Translation of mRNA to Amino Acids Explained
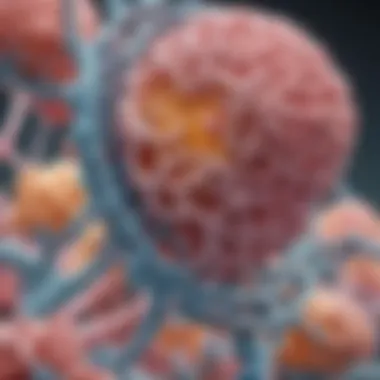
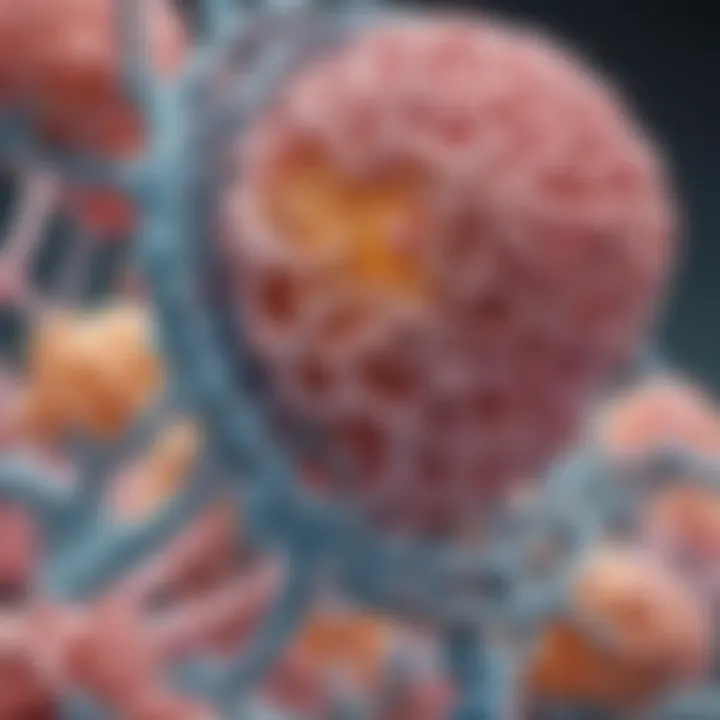
Intro
The translation of messenger RNA (mRNA) into amino acids is a pivotal step in protein synthesis. This intricate process is essential for life, as proteins play critical roles in cellular functions, structure, and regulation. Understanding how mRNA is decoded into proteins provides insight into the mechanisms that underlie many biological processes, including growth, repair, and immune responses. By exploring the various components involved, such as ribosomes and transfer RNA (tRNA), this article aims to clarify how genetic information is transformed into functional proteins.
Research Overview
Summary of Key Findings
Research on mRNA translation has unveiled several crucial components of the process:
- Ribosomes serve as the site for protein synthesis, facilitating the decoding of mRNA.
- tRNA molecules transport amino acids to the ribosome, matching them with the corresponding codons on the mRNA.
- Codons, sequences of three nucleotides on mRNA, dictate which amino acid is added to the growing polypeptide chain.
These elements work together in a highly coordinated manner to ensure accurate translation, which is fundamental for producing proteins that fulfill various roles in the body.
Background and Context
The translation process begins after mRNA is transcribed from DNA in the nucleus. Once mRNA leaves the nucleus, it travels to ribosomes in the cytoplasm. Ribosomes are made of ribosomal RNA (rRNA) and proteins, forming two subunits that come together during translation. This process is vital for maintaining cellular homeostasis and responding to environmental changes.
Understanding mRNA translation has significant implications in several fields:
- Genetic research: Insights into translation help in comprehending genetic disorders and variations.
- Biotechnology: Applications include the development of vaccines and therapeutic proteins.
- Medicine: A deeper understanding can lead to advances in treatments for diseases linked to protein misfolding or malfunction.
Methodology
Experimental Design
Investigations into mRNA translation often employ a variety of methodologies to explore how proteins are synthesized. Techniques such as:
- In vitro translation systems allow researchers to observe the translation process in controlled environments, providing valuable insights.
- Biochemical assays help in analyzing how various factors influence translation efficiency.
Data Collection Techniques
Data is gathered through physical analyses and molecular biology techniques:
- Sequencing technologies can identify the specific sequences of mRNA and the corresponding proteins.
- Spectrophotometry provides quantitative measurements of protein production, indicating the success of translation.
These methodologies are crucial for dissecting the nuances of the translation process. They enable scientists to better understand interactions among ribosomes, tRNA, and mRNA.
The translation of mRNA to amino acids stands as a fundamental biological process, essential for sustaining life.
Such insights foster a comprehensive understanding of how genetic information translates into functional units, crucial for ongoing research in biology and related domains.
Intro to mRNA Translation
Understanding the process of mRNA translation is critical in the field of molecular biology. It serves as the pillar for protein synthesis, which is essential for every cellular function in living organisms. This section provides a foundation for comprehending the subsequent details of how genetic information is transcribed into functional proteins. By grasping the intricacies of mRNA translation, researchers can appreciate its profound implications for genetic research, biotechnology, and medicine.
Defining mRNA and its Role
Messenger RNA, or mRNA, is a single-stranded molecule that acts as an intermediary between DNA and the synthesis of proteins. It carries the genetic code from DNA in the nucleus to the ribosomes in the cytoplasm, where proteins are produced. The sequence of nucleotides in mRNA encodes the order of amino acids in a protein, making it indispensable for translating genetic information into functional entities within the cell. This specificity is crucial, as any deviation in the mRNA sequence can lead to proteins that do not function as intended, thus affecting cellular processes.
Overview of Translation
The translation of mRNA to amino acids involves multiple steps and components that work in concert to ensure accurate protein synthesis. This comprehensive process includes the initiation, elongation, and termination phases, each with distinct molecular players such as ribosomes and transfer RNA (tRNA). During translation, the ribosome reads the codons of the mRNA, and the tRNA brings the corresponding amino acids to the growing polypeptide chain. Understanding the mechanics of this translation is essential, as it reveals how proteins are synthesized and modified post-translationally, highlighting its role in maintaining cellular function and stability.
The broader impacts of mRNA translation extend into various fields, influencing not only basic biological research but also innovative applications in medicine. Insights gained from studying this process drive advancements in gene therapies and vaccines, making it a topic of paramount importance in modern science.
Key Components of Translation
Understanding the key components of translation is essential for comprehending how mRNA is converted into functional proteins. Each element, from ribosomes to amino acids, plays a critical role in ensuring this intricate process runs smoothly. These components collaborate seamlessly to translate the genetic code embedded in mRNA into a sequence of amino acids, ultimately forming proteins essential for life.
Ribosomes: The Site of Protein Synthesis
Ribosomes serve as the machinery for protein synthesis, acting as the site where translation takes place. These complex structures are composed of ribosomal RNA (rRNA) and proteins. They can be found either floating freely in the cytoplasm or attached to the endoplasmic reticulum. The main function of ribosomes is to read the sequence of mRNA and facilitate the joining of amino acids in the correct order.
Ribosomes have two main subunits, the large subunit and the small subunit. The small subunit binds to mRNA first, scanning its length to find the start codon. Once located, the large subunit attaches, creating a complete ribosome ready for translation. This dynamic assembly allows ribosomes to interpret the mRNA sequence while coordinating with transfer RNA (tRNA).
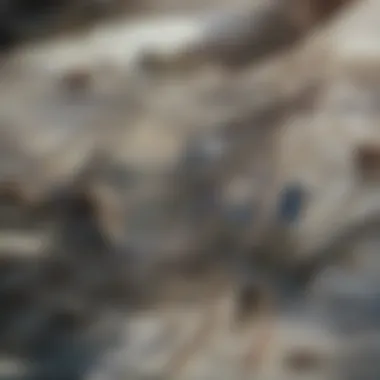
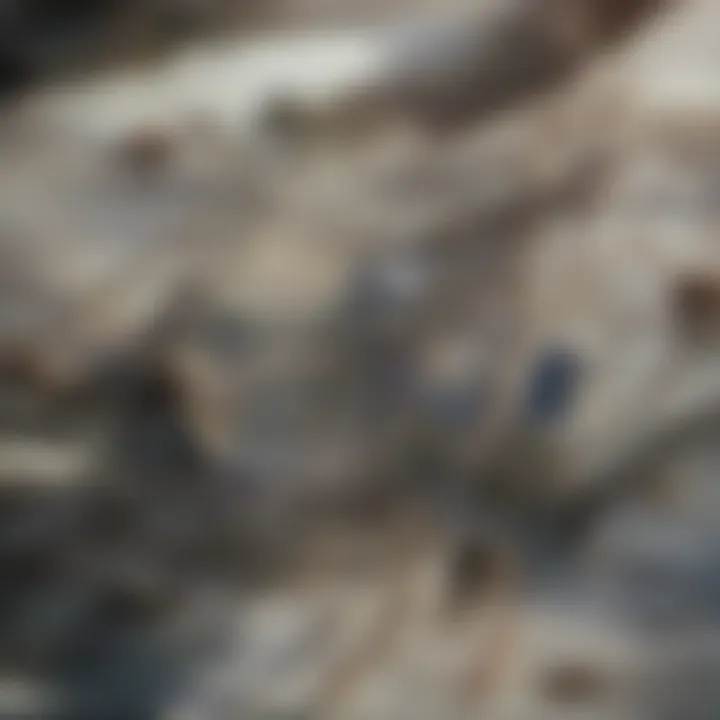
In essence, ribosomes are a crucial nexus of translation, forming the platform where the genetic code is enacted.
Transfer RNA (tRNA) Functionality
Transfer RNA, or tRNA, acts as the adapter molecule that interprets the mRNA codons and brings the corresponding amino acids to the ribosome. Each tRNA is specific to an amino acid and possesses an anticodon, a sequence of three nucleotides complementary to a codon on the mRNA strand. This specificity ensures that the correct amino acid is incorporated into the growing polypeptide chain.
During the elongation phase of translation, tRNA molecules bind to their respective codons on the mRNA. The ribosome facilitates the interaction, ensuring that the tRNA anticodons match the mRNA codons. Once bound, the ribosome promotes the formation of a peptide bond between the amino acids carried by two tRNA molecules. This process repeats, adding amino acids one by one until the entire protein is synthesized.
Amino Acids and Their Importance
Amino acids are the building blocks of proteins and are fundamentally important for countless biological functions. There are twenty standard amino acids, each characterized by a unique side chain (R group), which determines its properties and roles in proteins. The sequence and composition of amino acids in a polypeptide dictate the protein's structure and function, making their correct assembly during translation vital.
The availability of amino acids can influence translation efficiency. Cells obtain amino acids through diet or by synthesizing them. The balance of amino acids can affect cellular metabolism and protein synthesis. Deficiencies or imbalances can lead to functional impairments and various health issues.
In summary, the key components of translation—the ribosome, tRNA, and amino acids—interact in a highly orchestrated manner to facilitate the synthesis of proteins, shaping the landscape of cellular biology.
Mechanisms of mRNA Translation
The mechanisms of mRNA translation represent a pivotal aspect in the overall process of protein synthesis. Understanding these mechanisms is vital as they directly determine how the genetic code is translated into a functional polypeptide chain. This section elaborates on the intricacies of translation initiation, elongation, termination, and the modifications that occur post-translation. Each phase contributes to the efficiency and accuracy of synthesizing proteins, which are crucial for cellular functions.
Initiation Phase
The initiation phase is the first step in the translation process. It lays the groundwork for the assembly of the translation machinery. This phase begins with the formation of the initiation complex. The small ribosomal subunit recognizes the start codon on the mRNA and binds to it. In eukaryotic cells, this often involves several initiation factors that aid in stabilizing the interaction between the ribosome and the mRNA.
The initiator tRNA, which carries the amino acid methionine, then pairs with the start codon (AUG) on the mRNA. This codon is crucial as it designates the point where translation begins. Once the initiator tRNA is in place, the large ribosomal subunit joins the complex, completing the formation of the functional ribosome. Importantly, this phase ensures that the translation process begins with the correct amino acid sequence, which is determined by the mRNA template.
Elongation Phase
During the elongation phase, the ribosome moves along the mRNA strand, allowing tRNA to bring in amino acids sequentially. Each tRNA molecule matches its anticodon with the corresponding codon on the mRNA. As the ribosome moves, it facilitates the peptide bond formation between adjacent amino acids. This reaction is catalyzed by the rRNA present in the ribosome, emphasizing its role as a ribozyme.
The ribosome shifts down the mRNA in a process termed translocation. This allows the tRNA that was previously carrying the amino acid to exit the ribosome, making way for a new tRNA to enter. The elongation process continues until a stop codon is reached, creating a growing polypeptide chain which will eventually fold into its functional protein form.
Termination Phase
Termination occurs when the ribosome encounters a stop codon on the mRNA, which does not correspond to any tRNA. Instead, release factors bind to the ribosome, prompting the release of the newly synthesized polypeptide. This phase is crucial as it signals the end of translation and the completion of protein synthesis.
Upon termination, the ribosomal subunits dissociate from the mRNA. The mRNA can then be utilized in further rounds of translation or degraded, while the released polypeptide undergoes folding and further modifications. This process is essential for maintaining cellular function as proteins must reach their proper structure and stability to perform their intended roles.
Post-Translational Modifications
Post-translational modifications (PTMs) are essential for the functional maturation of proteins. After synthesis, polypeptides often undergo various modifications, which can include phosphorylation, glycosylation, and ubiquitination, among others. These modifications can alter a protein's activity, localization, and stability, greatly impacting its functionality within the cell.
For example, phosphorylation can activate or deactivate enzymes, while glycosylation is crucial for protein folding and stability in the extracellular space. Ubiquitination often marks proteins for degradation, thus regulating their lifespan within the cell. Understanding these modifications is critical as they play a significant role in cellular signalling and homeostasis, demonstrating the complexity of protein regulation in biological systems.
"Translation of mRNA to amino acids is a highly regulated and essential process for cellular function, and understanding its mechanisms provides insight into the dynamics of life itself."
The mechanisms of mRNA translation are fundamental to genetics and molecular biology. They not only elucidate how proteins are synthesized but also highlight the intricate regulation involved in cellular processes. As we explore further, recognizing variations in translation mechanistic pathways across different organisms will pave the way for advancements in biotechnology and medicine.
Codon Recognition and Decoding
Codon recognition is a fundamental aspect of the mRNA translation process. It ensures that the correct amino acids are incorporated into the growing polypeptide chain. This accuracy is critical for protein function and cellular health. Understanding codon recognition and decoding provides insight into how genetic information is expressed and translated into functional proteins that support life.
The Genetic Code
The genetic code is a set of rules that defines how sequences of nucleotide bases in the mRNA are translated into amino acids. It consists of codons, which are triplets of nucleotides. Each codon corresponds to a specific amino acid or a stop signal during protein synthesis. The universality of the genetic code across different organisms highlights its fundamental role in biology.
- Universality: The same codons specify the same amino acids in most organisms, suggesting a common evolutionary origin.
- Redundancy: Some amino acids are encoded by multiple codons. This feature adds flexibility to the genetic code, helping to mitigate the effects of mutations.
- Specificity: Each codon must be recognized accurately by transfer RNA (tRNA) to ensure the correct amino acid is added to the chain.
Codon-Amino Acid Correspondence
Codon-amino acid correspondence is the relationship between specific mRNA codons and the amino acids they encode. For instance, the codon AUG not only serves as the start signal but also codes for methionine. This specific pairing is vital for ensuring that proteins are synthesized correctly.
- Types of Codons:
- Amino Acids:
- Start Codon: AUG, which signals the beginning of the translation process.
- Stop Codons: UAA, UAG, and UGA, which terminate translation.
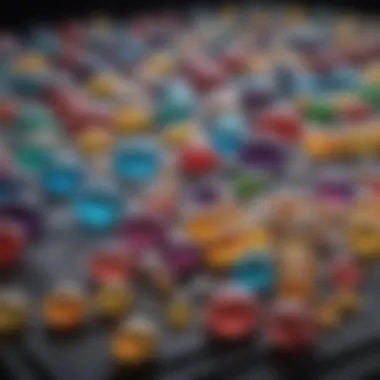
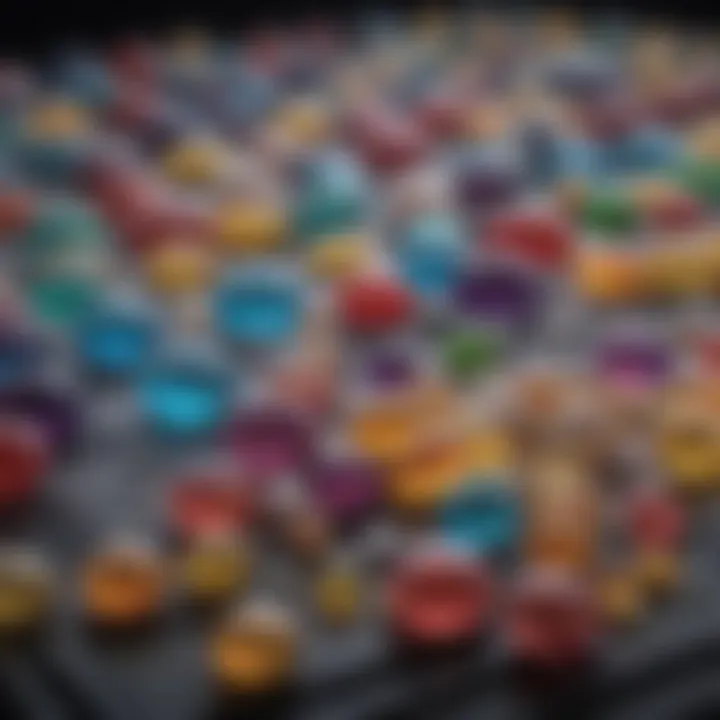
- Each of the 20 standard amino acids is linked to one or more specific codons.
- The precise pairing is crucial for maintaining the integrity of the protein structure and function.
"The accuracy of codon recognition is crucial for the proper synthesis of proteins, which in turn affects cellular function and overall organism health."
In summary, codon recognition and decoding are essential processes that bridge the gap between genetic information and functional proteins. The genetic code's structure, along with the correspondence between codons and amino acids, underpins the efficiency and accuracy of translation, making these mechanisms a focus of extensive research.
Factors Influencing Translation Efficiency
Translation efficiency is a critical aspect in the process of converting mRNA into amino acids, ultimately defining the rate at which proteins are synthesized. A variety of factors dictate how effectively this translation occurs, impacting cellular function and overall biological processes. Understanding these factors is essential for research in genetics, biotechnology, and cellular biology.
Concentration of Biological Components
The concentration of various biological components plays a vital role in translation efficiency. Each element involved, from ribosomes to tRNA and amino acids, must be present in adequate quantities to facilitate smooth translation.
- Ribosomes are the core machinery for translation. If ribosome levels are too low, they might become a bottleneck in the translation process since mRNA strands may accumulate without being translated into proteins.
- tRNA molecules are responsible for carrying the correct amino acids to the ribosome. A higher concentration of tRNA corresponding to frequently used codons can enhance translation speed and accuracy. Conversely, insufficient tRNA can lead to slow translation or mistakes in amino acid incorporation.
- Amino acids themselves must also be abundant. The availability of free amino acids directly affects the ability to build the polypeptide chains. When supply is limited, protein synthesis can be inhibited, impacting cellular health.
These concentrations create a systemic balance that is necessary for the efficiency of translation, as any imbalance—whether too much or too little—can affect how genes are expressed and how proteins function in biological systems.
Environmental Factors
Translation efficiency is not solely dependent on internal factors; external environmental conditions also exert significant influence. Temperature, pH levels, and the ionic strength of the cellular environment all contribute to the functionality of ribosomes and other translation components.
- Temperature: Optimal temperatures are necessary to maintain the structural integrity of the ribosomal complex and tRNA. Extreme temperatures, either high or low, can denature proteins or inhibit enzymatic functions, slowing translation.
- pH Levels: The pH of the cellular environment affects the ionization state of amino acids and tRNA molecules, which can impact binding and interaction with ribosomes. An appropriate pH is critical for enzymatic processes during translation.
- Ionic Strength: The concentration of ions in the cellular environment influences the stability of RNA and protein structures. Higher ionic strength can enhance interactions between molecules, potentially aiding translation, while lower levels may destabilize these interactions.
As such, the translation process operates optimally only within a narrow range of environmental conditions. Recognizing and manipulating these factors can lead to improved outcomes in research and practical applications in fields such as biotechnology.
Translation in Eukaryotes vs. Prokaryotes
Understanding the distinctions in mRNA translation between eukaryotes and prokaryotes is crucial for comprehending the overall dynamics of protein synthesis. Each domain of life employs unique mechanisms and processes that influence how genetic information is interpreted and utilized. The significance of these differences transcends basic biology; they layer into biotechnology applications and therapeutic strategies. Knowing how translation operates in these two realms informs researchers on drug design, gene therapy, and synthetic biology.
Eukaryotic Translation Mechanisms
Eukaryotic translation mechanisms involve a series of complex processes designed to ensure precise and regulated protein synthesis. The process initiates with the formation of the initiation complex, which consists of the small ribosomal subunit, initiation factors, and the initiator tRNA. The ribosomal complex attaches to the 5' cap of the mRNA, which is vital for proper binding and recognition.
A notable aspect of eukaryotic translation is the presence of multiple initiation factors. They not only help the ribosome assemble on the mRNA but also play roles in recognizing the correct start codon. When the start codon is located, the large ribosomal subunit joins the complex, forming the active ribosome.
The elongation phase follows, where tRNA molecules ferry amino acids to the ribosome. Eukaryotic cells utilize elongation factors that assist in the binding of tRNA to the ribosomes, significantly impacting the translation speed and accuracy. Additionally, ribosomes in eukaryotes are often found in a polysome configuration, allowing for multiple translations of a single mRNA strand simultaneously, which enhances protein production.
Finally, once the ribosome encounters a stop codon, the termination phase takes place. Release factors recognize the stop codon and facilitate the release of the synthesized polypeptide chain. This complex system signifies a more elaborate process in eukaryotes than in prokaryotes, allowing for increased regulation and diversity in protein synthesis.
Prokaryotic Translation Mechanisms
Prokaryotic translation mechanics, in contrast, are simpler and occur in a more streamlined process. There is no compartmentalization in prokaryotic cells; mRNA translation begins even before transcription has finished. The ribosome can bind to the mRNA shortly after it begins to be transcribed. This concurrent nature allows for rapid protein synthesis.
The initiation complex consists of the small ribosomal subunit, the mRNA strand, and a special initiator tRNA. In this case, Shine-Dalgarno sequences play a pivotal role in guiding the ribosome to the start codon by pairing with rRNA in the ribosomal subunit, facilitating a more efficient setup for translation.
During elongation, prokaryotic translation involves elongation factors but in less intricate forms than in eukaryotes. The efficiency of this phase is supported by the fact that the initiation phase promptly transitions to elongation without the need for numerous regulatory factors.
Termination occurs via a similar mechanism; when the ribosome reaches a stop codon, release factors guide the ribosome in releasing the newly formed polypeptide. This process is generally quicker and less regulated compared to eukaryotic processes, rendering prokaryotic translation both efficient and effective for the needs of these organisms.
In summary, while both eukaryotes and prokaryotes translate mRNA into proteins, the mechanisms exhibit fundamental differences rooted in complexity, regulation, and efficiency. These differences are significant in various scientific fields, including research into genetic diseases and developments in biotechnology.
Implications of mRNA Translation
The implications of mRNA translation are profound, influencing various realms of biology and medicine. Understanding this process not only enriches our comprehension of cellular mechanisms but also supports advancements in genetic engineering, therapeutic development, and more. Each element we explore—the role of mRNA, protein functions, and the interaction between genetic material and phenotypic expression—highlights the intricacy of life at the molecular level.
Role in Cellular Function and Homeostasis
mRNA translation is vital for maintaining cellular function and homeostasis. Proteins, synthesized through this translation process, serve as the workhorses of the cell, carrying out tasks such as catalyzing biochemical reactions and providing structural support. Without adequately translated mRNA, cells cannot produce the proteins necessary for processes like enzymatic reactions, signaling pathways, and metabolic regulation. An imbalance in protein synthesis can lead to cellular dysfunction, which may manifest as diseases.
Moreover, specific proteins regulate homeostasis, controlling the internal environment of cells. Enzymes ensure metabolic pathways operate correctly, while structural proteins maintain cell integrity. Therefore, the accurate translation of mRNA into functional proteins is crucial to sustaining life and ensuring organisms can adapt to changing conditions.
Significance in Genetic Research
The significance of mRNA translation extends deeply into genetic research. By studying the mechanics of this process, researchers can uncover how genes express traits in organisms. Understanding gene expression can have profound implications for developmental biology, cancer research, and variations in genetic disorders.
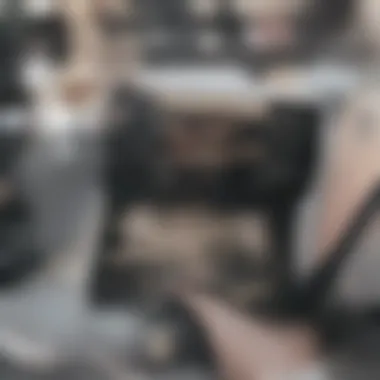
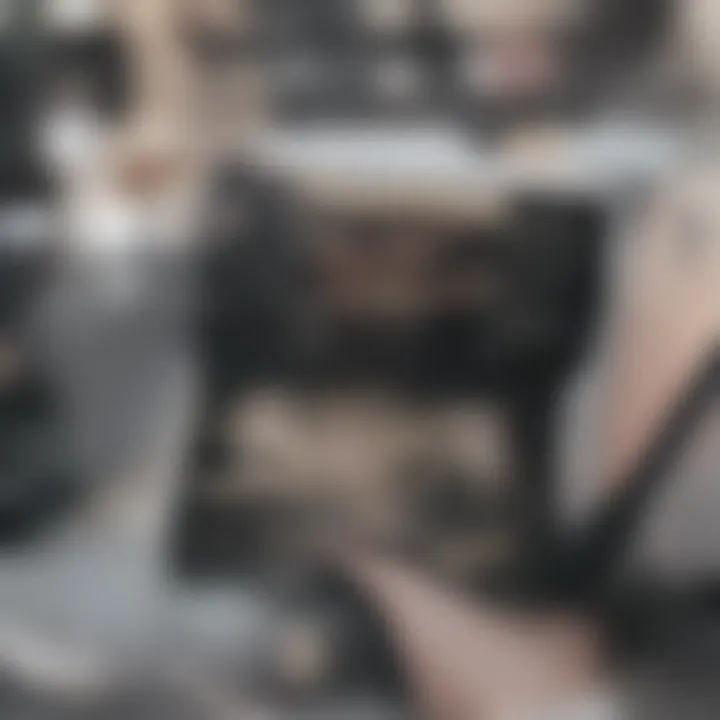
When researchers manipulate translation mechanisms, they can elucidate how external factors influence gene expression. For instance, next-generation sequencing technologies allow for an in-depth examination of how cells respond to varying environments at a genetic level. Insights drawn from these studies guide the development of personalized medicine approaches, tailoring therapies based on individual genetic profiles.
Applications in Biotechnology and Medicine
In biotechnology and medicine, the applications of mRNA translation research are expansive. One of the most notable advances is the development of mRNA vaccines, such as those created for COVID-19. These vaccines harness the body's cellular machinery to produce a harmless piece of the virus, prompting an immune response without introducing the pathogen itself.
Furthermore, understanding translation has led to innovative therapies targeting genetic disorders. Strategies like gene editing using CRISPR-Cas9 depend heavily on the manipulation of mRNA translation to correct faulty genes or enhance protein production. The potential for treatments that target protein synthesis directly offers new avenues for addressing previously untreatable conditions.
The advances in mRNA translation research are not just scientific achievements; they represent significant strides towards addressing global health challenges.
Through these applications, mRNA translation continues to shape the future of medical and biotechnological advancements, demonstrating its importance in both theory and practice.
Challenges in Translation Studies
The study of mRNA translation encounters various challenges that are vital for the comprehensiveness and precision of our understanding of protein synthesis. Recognizing these challenges can lead to insightful developments in both biochemical research and applied sciences. One key area of interest is how amino acid codon bias can influence translation efficiency. This bias refers to the preference for certain codons over others in different organisms, affecting how effectively proteins are synthesized. It has implications in both evolutionary biology and biotechnological applications. Researchers must consider codon bias when developing synthetic genes or designing expression systems, as it can lead to suboptimal protein yields.
Another challenge lies within translational regulation mechanisms. Regulation of translation is a complex process influenced by numerous factors, such as RNA sequences, structures, and the abundance of regulatory proteins. Understanding these mechanisms provides insights into how cells adapt their protein synthesis according to specific needs or environmental changes. Failure to grasp these regulatory pathways can lead to misinterpretation of data or flawed applications in genetic engineering.
These challenges highlight the need for continued research in the field. Addressing them improves our understanding of cellular functions and the overall resilience of biological systems. As techniques evolve, researchers can better examine these complexities to unlock the secrets of efficient protein synthesis.
"Understanding the challenges in translation studies is crucial for advancing molecular biology and developing effective biotechnological solutions."
Amino Acid Codon Bias
A significant aspect of translation studies is amino acid codon bias, which describes the uneven usage of synonymous codons in the genetic code. Different species exhibit distinct preferences for certain codons, even though multiple codons may encode the same amino acid. This bias can have profound effects on translation efficiency, stability of mRNA, and overall protein production.
Codon usage can influence several translational outcomes:
- Translation Speed: Certain codons can be synthesized more rapidly than others due to the availability of corresponding tRNA molecules. If a gene contains a high frequency of less common codons, the ribosome might stall, leading to inefficiency.
- Protein Folding: The speed of translation affects how newly synthesized polypeptides fold. Incorrect folding can lead to malfunctioning proteins and cellular stress.
- Gene Expression Levels: Codon bias can dictate how much protein is ultimately produced. For instance, genes optimized for a particular organism can be translated at higher rates when they utilize the preferred codons of that organism.
In biotechnology, considering codon bias becomes pivotal when designing synthetic genes for expression in host organisms. Harmonizing codon usage with the host cellular machinery enhances protein yield and activity, which is essential in producing therapeutics and other biochemical products.
Translational Regulation Mechanisms
Translational regulation mechanisms are crucial for fine-tuning protein synthesis in response to cellular conditions and demands. These mechanisms orchestrate when and how translation occurs, impacting cellular function significantly. Various elements contribute to translational regulation, including:
- 5' and 3' Untranslated Regions (UTRs): These non-coding regions flanking the coding sequence can influence the translation process, affecting the accessibility of the ribosome. Specific motifs within UTRs can enhance or inhibit translation based on cellular signals.
- RNA Binding Proteins: Proteins that bind to mRNA can modulate its stability and translational efficiency. They can act as repressors or activators, responding to various internal cues, such as nutrient availability or stress conditions.
- MicroRNAs: These small RNA molecules can bind to targeted mRNA transcripts, leading to degradation or translational repression. MicroRNAs play a significant role in regulating gene expression at the post-transcriptional level.
Inherent complexities arise due to the interplay of these regulatory mechanisms. Disruptions in translational regulation can lead to diseases and may offer intriguing insights into cancer biology and genetic disorders. Thus, elucidating these mechanisms is essential for the development of targeted therapeutic strategies in medicine.
Continued investigation into these regulatory elements will enhance our understanding of translation as well as its broader implications in cellular dynamics.
Current Trends in Translation Research
The study of translation research is evolving rapidly, fueled by advancements in technology and a deeper understanding of molecular biology. Current trends highlight the significance of integrating new methods and approaches to unravel the complexities involved in mRNA translation to amino acids. Researchers are increasingly recognizing the need for precise and innovative techniques to explore the nuances of protein synthesis.
One major focus is the application of high-throughput techniques. These methods significantly enhance the ability to analyze large quantities of biological data quickly. For instance, next-generation sequencing allows scientists to sequence millions of DNA strands simultaneously, offering insights into the translation landscape at an unprecedented scale. Such capabilities enable researchers to identify variations in translation efficiency or monitor changes in protein expression levels across different conditions.
"Advancements in sequencing technologies are crucial for understanding the translation process and its regulation."
In addition to sequencing techniques, there is a growing emphasis on characterizing the translational landscape comprehensively. This involves studying the various factors that can impact protein synthesis, such as ribosome dynamics, tRNA availability, and the influence of upstream regulatory sequences. Through advanced modeling and computational approaches, researchers can create more precise simulations of translational processes, leading to better predictions and insights into biological outcomes.
Next-Generation Sequencing Techniques
Next-generation sequencing (NGS) techniques represent a leap forward in molecular biology research. These methods have fundamentally transformed the way scientists study the genome and transcriptome, influencing our understanding of translation. NGS provides a comprehensive view of gene expression, allowing researchers to quantify mRNA levels accurately and assess how they vary under different conditions.
Some key benefits of NGS in translation research are:
- Increased Speed and Throughput: NGS can sequence a vast number of samples in a short time, enabling large-scale studies that were previously unfeasible.
- High Resolution: The resolution of NGS allows for the detection of rare transcripts, providing a more detailed understanding of potentially overlooked molecules that play roles in translation.
- Insights into Post-Transcriptional Regulation: NGS facilitates the identification of alternative splicing events and RNA editing, both of which can influence the translation of specific genes.
While traditional sequencing methods focus primarily on the DNA sequence, NGS encompasses both DNA and RNA analysis, yielding a wealth of data. The integration of NGS into translational research is paving the way for discoveries that can have implications in genetic studies, drug development, and understanding diseases at the molecular level.
Innovative Approaches to Study Translation
Researchers are embracing innovative methodologies to study translation, aiming for a more holistic understanding of the process. Some trending approaches include:
- Ribosome Profiling: This technique captures a snapshot of ribosome positions on mRNA molecules, providing insight into which codons are being translated at any given moment. This data can reveal the dynamics and efficiency of translation across different cellular conditions.
- CRISPR-Based Techniques: The application of CRISPR technology is enhancing the ability to manipulate specific genes directly, allowing researchers to study the effects of targeted mutations or deletions on translation. This has profound implications for understanding gene function and regulation.
- Fluorescence Microscopy: Advanced microscopy techniques enable the visualization of translation in live cells. By tagging proteins or mRNA with fluorescent markers, researchers can observe the dynamics of translation in real-time, offering unprecedented insights into the spatial aspects of protein synthesis.
By adopting these innovative approaches, the scientific community is advancing its understanding of translation. These techniques illuminate the intricacies of the process, allowing for discoveries that could lead to breakthroughs in healthcare, biotechnology, and fundamental biology.
In summary, the current trends in translation research indicate a merging of traditional methods with cutting-edge technologies. Next-generation sequencing and innovative experimental approaches are setting the stage for a deeper insight into the mechanisms of translation, with far-reaching implications for various fields.