Understanding the AAV Model: Insights and Implications
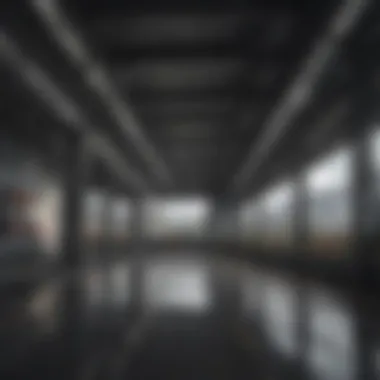
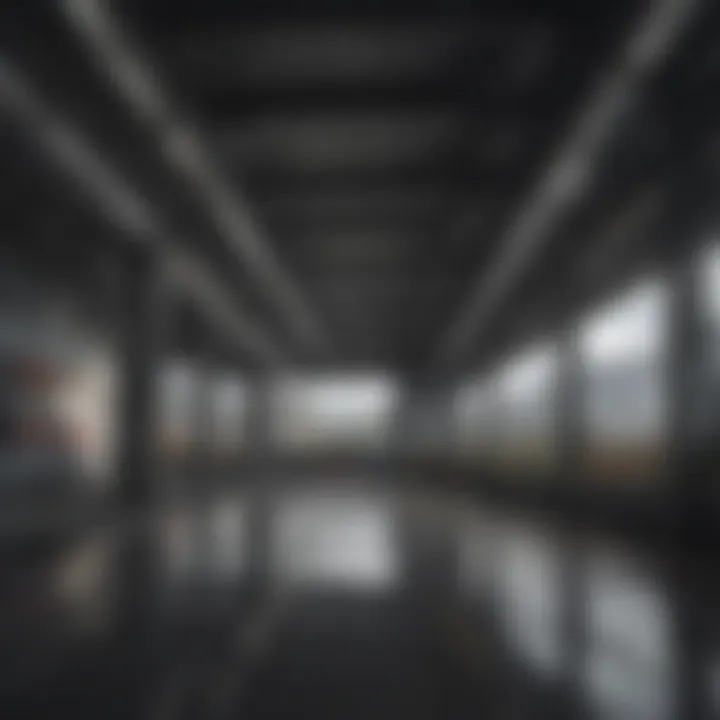
Intro
The AAV model, while not always at the forefront of conversation, carries substantial weight in the realm of gene therapy. It's a subject interwoven with intricate scientific principles that bridges the gap between theoretical research and practical applications. Here, we dive into the nuances of AAV vectors, their utilization in delivering genetic material effectively, and the broader implications of these mechanisms in precision medicine.
Gene therapy itself has undergone significant evolution over the past few decades, transforming from a fledgling concept into a powerful tool with the potential to treat a variety of genetic disorders. At the heart of this transformation lies the AAV model, often referred to as a 'workhorse' in gene delivery. Understanding its architecture and operational mechanisms is essential for anyone looking to grasp the current advancements in biological research.
Research Overview
In order to fully appreciate the impact of the AAV model, one must first consider its essence and the surrounding landscape. This overview aims to consolidate our understanding of AAV vectors, elucidate their roles, and offer insight into their potential.
Summary of Key Findings
- AAV Vectors: These are non-pathogenic viruses that play a critical role in the delivery of genetic material. Their ability to stably incorporate themselves into the host genome sets them apart from other delivery methods.
- Applications: Ranging from treating genetic disorders to utilizing in cancer therapies, the versatility of AAV vectors opens numerous doors in therapeutic avenues.
- Innovation: Recent advancements focus on increasing the capacity of AAV vectors to deliver larger genes, while fine-tuning their targeting abilities to specific tissues or cells.
Background and Context
The context surrounding AAVs is rich and complex. Adeno-associated viruses, discovered in the 1960s, were initially deemed insignificant. However, as the science behind gene therapy continued to develop, so did the understanding of these viruses. Researchers recognized the potential of AAV vectors not only for their safety profile but also for their efficacy in transducing various cell types.
These developments have changed the landscape of how genetic diseases are addressed therapeutically. It presents a shift from merely symptom management to direct genetic modifications, reshaping how we approach complex diseases.
Methodology
Understanding the inner workings of AAV models requires a clear, systematic approach to examining their applications in gene therapy. Here, we outline the methodology typically employed in the research and development of AAV-based treatments.
Experimental Design
Typically, the study of AAV involves a well-thought-out experimental design, including:
- Vector Construction: Creating modified AAV vectors designed to carry and deliver specific genes of interest.
- In Vitro Studies: Conducting tests in laboratory settings to observe the behavior of these vectors in cell cultures.
- Animal Models: Utilizing animal subjects to better assess the vectors’ efficacy and safety prior to human trials.
Data Collection Techniques
Data collection on AAV models involves several techniques, such as:
- PCR (Polymerase Chain Reaction): Amplifying DNA segments to study the presence of the therapeutic gene introduced by AAV vectors.
- Sequencing: Analyzing the genomic integration of the AAV vector.
- Flow Cytometry: Assisting in the quantification of gene expression levels in targeted cells.
Through these strategies, researchers engage in a thorough exploration of AAVs, continuously refining their understanding and paving the way for innovative therapies.
"The AAV model stands as a testament to the power of innovation in navigating the challenges of gene therapy. It embodies the shift towards precision medicine, where the focus lies on tailored solutions for complex genetic disorders."
Prelude to AAV Models
The significance of AAV (Adeno-Associated Virus) models in modern science cannot be understated. Positioned at the intersection of gene therapy and advancements in biotechnology, these models offer a unique method to deliver genetic material into host cells. As researchers push the boundaries of medical science, understanding the AAV model is crucial for unraveling intricate biological processes and developing innovative treatments for genetic disorders, cancer, and neurodegenerative diseases.
Defining AAV
AAV, or Adeno-Associated Virus, is a small, non-pathogenic virus that belongs to the Parvoviridae family. It is often employed as a vector in gene therapy due to its safety and efficiency in transferring genetic material into targeted cells. Unlike many other viral vectors, AAVs generally do not trigger substantial immune responses, making them a favorable choice for therapeutic interventions. These vectors can be engineered to carry therapeutic genes, allowing them to effectively deliver these genes into human cells for potential disease management. This aspect of AAV is not just a footnote in scientific journals; it has led to significant breakthroughs in various fields of medicine.
Historical Context
The journey of AAV began in the mid-1960s when researchers first identified this virus in the context of cellular infections. Fast forward to the 1990s, and AAV vectors started capturing interest in gene therapy circles. Researchers were keen to explore AAV's potential due to its ability to integrate itself into the host genome with relatively low risk of causing unintended mutations, thus unlike retroviral vectors. Clinical trials exploring AAV vectors gained momentum, paving the way for major advancements in therapies for inherited diseases. As the years went by, studies unveiled the potential of AAV vectors in treating not just rare genetic disorders but also more prevalent diseases like hemophilia and certain types of muscular dystrophy.
Current Relevance
In today's rapidly evolving landscape of genetic engineering, AAV models stand at the forefront of innovation. With breakthroughs in genome editing, the relevance of AAV has only grown. Recent scientific investigations have expanded the use of AAV vectors in several therapeutic applications, including genetic vaccines and therapies aimed at neurodegenerative conditions like Parkinson's disease. The ability to customize AAV serotypes also enables targeted delivery to specific tissues, thereby enhancing the therapeutic response and minimizing side effects.
"AAV vectors are not merely tools; they symbolize a significant leap towards precision medicine, offering hope to many ailing from genetic disorders."
The ongoing research into AAV's mechanisms, safety, and effectiveness is invaluable. As researchers delve deeper into understanding and optimizing these viral vectors, they illuminate the path towards novel treatment modalities, promising not just to alleviate suffering but also to potentially cure debilitating conditions. The current dialogues around AAV models reflect a growing appreciation for their utility in various therapeutic contexts, making them a hot topic in scientific and medical communities alike.
Mechanisms of AAV Vectors
The mechanisms of AAV vectors are paramount in understanding how they contribute to gene therapy advancements. Their unique characteristics allow for effective gene delivery, promising avenues to tackle various genetic disorders. A deeper look into their structural attributes, replication cycle, and transduction process gives clarity on why AAVs are at the forefront of biomedical research.
Structure of AAV
Adeno-associated viruses (AAV) are small, non-pathogenic viruses that rely on helper viruses for replication. Their structure is remarkably simple yet sophisticated. At the core, AAV consists of a single-stranded DNA genome encapsulated within a protein coat, commonly referred to as a capsid.
The capsid is composed of 60 protein subunits arranged in an icosahedral symmetry, which is crucial for its stability and function. Notably, AAV can come in various serotypes, each with distinct capsid proteins. This diversity in serotypes allows for tailored approaches to gene therapy since different serotypes exhibit varying tissue tropism and immunogenicity, making them suitable for specific applications in the body. For instance, AAV2 has a preference for neurons, while AAV9 shows strong heart tropism.
"Understanding the structure of AAV vectors is akin to drawing a blueprint for gene therapy; it defines how we can approach treatment."
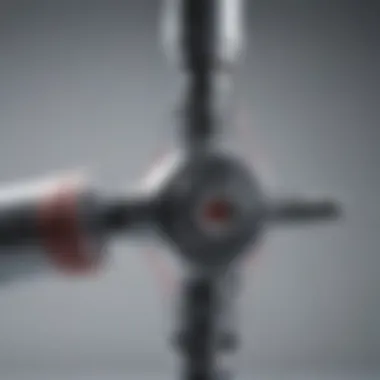
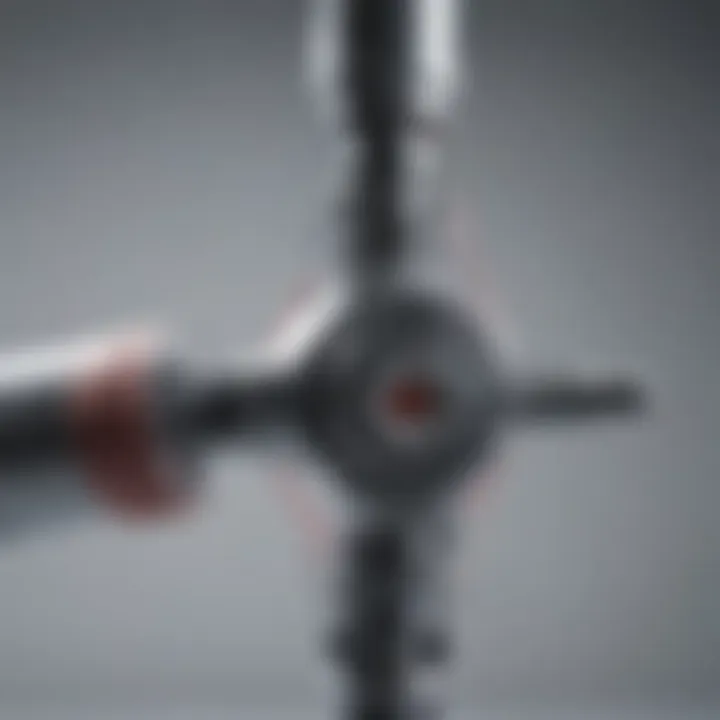
Replication Cycle
The replication cycle of AAV is a fascinating saga that requires insight into its interactions with host cells. AAV can either integrate into the host genome or exist as an episome. This cycle divides into several crucial steps: attachment, entry, and replication.
- Attachment: AAV first binds to the surface receptors of the target cell. This binding is facilitated by specific proteins on the AAV capsid, which recognizes various receptors on the cell surfaces.
- Entry: Following attachment, the virus enters the cell through endocytosis. Once inside, the acidic environment of the endosome triggers conformational changes in the capsid, allowing the viral genome to escape into the cytoplasm.
- Replication: After escaping the cytoplasm, the single-stranded DNA is converted into a double-stranded form, allowing for expression and replication using the host's cellular machinery. This phase is vital as it determines how effectively foreign genes can be expressed within the target tissues.
The intricate dynamics of this cycle not only dictate the efficiency of gene delivery but also lay the groundwork for future innovations in AAV technology.
Transduction Process
The transduction process describes how AAV vectors transfer therapeutic genes into target cells. Once AAV has entered the host cell and bypassed the endosomal membrane, it encounters a series of internal cellular pathways. The therapeutic DNA is unpacked, and critical viral proteins assist in the integration into the host's cellular mechanisms.
During the transduction, the therapeutic gene can either integrate into the genome or remain as an episome. If it integrates, it allows for sustained expression, which is an essential trait in treating chronic conditions.
Furthermore, the efficiency of transduction is often influenced by the type of AAV serotype used, the delivery method, and the target tissue. The ability to pinpoint and modify these factors underscores the versatility of AAVs in gene therapy.
In summary, understanding the mechanisms of AAV vectors is crucial to unlocking their potential in medical applications. By delving into their structure, replication cycle, and transduction process, researchers and practitioners can better design therapies that address a myriad of genetic disorders, pushing the frontiers of science forward.
Applications of AAV Models in Gene Therapy
Adeno-associated virus (AAV) models have established themselves as a cornerstone in the landscape of gene therapy. These models facilitate unique approaches aimed at treating a range of genetic disorders and diseases, improving patient outcomes in ways that were once thought unimaginable. Their versatility, when paired with a safety profile that is quite impressive, has opened up new avenues for researchers to explore.
In this section, we will delve into specific aspects of AAV applications in gene therapy including how they help in treating genetic disorders, their role in addressing neurodegenerative diseases, and their significance in cancer therapy.
Treating Genetic Disorders
Genetic disorders arise from anomalies in DNA, often leading to substantial challenges for affected individuals. Traditional therapies sometimes miss the mark when it comes to addressing the root causes of these disorders. AAV-based therapies present a method to directly target and correct the genetic defects at play.
One prominent example involves AAV vectors delivering therapeutic genes to replace faulty ones. Consider Spinal Muscular Atrophy (SMA)—a condition that causes weakness and atrophy in muscle tissues. By using AAV vectors to deliver the SMN1 gene, researchers have observed remarkable improvements in muscle function in clinical trials. This is just one illustration of AAV's potential impact in this domain.
Key benefits of AAV in treating genetic disorders include:
- Long-lasting effects: AAV can achieve stable expression of therapeutic genes, often eliminating the need for frequent re-treatment.
- Specificity: AAV vectors can be engineered to target specific tissues, enhancing therapeutic outcomes while minimizing side effects.
- Immunomodulation: These vectors can evade the immune system to a degree, enabling the delivery of sustained therapeutic doses even in patients with pre-existing immunity.
Neurodegenerative Diseases
Neurodegenerative conditions, such as Alzheimer’s and Parkinson’s disease, pose an increasing burden on healthcare systems worldwide. These disorders are characterized by gradual neuron deterioration, ultimately leading to loss of cognitive and motor functions. AAVs hold promise for these conditions, primarily through their abilities to deliver neuroprotective factors and promote neuronal regeneration.
In experiments, AAV vectors have been utilized to introduce genes associated with neuroprotection into animal models of diseases like Huntington’s disease. For instance, introducing BDNF (Brain-Derived Neurotrophic Factor) via AAV has shown potential in elevating neuronal resilience and functionality. This approach provides dual support by mitigating cell death while bolstering the health of existing neurons.
Moreover, AAVs can:
- Enable localized delivery: By injecting AAV vectors directly into the brain, it's possible to enhance the potency of the treatment while minimizing systemic exposure.
- Encourage novel approaches: Combining AAV therapy with other treatments could lead to synergistic effects that may be more effective than either method alone.
Role in Cancer Therapy
Cancer therapy has a reputation for being complex and challenging. AAV models step into this arena with innovative strategies that include delivering genes that can either suppress tumor growth or enhance the immune response. This strategy brings forth the idea of gene therapy as a complementary approach to conventional cancer treatments.
One application is using AAV to introduce genes that promote the expression of cytokines. For instance, delivering interleukin-12 (IL-12) through AAV vectors has shown promise in preclinical studies aimed at creating an immune response against tumors. In essence, this has the potential to turn a patient's immune system into a more effective fighter against cancer cells.
The advantages of AAV in cancer therapy include:
- Targeted action: AAV can be engineered to target specific types of tumors, potentially safer than systemic therapies which affect healthy cells as well.
- Sustained expression: AAV vectors can maintain therapeutic gene expression over extended periods, potentially reducing the need for multiple treatments.
In summary, AAV models are part of a transformative trend in gene therapy, offering promising avenues for treating genetic disorders, neurodegenerative diseases, and cancer. Their unique properties enhance both the effectiveness and safety of therapeutic interventions, making AAV vectors an essential tool in the ongoing fight against some of the most challenging medical conditions.
Advantages of AAV Models
The exploration of AAV models marks a crucial juncture in the advancement of gene therapy. These models do not merely act as vehicles for delivering genetic material but embody a paradigm shift in therapeutic strategies. To fully appreciate the efficacy of AAV models, one must delve into specific elements that set them apart in medical research and treatment scenarios.
Safety Profile
When it comes to gene therapy, the safety profile of any vector must be foremost in consideration. Atypical of many viral vectors, AAVs display a notably low immunogenicity. This means that they tend to elicit a minimal immune response from the host’s body. Researchers have documented instances where subjects treated with AAV vectors exhibited fewer adverse effects compared to other gene delivery systems. This aspect can be a game-changer in sensitive patient populations, particularly when treating conditions with historical safety concerns.
For example, clinical trials involving AAV-mediated therapies for diseases such as spinal muscular atrophy reported an enviable safety profile. The documentation from these trials has shown that many participants only experienced mild, transient reactions. This vital characteristic enhances the appeal of AAVs as safe therapeutic tools, which could foster more widespread acceptance and usage in clinical settings.
Therapeutic Efficacy
Moving from safety to therapeutic efficacy, AAV models offer remarkable potential in altering disease trajectories. The ability of AAVs to deliver therapeutic genes into target cells efficiently sets a solid foundation for their success. Long-term expressions of delivered genes have been noted in various studies, showcasing sustained therapeutic outcomes, which are crucial for chronic or genetic conditions.
- Noteworthy Examples:
- Hemophilia: AAV gene therapy has shown promise in correcting deficiencies, allowing patients to lead lives with fewer limitations.
- Retinal Diseases: Trials with AAV-delivered genes have resulted in significant improvements in vision in patients with inherited retinal disorders.
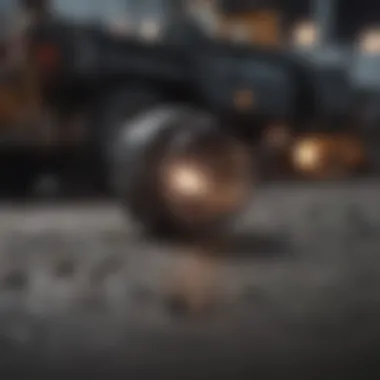
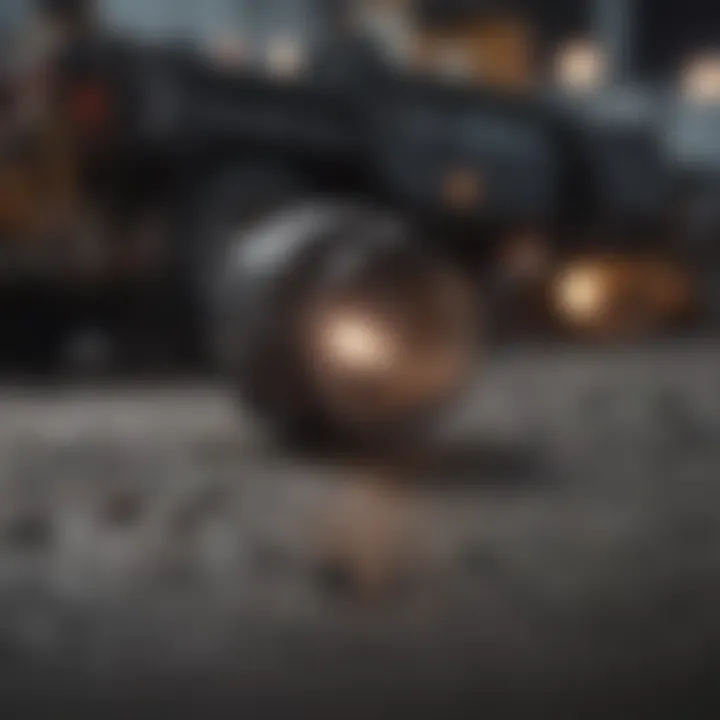
This efficacy is key for expanding the range of conditions that AAVs can address, including but not limited to genetic disorders, cancer, and beyond. Continued advancements in vector engineering are likely to enhance this efficacy further.
Versatility of Serotypes
AAV's versatility of serotypes is another notable advantage. With multiple serotypes available, researchers can strategically choose specific AAV variants that exhibit preferential tropism for various tissues. This allows for more targeted delivery of therapeutic genes, increasing the likelihood of successful treatment outcomes.
- Examples of AAV Serotypes and Their Targets:
- AAV2: Primarily targets the central nervous system, making it suitable for brain-related therapies.
- AAV8: Often utilized for liver-targeted therapies, beneficial in metabolic diseases.
This targeted approach can be paramount in reducing off-target effects and enhancing treatment precision, thus marrying the concept of efficacy with patient safety.
The multifaceted advantages of AAV models highlight their potential as transformative tools in gene therapy, fundamentally altering therapeutic landscapes while maintaining safety and efficacy.
Challenges in AAV Research
Understanding the challenges associated with AAV research is essential for delineating the potential and limitations of this pivotal technology in gene therapy. While AAV models hold great promise for therapeutic applications, several roadblocks can impede progress. Thoroughly analyzing these challenges sheds light on necessary considerations and opens avenues for innovative solutions. Below are critical challenges that researchers face in advancing AAV technology.
Immunogenic Responses
One of the most pressing concerns in AAV research is the immune response elicited by AAV vectors. The body's immune system tends to recognize foreign entities, and AAV vectors are no exception. When introduced, they can trigger an immune reaction that neutralizes the vectors before they can effectuate the desired genetic transfer. This immunogenicity not only hampers the efficacy of AAV-mediated therapies but can also lead to adverse reactions in patients.
Factors contributing to these immune responses include the serotype of the AAV vector used, previous exposure to AAV in the population, and individual variations among patients.
- Previous Exposure: Many patients may have already been exposed to AAVs naturally, resulting in existing antibodies that could impede vector uptake.
- Serotype Selection: The choice of AAV serotype is crucial as different serotypes elicit varying immune responses.
Understanding and mitigating these immune interactions is key. Researchers are developing strategies to enhance vector design and delivery methods to minimize immunogenic responses. For instance, employing immunosuppressive protocols or preemptive administration of anti-inflammatory agents may improve outcomes.
Limited Packaging Capacity
Another significant hurdle in AAV research is the limited packaging capacity of AAV vectors. AAVs can typically accommodate around 4.7 kb of genetic material. This restriction can pose a significant obstacle when attempting to deliver larger genes or multiple therapeutic sequences simultaneously. The limitation can mean that certain diseases that require a larger gene replacement or multiple genes cannot be addressed effectively using standard AAV vectors.
Strategies to tackle this limitation are being explored, such as:
- Modified Vectors: Scientists are experimenting with engineering AAVs to increase their packaging capacity.
- Dual Vector Systems: Using two separate AAV vectors to deliver different parts of a larger gene represents a promising avenue. This approach can involve a split gene strategy where one vector carries one segment and another vector delivers the complementary segment, necessitating precise reassembly within the host.
While much hope rests on overcoming this packaging challenge, researchers continue to find innovative measures, ensuring that gene therapy remains adaptable for a wider range of conditions.
Targeting Specific Tissues
The ability to effectively target specific tissues with AAV vectors is crucial for maximizing therapeutic outcomes. Different tissues exhibit distinct barriers, which can interfere with the efficacy of gene delivery. Without precise targeting, even if vectors are successfully administered, therapeutic effects may prove disappointing due to inadequacies in reaching the desired cells.
Several factors complicate tissue targeting:
- Tissue-Specific Expression: Different tissues have unique characteristics, which necessitate the use of serotypes with affinity to those specific cells. The mismatch may lead to suboptimal gene expression.
- Biological Barriers: The blood-brain barrier in the central nervous system presents a notorious challenge in targeting brain tissues using AAVs. This barrier prevents many therapeutic entities from reaching their intended site.
Innovations in research are underway, aiming to overcome these limitations:
- Targeting Ligands: Coupling AAVs with targeting ligands ensures that the vectors can specifically home in on desired cells.
- Adaptive Delivery Systems: Developing adaptive delivery mechanisms that can dynamically adjust based on the target tissue characteristics plays a promising role in enhancing tissue-specific targeting.
Recent Advances in AAV Technology
Advancements in AAV technology are making waves in gene therapy, enhancing both the effectiveness and scope of treatments available today. The relevance of these developments cannot be overstated as they pave the way for innovative therapeutic strategies. First off, scientific curiosity and a growing need for precision medicine fuel recent breakthroughs, highlighting the benefits that can potentially arise from such advancements in vector engineering. Investigating the nuances in this area reveals a promising landscape, especially in how these innovations could address current limitations in gene therapy.
Engineering Enhanced Vectors
Engineering enhanced vectors stands as a vital component in the evolving narrative of AAV technology. By incorporating advanced genetic edits, researchers can significantly improve the transduction efficiency of vectors. This means that the vectors not only improve responsiveness in target cells but also enhance the overall delivery of the genetic material. One notable approach includes developing hybrid vectors, which combine features from multiple serotypes for greater efficiency.
Moreover, scientists are utilizing techniques like CRISPR-Cas9 to tailor AAV vectors for specific applications. This level of customization brings researchers closer to targeting hard-to-reach tissues, which is often a major hurdle in the field of gene therapy. This engineering shift is not merely incremental; it represents a paradigm shift.
Combination Therapies
Combination therapies using AAV vectors are gaining traction. They entail pairing an AAV vector with other treatment modalities, such as small molecules or even checkpoint inhibitors in cancer therapy. The idea is to amplify the therapeutic efficacy of gene delivery while minimizing potential side effects. Research suggests that using combination approaches might improve patient outcomes for complex conditions that do not respond well to single-agent therapies.
By blending traditional methods with AAV technology, the overall treatment landscape becomes multifaceted. For instance, in combating certain forms of muscular dystrophy, combining a gene-editing AAV vector with an anti-inflammatory drug may substantially boost recovery chances and muscle preservation. Researchers are still navigating the waters of optimization, assessing how these combinations can achieve the best synergistic effects.
Regulatory Prospects
Looking ahead, regulatory prospects for AAV technology play a crucial role in its advancement and application. As these techniques mature, regulatory bodies are sizing up the need for new guidelines tailored specifically for AAV-based therapies. Ensuring safety and efficacy while facilitating patient access will necessitate a thorough understanding of long-term effects—a topic that requires keen observation and continual learning.
Furthermore, the role of transparency in regulatory processes cannot be downplayed. Scientists and pharma companies must provide clear data substantiating their claims. This is especially pertinent when considering the gains made through engineering advancements and combination therapies. Regulatory bodies like the FDA and EMA are starting to lay down frameworks that can accommodate the unique aspects of AAV technology, allowing faster transitions from lab to clinical settings.
In summary, recent strides in AAV technology are captivating. By focusing on better vector engineering, exploring combination therapies, and understanding the shifting regulatory landscape, we can observe a future enriched with possibilities for more effective gene therapy. > "The pace at which AAV technology evolves could redefine not only gene therapy but also our comprehension of treating complex diseases."
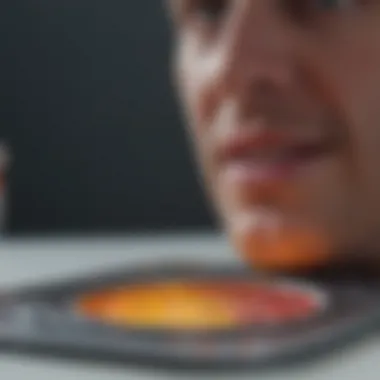
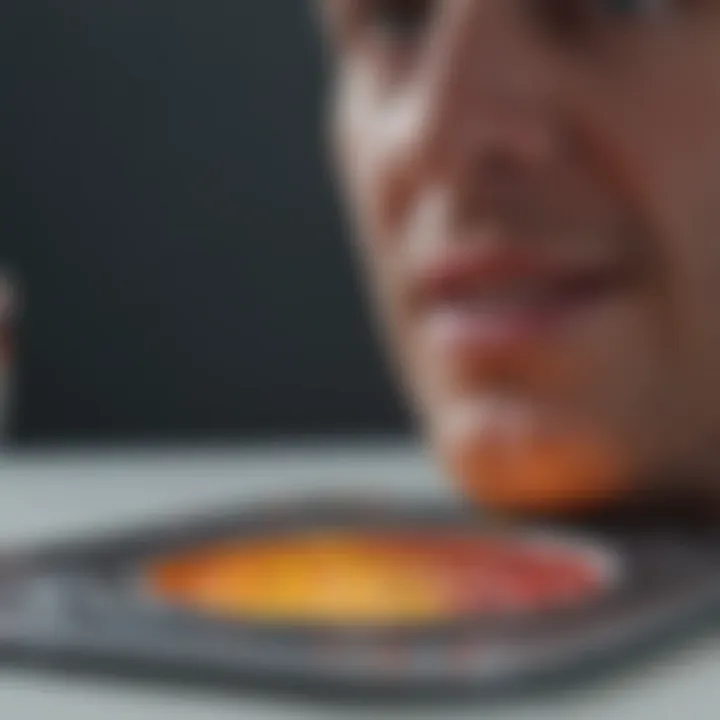
While there remain challenges to overcome, it's clear that this area of research is poised to offer impactful solutions, revolutionizing how we approach treatment possibilities in medicine.
Ethical Considerations in Gene Therapy Using AAV
Understanding the ethical landscape surrounding gene therapy, particularly with AAV models, is not just important, it's essential. The rapid advancements in genetic technologies invite a plethora of ethical questions that need careful consideration. Failing to address these concerns can lead to public mistrust and regulatory hurdles that might slow down promising research and therapies. This section delves into some pivotal ethical aspects, primarily focusing on informed consent, long-term effects, and accessibility and equity.
Informed Consent
Informed consent is a cornerstone of ethical practice in medicine and research. It ensures that patients are fully aware of and understand the procedures they will undergo, the risks involved, and the expected benefits. In the context of AAV gene therapy, informed consent has layers of complexity.
For instance, patients might not be familiar with the technicalities of vector delivery systems, and the impact of genetic modification can be daunting. Researchers and healthcare professionals must go beyond standard information sheets. They need to offer clear and concise explanations of what gene therapy entails, what viruses like AAV do, and the specific targets within their genetic makeup. Here’s a few points that can help clarify this process:
- Use layman's terms when discussing genetic concepts.
- Ensure ongoing dialogue: consent isn’t just a one-off event; it should be revisited as new information arises.
- Engage family members or caregivers in the discussion to provide additional support for the patient.
"Properly navigating informed consent can mean the difference between a hopeful patient and one who is overly anxious about the treatment."
Long-Term Effects
When considering gene therapy using AAV, one cannot ignore the potential long-term effects. While immediate benefits may be evident, the long-range possibilities pose significant ethical questions. The reality is that genetic modifications can have unpredictable repercussions that may not manifest until years later.
Researchers need to actively monitor patients long after they receive treatment. This long-term follow-up is vital for several reasons:
- Evolving Understanding of Genetics: As scientific knowledge grows, more insights may come to light about how AAV-mediated changes affect health over time.
- Adverse Effects: There could be unforeseen side effects that arise long after treatment.
- Heritability: If germline editing is involved, there's a question of how modifications might be passed to future generations.
The quest for balancing benefits and risks is a challenging path; thorough ethical frameworks need continuous development, ensuring all potential outcomes are assessed and ready to be reported back to participants.
Accessibility and Equity
Last but not least, accessibility and equity in gene therapy demonstrate the social implications of scientific advancements. The capacity for AAV models to revolutionize treatment for genetic disorders is clear, but there remains a stark reality — not everyone has equal access to these groundbreaking therapies.
Some critical points include:
- Cost of Treatments: The financial burden of gene therapy can be exorbitant, limiting access to only a select few.
- Geographical Barriers: Not all regions have the facilities or expertise to provide AAV-based therapies.
- Insurance Coverage: Insurance policies can often be ill-equipped to provide adequate coverage for experimental treatments like gene therapy.
Promoting equitable access isn't merely an ethical obligation; it is a necessity for ensuring that advancements in medicine truly benefit all. Collaborative efforts must be encouraged between governments, medical institutions, and companies to level the playing field, making sure the promise of AAV models reaches those who need it most.
Future Directions for AAV Research
The landscape of gene therapy is rapidly changing, and AAV (Adeno-Associated Virus) research is no exception. The future directions of AAV research present both exciting opportunities and complex challenges. As we navigate through this era of technological innovation, exploring smarter ways to enhance vector delivery and expand therapeutic applications holds immense promise. These advancements not only aim to cure existing diseases but also to pave the way for understanding the potential of gene editing tools in personalized medicine.
Innovative Approaches to Vector Delivery
Innovative methods in vector delivery are crucial for maximizing the effectiveness of AAVs. Traditional delivery mechanisms often encounter barriers such as immune responses and limited tissue targeting. Addressing this issue has led to several novel strategies aimed at improving the precision and efficiency of AAV delivery systems.
- Targeted Delivery Systems: Researchers are actively developing constructs that could target specific tissues more effectively. Employing ligands or cell-specific promoters allows for a more tailored approach to gene therapy, potentially reducing the dosage required and minimizing side effects.
- Nanoparticles and Co-delivery Platforms: The integration of AAVs with nanoparticles or liposomes is being extensively explored. This combination not only enhances stability but can also facilitate the transport of a wider range of genetic materials.
- Optimizing Serotype Selection: Different serotypes of AAV have unique tropisms, which influence their ability to target particular tissues. By engineering AAVs with improved serotypes suited for specific diseases, the effectiveness of therapeutic interventions can be notably increased.
These innovative approaches to vector delivery are vital to ensuring that AAV therapy not only becomes more precise but also more accessible to patients who could greatly benefit.
Expansion of Therapeutic Applications
The future of AAV research is intrinsically linked to expanding its therapeutic applications. As our understanding of genetics deepens, AAVs are being evaluated for a growing number of conditions beyond hereditary diseases. This extension signifies a shift toward a broader application of gene therapy methods.
- Infection Control: There is emerging interest in using AAV vectors as a means to combat viral infections. This could revolutionize the way diseases like HIV are managed, shifting the focus from symptomatic treatment to genetic interventions.
- Chronic Diseases: AAVs are being considered for chronic conditions, such as diabetes and cardiovascular diseases, offering a potential genomics-based method of treatment.
- Gene Editing Applications: The advancement toward pairing AAV delivery with CRISPR technology opens a plethora of avenues for correcting genetic disorders at the molecular level. This synergy could redefine treatment protocols and lead to novel therapies.
The expansion of these therapeutic applications not only helps in addressing a wide range of diseases but could also lead to groundbreaking advancements in our approach to healthcare.
Global Collaboration and Funding
For the future of AAV research to be realized, global collaboration and funding are indispensable. Investing in multidisciplinary partnerships can facilitate the sharing of knowledge and resources that are critical for breakthroughs.
- International Research Networks: Establishing connections between universities, biotech companies, and research institutions globally fosters an environment of innovative collaboration.
- Public and Private Partnerships: To drive large-scale clinical trials and product development, synergizing public initiatives with private investments can foster an environment that nurtures discovery.
- Grant Programs and Funding Initiatives: Increasing funding opportunities specifically targeting AAV research will enable more teams to engage in pioneering studies and expedite the overall progress in this field.
The journey ahead for AAV research, enriched by collaboration and ample funding, holds the potential to transform our understanding and application of gene therapy dramatically. By addressing these critical directions, the hope is to set the stage for innovative breakthroughs that could redefine the future of medicine.
Finale
The conclusion serves as a pivotal aspect of this article, encapsulating the key learnings and broader implications of AAV models within gene therapy. In this fast-evolving field, drawing coherent insights from the previous sections empowers researchers, students, and professionals alike to track developments and crucial findings.
Summarizing Key Points
In retracing our steps through the complexities of AAV models, we can underscore several predominant themes:
- ** Mechanisms of AAV Vectors**: We explored the unique structure and replication cycle of AAVs, pinpointing their effectiveness in transducing target cells. This understanding is crucial for researchers aiming to optimize gene delivery systems.
- Applications in Gene Therapy: AAVs have shown promise in combating genetic disorders, neurodegenerative diseases, and even certain cancers. The real-world implications of these applications cannot be overstated, as they demonstrate transformative potential in treatments previously thought impossible.
- Advantages and Challenges: While the AAV's safety profile and therapeutic efficacy are significant benefits, impressive advances do not come without challenges, such as immunogenic responses and limited packaging capacity. Acknowledging these hurdles offers a roadmap for future innovations in AAV research.
- Ethical Considerations: It's vital to remain cognizant of the ethical implications while advancing AAV gene therapy, ensuring informed consent and equitability in access to therapies being developed.
Implications for Future Research
Looking forward, the future of AAV research offers extensive possibilities. It's clear that the pathway is not solely about amplifying existing techniques but also about exploring novel approaches. Here are some anticipated directions for research:
- Vector Engineering: Enhancing the delivery efficiency and specificity of AAV vectors through advanced engineering techniques could revolutionize treatment protocols.
- Expanding Applications: Newer therapeutic targets will likely emerge as understanding of AAV capabilities continues to deepen, potentially extending into previously untouched areas of medicine.
- Global Collaboration: As with many scientific endeavors, fostering international collaboration and securing funding will be key to overcoming barriers. Sharing knowledge across borders can accelerate progress.