region of the plasmid. The advantage of Colony PCR lies in its speed and efficiency. It allows identification of successful transformants without the lengthy process of growing cultures and isolating plasmid DNA. If the PCR product is as expected, it indicates the presence of the plasmid in the selected colony. However, Colony PCR requires some optimization in the PCR conditions to avoid false negatives or non-specific amplifications.
In summary, both Blue-White Screening and Colony PCR offer unique advantages and limitations. Understanding these methodologies enhances the selection process and ensures a more successful transformation. This approach is critical in finding the most effective strategies in genetic engineering.
Applications of Bacterial Transformation
Bacterial transformation plays a crucial role in various fields of molecular biology and genetic engineering. By enabling the introduction of plasmids into bacterial cells, scientists can exploit the unique capabilities of these microorganisms for a range of practical applications. Understanding these applications provides insights into the significance of bacterial transformation and its influence on biotechnology.
Recombinant Protein Production
One of the foremost applications of bacterial transformation is the production of recombinant proteins. This process allows for the insertion of genes coding for specific proteins into plasmids, which are then introduced into bacteria like Escherichia coli. Once transformed, these bacteria can replicate rapidly, producing the desired protein in significant quantities.
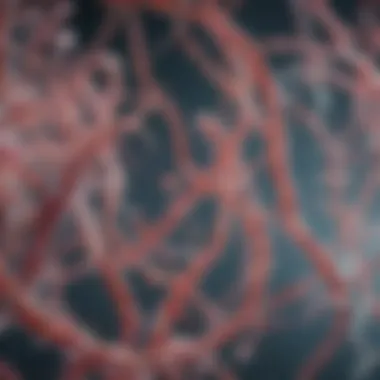
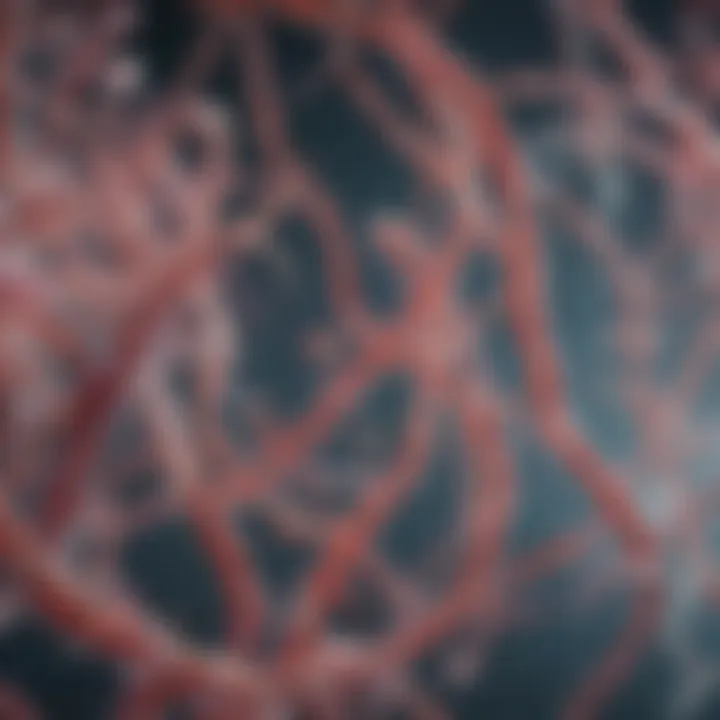
The production of insulin is a well-known example. The gene for human insulin can be inserted into a plasmid, and after transformation, E. coli can be induced to produce insulin that is functionally similar to its human counterpart. Such biotechnological applications not only provide essential therapeutic proteins but also enhance efficiency and lower production costs, making treatments more accessible.
Gene Cloning
Gene cloning is another vital application of bacterial transformation. This technique involves the replication of specific DNA fragments by introducing them into bacterial cells. This is achieved through inserting the target gene into a plasmid and then transforming the bacteria. Once the bacteria replicate, they produce multiple copies of the gene, which can be purified for further study.
This method is essential for creating gene libraries that researchers can utilize to study gene function and regulation. Moreover, gene cloning serves as a foundation for advanced research applications such as functional genomics and molecular mapping, which are necessary for understanding complex biological systems.
Gene Therapy Research
Gene therapy research is rapidly advancing, and bacterial transformation plays a significant role in this field. By using plasmids, researchers can explore potential therapies that involve the introduction or alteration of genes within human cells. Although bacteria are not used directly in human treatments, their ability to amplify and engineer plasmid DNA is critical for developing gene delivery systems.
For instance, researchers often use plasmids to assess the functionality of therapeutic genes before transitioning to more complex eukaryotic systems. However, careful consideration must be given to delivery methods and the potential immune response when transitioning to clinical applications. The outcomes of these studies can lead to significant advancements in treating genetic disorders, cancer, and infectious diseases, emphasizing the importance of basic research rooted in bacterial transformation methodologies.
"Bacterial transformation is a gateway for biotechnology, enabling innovations that enhance health and sustainability."
Challenges and Considerations
In any scientific process, challenges are often inevitable. When transforming plasmids into bacterial cells, there are specific aspects that researchers must consider, which can greatly influence the success of the transformation. Understanding these challenges is crucial, notably as they can affect the efficacy of the entire experiment. Whether a student, a researcher, or a professional in the field, knowing these factors can guide effective decision-making, improving overall outcomes.
Transformation Efficiency
Transformation efficiency refers to the effectiveness with which plasmids can enter host bacterial cells. A variety of factors can influence this efficiency.
- Cell competency: Not all bacterial cells can take up plasmids effectively. Preparations must ensure that the cells are competent, which means they can be transformed. This can be achieved through techniques such as treating cells with calcium chloride or using electroporation. Each method has its specific requirements for achieving optimal competency.
- Plasmid characteristics: Various features of the plasmid, such as size and design, also play a role. Large plasmids may have lower transformation rates due to their size. Moreover, the presence of specific sequences or markers can enhance or inhibit uptake.
- Environmental conditions: Conditions during the transformation process, like temperature and ionic strength, significantly impact efficiency. Generally, a carefully controlled environment will lead to a higher transformation success rate.
Researchers continuously seek methods to enhance transformation efficiency, for example, by developing more competent cell strains or employing advanced techniques. Better understanding of these nuances can lead to breakthroughs in transformation protocols in various applications.
Contamination Issues
Contamination is another significant challenge in plasmid transformation. Even minor contamination can compromise experimental results, leading to inaccurate conclusions. Various types of contamination can occur during the transformation process:
- Microbial contamination: This involves the introduction of unwanted microorganisms. It may occur from culture media, reagents, or even laboratory equipment. Ensuring a sterile environment is critical.
- Cross-contamination: When samples or reagents inadvertently mix, the integrity of the results can be placed in jeopardy. Use of dedicated tools for each sample can mitigate this risk.
- Chemical contamination: Impurities from reagents may also interfere. Quality control of chemicals is essential for reliable results.
Effective strategies to combat contamination include rigorous aseptic techniques, regular monitoring of reagents, and proper training of personnel involved in the transformation process. By prioritizing these considerations, researchers can improve the reliability of their experiments, making it easier to trust the results and conclusions drawn from them.
"Only a few small deviations in technique can lead to substantially differenet outcomes."
Managing challenges related to transformation efficiency and contamination requires awareness and strategy. The landscape of genetic engineering is always evolving, and staying informed about best practices can significantly enhance the perceived impact of research. By cultivating this awareness, the scientific community can drive future innovations within the field.
Future Perspectives in Transformation Research
The exploration of transforming plasmids into bacteria holds considerable significance in the field of molecular biology. It serves not only as a core technique in genetic engineering but also as a foundation for future research advancements. The future perspectives in this area provide insightful considerations that can shape and redefine the landscape of biotechnology. By examining innovative techniques and their implications, a clearer understanding of the benefits and challenges becomes evident.
Innovative Techniques
Advancements in innovative techniques have the potential to drastically enhance transformation efficiency and precision. Techniques such as CRISPR-Cas9 are emerging as transformative tools that can streamline genetic modifications. These methods offer targeted gene editing capabilities that surpass traditional transformation techniques. Additionally, advancements like microfluidics and single-cell analysis are gaining traction. These methodologies enable researchers to manipulate and analyze individual cells under controlled conditions, ultimately leading to improved outcomes in bacterial transformation.
Moreover, integration with artificial intelligence is becoming more prominent in optimizing transformation protocols. By leveraging machine learning algorithms, adjustments can be made in real-time to protocols based on empirical data, significantly refining the process.
Impact on Synthetic Biology
The implications of these advancements reach far beyond laboratory settings; they influence the broader field of synthetic biology. Transforming bacteria with plasmids opens up new avenues for the production of complex biomolecules, such as enzymes and pharmaceuticals. Researchers now aim to design synthetic biological systems that can solve real-world problems.
For instance, engineered bacteria may be utilized in bioremediation to detoxify pollutants or in the production of biofuels as sustainable energy alternatives. The impact of transformation research on synthetic biology aligns with global goals for sustainability and innovation.
The future of transformation research is not merely about understanding processes; it's about applying this knowledge to create solutions that address global challenges.
In summary, the future perspectives in transformation research accentuate a transformative journey driven by innovative techniques and their indirect influence on synthetic biology. Virtually every aspect of this research will continue to unfold new possibilities, driving advancements in health, environmental sustainability, and material science.
Ends
The topic of conclusions serves as a crucial element in understanding the full scope of transforming plasmids into bacteria. It encapsulates the essence of the preceding discussions, providing clarity on the importance of this process in genetic engineering and biotechnology.
Summary of Key Points
In this article, several key points have been made regarding the transformation of plasmids into bacteria.
- Bacterial Transformation: This is fundamental to genetic manipulation. It allows for the incorporation of new genetic material into bacterial cells, enhancing their functions.
- Plasmids: The structure and characteristics of plasmids have been thoroughly analyzed. Their diverse types, including conjugative and cryptic plasmids, hold significant roles in the genetic transformation process.
- Transformation Methods: Various methods, both natural and artificial, for transforming bacteria with plasmids were highlighted. These methods, such as electroporation and microinjection, showcase the technical advancements in this field.
- Applications: Practical applications, including gene cloning and recombinant protein production, were discussed. Understanding these applications helps to see the broader implications of plasmid transformation in research and industry.
- Future Perspectives: Emerging techniques and trends in transformation research point towards exciting developments in synthetic biology, which could shape future applications and innovations.
The Broader Impact
The broader impact of plasmid transformation in bacteria extends beyond laboratory boundaries. The implications are substantial across several fields:
- Biotechnology: This area benefits greatly as transformed bacteria can be used in pharmaceutical production, including the creation of vaccines and therapeutic proteins.
- Agriculture: Genetically modified bacteria can enhance crop production and pest resistance, contributing to more sustainable agricultural practices.
- Medicine: The advancements in gene therapy hinge on successful bacterial transformation, enabling treatment of genetic disorders and potential cures for diseases.
- Environmental Science: Microorganisms can be engineered for bioremediation efforts, helping to clean up pollutants and restore ecosystem balance.
The understanding and skills surrounding plasmid transformation into bacteria remain essential. As science progresses, it is vital to maintain an awareness of both the technical methods and ethical considerations involved in this field. This holistic view will ultimately foster responsible and innovative applications.