Understanding DNA Mutations: Their Nature and Impact
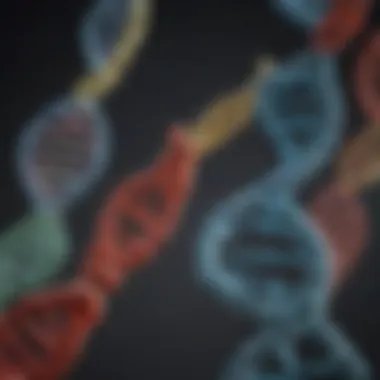
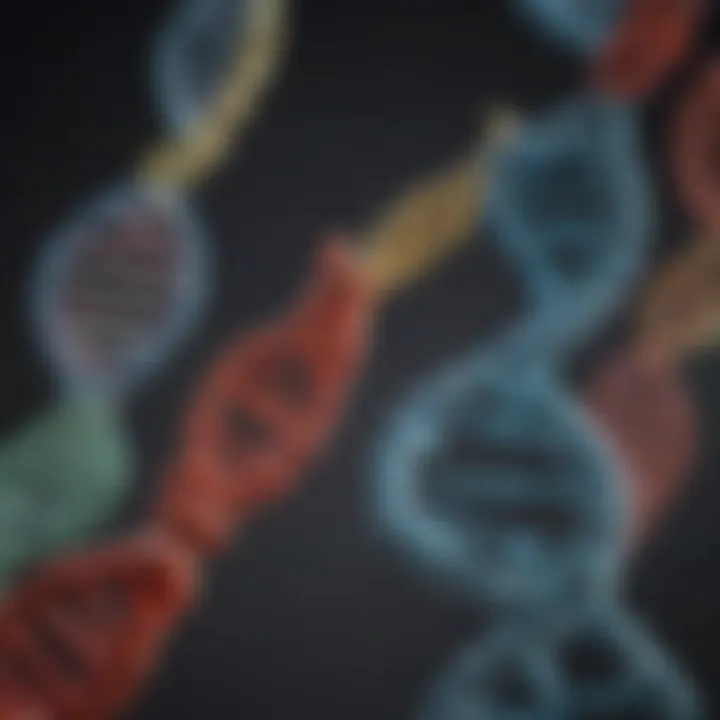
Intro
Mutations in DNA are fundamental to the study of genetics and the mechanisms of evolution. They represent changes in the genetic sequence and can occur for various reasons, from environmental factors to errors during DNA replication. Understanding these changes is crucial for many fields, including medicine, biology, and evolutionary studies. This article will comprehensively explore what mutations are, their types, causes, and the significant roles they play in health, disease, and the evolution of species.
Research Overview
Summary of Key Findings
Mutations can lead to a range of outcomes, from benign variations that contribute to genetic diversity to harmful alterations that may result in diseases like cancer. The research shows that not all mutations are created equal; some are silent while others can drastically change the function of a protein.
"Mutations are the raw materials for evolution, driving the diversity of life we observe today."
Background and Context
Historically, the study of mutations was limited to laboratory work. However, advances in genomic technologies have opened new avenues for exploration. Researchers now analyze mutation patterns and their implications not only in laboratory settings but also in wild populations. Much of our understanding stems from both classical genetics and modern molecular biology.
Methodology
Experimental Design
Understanding mutations requires a multi-faceted approach. Researchers utilize both observational and experimental designs to gather data on mutations.
Data Collection Techniques
Data collection for mutation studies often involves:
- Sequencing: Identifying the sequence of nucleotides in DNA to pinpoint mutations.
- Comparative analysis: Examining genetic material from different species to study evolutionary changes.
- Bioinformatics: Using software tools to analyze large genomic datasets and identify patterns in mutations.
The interplay of data collection methods leads to a rich dataset that informs our understanding of mutations in various contexts.
Prelude to DNA and Mutations
The interplay between DNA and mutations is fundamental to the study of genetics and biology. Understanding how mutations occur and their significance is not just an academic exercise; it informs numerous fields, from evolutionary biology to medicine. The examination of DNA, which is the blueprint of life, reveals intricate mechanisms that govern cellular functions. This section serves as a foundation, guiding the reader through the core aspects of DNA structure and the definition of mutations, setting the stage for subsequent discussions on their types and implications.
Basic Structure of DNA
DNA, or deoxyribonucleic acid, is a double-stranded molecule composed of nucleotides. Each nucleotide consists of a sugar, phosphate group, and a nitrogenous base. There are four types of nitrogenous bases in DNA: adenine, thymine, cytosine, and guanine. The specific sequence of these bases encodes genetic information.
The double helix structure of DNA, first described by James Watson and Francis Crick in 1953, allows for efficient storage of genetic data. The strands are held together by hydrogen bonds between complementary base pairs—adenine pairs with thymine, and cytosine pairs with guanine. This arrangement is crucial for replication, where the strands separate and serve as templates for creating new strands.
In summary, the basic structure of DNA underpins its function. Any alteration within this structure can lead to mutation, which can affect organismal traits and functions.
Definition of Mutations
Mutations are defined as permanent alterations in the DNA sequence that make up a gene. These modifications can arise through various mechanisms, such as errors during DNA replication, environmental factors, or even as a result of cellular processes. Mutations can occur in different forms, affecting a single nucleotide or larger segments of DNA.
They may be classified based on their impact:
- Beneficial mutations contribute positively to an organism’s fitness, potentially providing an advantage in changing environments.
- Neutral mutations have no noticeable effect on an organism, neither enhancing nor hindering survival.
- Harmful mutations can disrupt normal functioning, leading to diseases or disorders.
It is essential to recognize that while mutations are often viewed negatively, they play a crucial role in the evolution of species. Genetic diversity, driven by mutations, allows populations to adapt over time. Understanding the nuances of mutations aids in comprehending broader biological processes, influencing areas from medicine to ecology.
"Mutations are not just errors; they are vehicles of evolution, fueling the tapestry of life through diversity."
Types of DNA Mutations
Understanding the different types of mutations in DNA is crucial for several reasons. Firstly, they play a key role in genetic diversity, which is vital for evolution and adaptation. Secondly, knowing the types helps in understanding how certain mutations can lead to diseases. Each type has unique characteristics and implications, adding layers to our knowledge of genetics.
Point Mutations
Point mutations involve a change in a single nucleotide. This subtle alteration can have varying significance within the genetic code.
Missense Mutations
Missense mutations are a specific type of point mutation where one amino acid is replaced by another in a protein. This can lead to changes in protein function, which is critical for numerous biological processes. The key characteristic of missense mutations is that they can be either harmless or detrimental, depending on how the alteration affects protein activity.
They are beneficial to study because they reveal how small changes can lead to different phenotypes. A unique feature of missense mutations is their ability to confer new functions or properties to proteins, thus being a significant source of variability among species. However, some missense mutations can disrupt essential functions, leading to diseases.
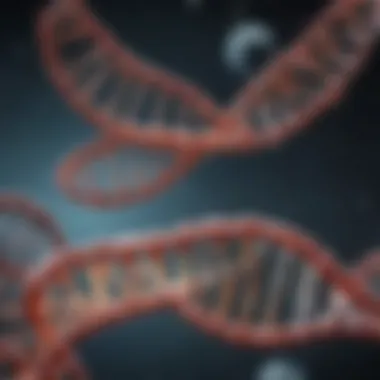
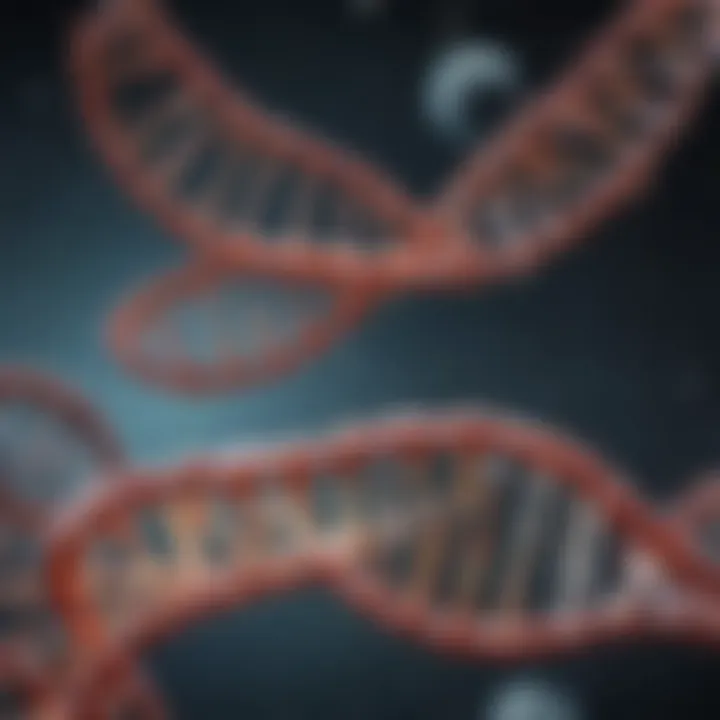
Nonsense Mutations
Nonsense mutations introduce a premature stop codon in the sequence, resulting in a truncated protein. This is significant as it often leads to nonfunctional proteins, severely impacting organismal health. The key aspect of nonsense mutations is their high potential to cause genetic disorders.
They are notably impactful for this article since they exhibit how drastic changes occur from a single nucleotide alteration. Their unique feature lies in their ability to cause loss of function in proteins. The disadvantage is clear; such mutations are frequently associated with severe genetic conditions.
Silent Mutations
Silent mutations are intriguing because they do not change the amino acid sequence of a protein. Despite this, they can have functional implications, such as affecting protein folding or stability. The key characteristic is their ability to silently exist without manifesting changes in the phenotype. This makes them an interesting choice for study.
Their unique feature is their prevalence in the genetic code without apparent consequences. However, some silent mutations may still impact gene expression or regulation, presenting both advantages (for genetic stability) and disadvantages (potential unrecognized effects).
Insertions and Deletions
Insertions and deletions are mutations that add or remove nucleotides, respectively. These changes can have dramatic effects on the reading frame of the genetic code.
Frameshift Mutations
Frameshift mutations result from insertions or deletions that are not in multiples of three nucleotides. This disrupts the entire downstream reading frame, altering the protein translation process. The importance of frameshift mutations lies in their potential to yield entirely different proteins.
These mutations are popular in discussions about genetic disorders due to their often catastrophic effects on proteins. A unique feature of frameshift mutations is their widespread occurrence in various genetic diseases. The disadvantage is that they frequently lead to nonfunctional proteins, significantly affecting organismal viability.
In-frame Mutations
In-frame mutations involve the insertion or deletion of nucleotides in multiples of three. This type maintains the original reading frame, resulting in the addition or loss of one or more amino acids without altering the rest. The contribution of in-frame mutations is notable because they can change protein properties while preserving overall structure.
Their key characteristic is that they can introduce variability without severe consequences. In-frame mutations are practical for illustrating how proteins can adapt through small modifications. However, their unique feature may still cause functional differences depending on the location of the insertion or deletion.
Large-scale Mutations
Large-scale mutations can affect long segments of DNA, having substantial consequences for organisms. Understanding these mutations helps elucidate genetic complexities and evolutionary processes.
Duplications
Duplications involve the copying of a segment of DNA, leading to extra genetic material. This is essential because it can create gene redundancy, potentially helping in evolution. The key characteristic is the potential for gene families to form, which can provide new functions over time.
Duplications are advantageous because they give genetic material a chance to evolve new functions while the original remains functional. Their unique aspect allows for adaptability in changing environments, but disadvantages include possible genomic imbalances.
Deletions
Deletions remove segments of DNA, which can disrupt gene function. Their importance is evident in many genetic disorders where critical genes are lost. The key characteristic of deletions is that they can lead to loss of essential functions.
Deletions are beneficial to study as they provide insight into how loss of genetic information can impact health. Their unique feature is their contribution to various genetic diseases. However, deletions can lead to significant setbacks in organism viability.
Translocations
Translocations occur when sections of DNA are relocated within the genome. This can result in new genetic combinations and is a key aspect in cancer development. The importance of translocations is underscored by their role in oncogenes, where they can activate or dysregulate critical genes.
Key characteristics include their potential to create fusion genes, which can lead to novel protein products. Translocations are vital in cancer biology for understanding tumorigenesis. Their unique aspect is their ability to restructure genetic information. However, they can lead to complexities in gene expression and potential health issues.
Mechanisms of Mutation
Understanding the mechanisms of mutation is crucial, as it connects the processes that lead to genetic variation and the evolution of species. Mutations can arise spontaneously or be induced by environmental factors. Every mutation adds to the complexity of how life adapts and evolves. This section will cover both of these aspects, along with the repair mechanisms that are essential to maintaining genetic integrity.
Spontaneous Mutations
Spontaneous mutations occur without any external influence. These mutations can arise from errors in DNA replication, spontaneous damage from internal cellular processes, or the natural chemical degradation of DNA.
One key characteristic of spontaneous mutations is their unpredictability. They can result in various forms of genetic change, from single nucleotide substitutions to larger structural variations. The random nature of these mutations provides a fertile ground for evolution, enabling organisms to adapt to changes in their environment over generations. Despite their potential contributions to evolution, spontaneous mutations can also lead to detrimental effects, such as genetic disorders.
Induced Mutations
Induced mutations are a result of external factors, which can be classified into two main categories: chemical agents and radiation. These factors significantly influence mutation rates and can drive genetic diversity.
Chemical Agents
Chemical agents, such as certain drugs or environmental toxins, can directly alter the DNA structure. These agents tend to cause changes that mimic natural processes but with a much higher frequency. Their contribution to mutations is significant and well-documented. The key characteristic of chemical agents is their ability to interact with DNA bases, leading to mispairing during replications.
One unique feature is that many chemical agents act as alkylating agents, attaching alkyl groups to DNA and causing changes in base pairing. This can lead to mutations that can be either harmful or benign. While chemical agents can be useful in genetic research to understand mutagenesis, they also pose risks to human health.
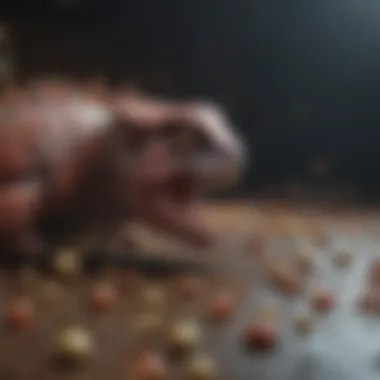
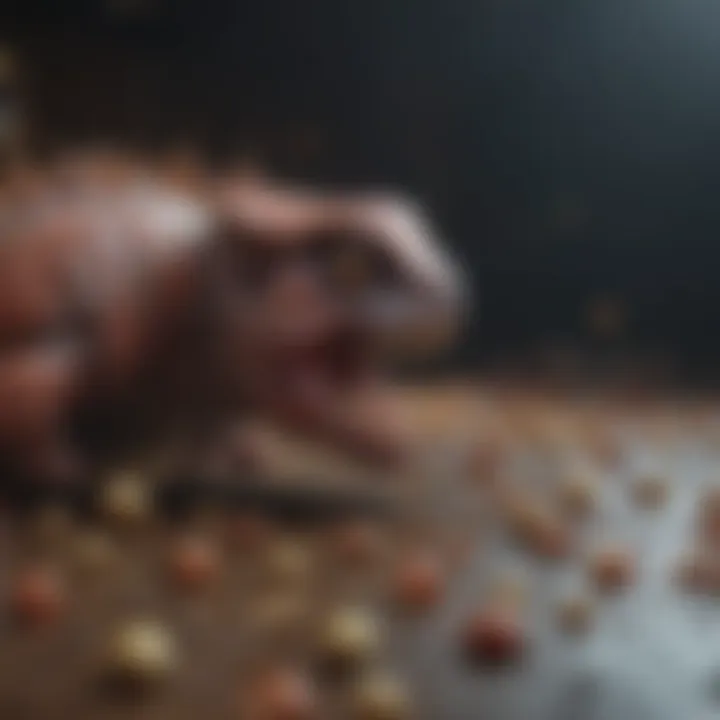
Radiation
Radiation, including ultraviolet (UV) and ionizing radiation, is another significant cause of induced mutations. The energy from radiation can break chemical bonds in DNA, leading to structural damage. A key characteristic of radiation-induced mutations is their ability to create thymine dimers, a common form of damage from UV light.
The unique feature of radiation is its capacity to cause double-strand breaks in the DNA backbone. This can lead to severe consequences, such as genomic instability. While radiation is a natural component of the environment, its excessive exposure can result in increased mutation rates, highlighting the delicate balance required in evolution.
DNA Repair Mechanisms
Even though mutations are a natural part of genetic changes, organisms have evolved complex DNA repair mechanisms to correct or minimize these alterations. Understanding these repair processes is important for grasping how cells maintain genomic stability.
Mismatch Repair
Mismatch repair (MMR) is a crucial mechanism for correcting errors that occur during DNA replication. In MMR, specialized proteins recognize and repair mismatched base pairs. The key characteristic of this mechanism is its ability to efficiently identify and correct errors, which prevents the accumulation of mutations.
One unique feature of MMR is its capability to differentiate between the newly synthesized strand and the template strand. This allows the system to correctly target mistakes for repair. The advantages of MMR become evident, as it significantly reduces the incidence of mutations that could lead to genetic disorders or contribute to cancer.
Nucleotide Excision Repair
Nucleotide excision repair (NER) is another fundamental DNA repair mechanism. NER is responsible for removing bulky DNA adducts, such as those caused by UV exposure. The key characteristic of NER is its ability to recognize and remove a range of DNA lesions, restoring normal DNA structure.
One of the essential features of NER is its multi-step repair process, involving several enzymes and proteins that coordinate to detect damage, excise the defective DNA segment, and fill the gap with the correct nucleotides. The advantages of NER are profound, as it can rectify a broad spectrum of DNA damage that arises from environmental insults, thus maintaining genomic integrity.
Conclusion: The mechanisms of mutation play a significant role in evolution and health. From spontaneous mutations to those induced by chemicals and radiation, each type makes a mark on the genetic landscape. Additionally, DNA repair mechanisms safeguard against these changes, underlining their importance in biological systems.
The Role of Mutations in Evolution
Mutations serve as a crucial mechanism for biological evolution. By altering the genetic code, they generate new alleles and lead to variations within a population. This is significant because genetic variation is the foundational element for evolutionary processes such as natural selection and adaptation. To grasp the role of mutations fully, one must consider both their immediate effects and their broader implications over time.
Mutations as a Source of Genetic Variation
Mutations introduce changes to the DNA sequence. These changes can manifest in various ways, including single nucleotide variations, insertions, or deletions. Each mutation provides new options for a population's genetic makeup.
- Organisms with advantageous mutations may have a better chance of surviving and reproducing.
- This leads to a gradual accumulation of beneficial traits in successive generations.
For example, if a mutation occurs that allows an organism to better utilize resources in its environment, that organism is likely to thrive compared to those without such mutation. Thus, this newfound genetic diversity is essential for the adaptability of species.
Natural Selection and Adaptation
Natural selection acts on the variations created by mutations. The environment exerts selective pressures that favor certain traits over others. In essence, the environment is the yardstick against which a mutation’s utility is measured.
Key points regarding natural selection and adaptation include:
- Traits that enhance fitness become more common in the population.
- Conversely, detrimental mutations can lead to reduced survival or reproductive success, leading to their eventual elimination from the gene pool.
Thus, over time, natural selection shapes the evolutionary trajectory of species. Mutations are not random events; they catalyze changes that can drive significant evolutionary shifts.
"Without mutations, evolution would be stagnant, lacking the essential variability necessary for the adaptation and survival of species in ever-changing environments."
To summarize, mutations are fundamental to evolution. They create the genetic diversity needed for natural selection to function effectively. As a result, they underscore the dynamic nature of life on Earth.
Mutations and Human Health
Understanding the connection between mutations and human health is crucial for recognizing how genetic variations can lead to significant health issues. Mutations can disrupt normal biological processes, leading to conditions that can affect various systems in the body. This section will explore specific examples of genetic disorders and cancer, illustrating the practical implications of mutations on human health.
Genetic Disorders
Cystic Fibrosis
Cystic Fibrosis is a genetic disorder caused by mutations in the CFTR gene. This gene is responsible for coding a protein that regulates the movement of salt and water in and out of cells. The most common mutation, known as F508del, leads to the production of a misfolded protein that does not function properly.
The key characteristic of Cystic Fibrosis is the accumulation of thick mucus in various organs, particularly the lungs and pancreas. This results in difficulties in breathing and digestive issues. Cystic Fibrosis is a well-known example in discussions about genetic disorders. It illustrates how a single mutation can have widespread effects on health. The unique feature of this disorder is its impact on both respiratory and gastrointestinal systems, making it a significant focus for medical research and treatment development.
Advantages/Disadvantages
The advantage of studying Cystic Fibrosis lies in the advances in treatments, such as CFTR modulators, that have improved quality of life for many patients. However, it also highlights the limitations in gene therapy and the challenges in providing effective treatments for all affected individuals.
Sickle Cell Anemia
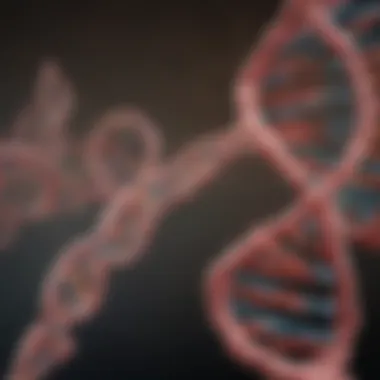
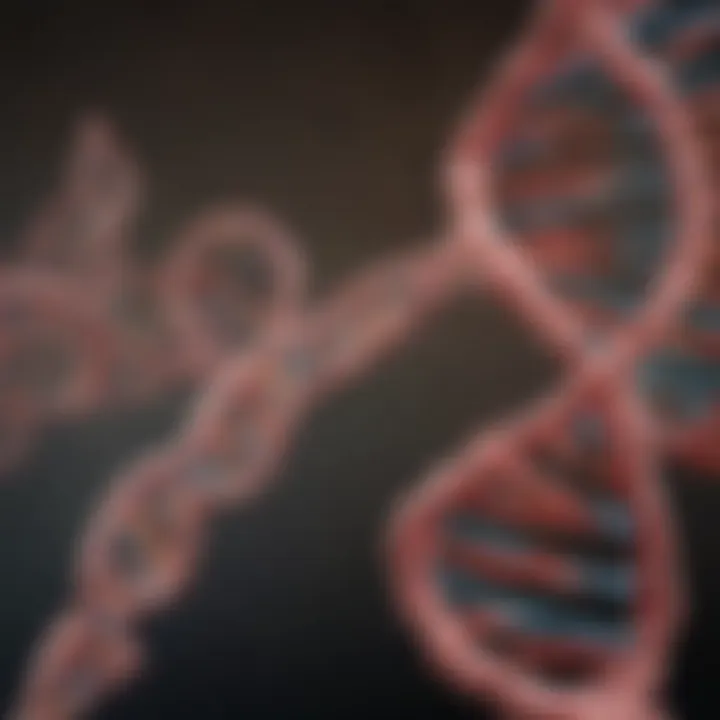
Sickle Cell Anemia is another genetic disorder caused by a single point mutation in the HBB gene. This mutation results in the production of abnormal hemoglobin, leading red blood cells to form a sickle shape. This distorted shape causes blockages in small blood vessels, leading to pain and potential organ damage.
The key characteristic of Sickle Cell Anemia is its impact on oxygen delivery throughout the body. This disorder is chosen for discussion due to its significant implications for public health, particularly in populations of African descent. The unique feature of Sickle Cell Anemia is its role in providing a survival advantage against malaria, a factor that has shaped its prevalence in certain regions.
Advantages/Disadvantages
Understanding Sickle Cell Anemia has led to beneficial treatments, including blood transfusions and hydroxyurea, which can reduce symptoms. However, it also underscores the socio-economic challenges faced by patients in accessing care and the ongoing need for research into curative therapies.
Cancer and Mutations
Oncogenes
Oncogenes are mutated forms of normal genes (proto-oncogenes) that promote cell division. Mutations can lead to the overactivation of these genes, resulting in uncontrolled cell growth, a hallmark of cancer. The study of oncogenes is vital for understanding cancer development.
A key characteristic of oncogenes is their ability to drive the proliferation of tumors. They are an essential part of cancer biology discussions, given that many targeted therapies developed focus on inhibiting the action of these genes. The unique feature of oncogenes is that they can be activated by various factors, including chemical exposure and radiation, making them a significant focus in cancer research.
Advantages/Disadvantages
The advantage of studying oncogenes is the development of targeted therapies like trastuzumab for breast cancer. However, the challenge remains in understanding the complexity of tumor evolution and resistance mechanisms.
Tumor Suppressor Genes
Tumor suppressor genes play a critical role in regulating cell growth and preventing tumor formation. When mutations occur in these genes, such as the TP53 gene, the normal control over cell division is lost, increasing cancer risk.
A key characteristic of tumor suppressor genes is their protective function against cancer. This makes them a vital topic in health discussions related to cancer prevention and treatment strategies. The unique feature is their mechanism of action, which often involves DNA repair and apoptosis regulation.
Advantages/Disadvantages
Studying tumor suppressor genes has led to significant progress in understanding cancer biology, emphasizing prevention strategies. However, the disadvantage lies in the hereditary nature of some mutations, as seen in families with a history of certain cancers, which raises ethical considerations for gene testing and screening.
Conclusion: Mutations in DNA, whether in the form of genetic disorders or cancer-related changes, exhibit profound effects on human health. Understanding these processes offers both hope and challenges, emphasizing the importance of continued research.
Research and Future Directions
The study of mutations in DNA is essential for advancing our understanding of genetics and biology. This section focuses on two crucial areas: genomics and mutation studies, and the revolutionary CRISPR and gene editing technologies. Each of these areas plays a significant role in how we view genetic mutations and their implications.
Genomics and Mutation Studies
Genomics involves the comprehensive analysis of genomes, the complete set of genetic material within an organism. In the context of mutation studies, genomics provides a framework to identify and categorize mutations at an unprecedented scale. Through high-throughput sequencing technologies, researchers can now examine entire genomes, detecting mutations that may not be visible through traditional methods.
Understanding the patterns of mutations helps in various fields, including evolutionary biology and medical genetics. By mapping how mutations occur across different species, scientists gain insights into evolutionary processes. They can also associate specific mutations with diseases, leading to the discovery of genetic markers that can predict disease susceptibility.
Some key aspects of genomics in mutation studies include:
- Data Mining: Utilizing large datasets from genomic studies to identify recurring mutations that could play a role in diseases.
- Population Genetics: Analyzing how mutations vary within and between populations to understand human history and provide insights into inherited conditions.
- Mutational Signatures: Characterizing the unique patterns of mutations produced by environmental factors or genetic predispositions.
CRISPR and Gene Editing Technologies
The advent of CRISPR technology has profoundly changed the landscape of genetic research. CRISPR, short for Clustered Regularly Interspaced Short Palindromic Repeats, allows for precise editing of the genome. This capability to modify DNA sequences brings a dual advantage: understanding mutations and potentially correcting deleterious mutations.
Researchers use CRISPR not only to explore the function of specific mutations but also to develop therapies for genetic disorders. The ability to knock out or edit genes responsible for diseases showcases the potential of CRISPR in clinical applications. However, this technology also raises important ethical and safety considerations regarding its use in human genetics.
Key points of CRISPR and gene editing technologies include:
- Targeted Gene Editing: CRISPR's tailored approach lets scientists alter specific genes related to conditions such as cystic fibrosis or sickle cell anemia.
- Ethical Frameworks: The rapid developments in gene editing require robust ethical guidelines to manage the implications of germline modifications.
- Future Applications: Potential uses cover a wide range, from agricultural improvements to advanced therapeutics for genetic diseases and cancers.
The integration of genomics and CRISPR offers endless possibilities. As scientists delve deeper into DNA mutations, they pave the way for transformative changes in medicine, agriculture, and beyond.
Closure
In this section, we will consolidate the various aspects discussed about mutations in DNA. Understanding mutations is vital because they are fundamental to the diversity of life. Their role in genetics is multifaceted, influencing evolution, health, and even biotechnology. Mutations can lead to significant changes in organisms, giving rise to new traits that can enhance survival or adaptability.
Moreover, they are essential in the context of genetic disorders and cancers. By grasping the nature and dynamics of mutations, scientists can develop better diagnostic tools and therapies, ultimately benefiting human health. This underscores the need for continued research into DNA mutations and their implications.
Summary of Key Points
- Definition and Types: Mutations are alterations in the DNA sequence. They can be classified into several types, including point mutations, insertions, deletions, and large-scale mutations.
- Mechanisms: Mutations can occur spontaneously or be induced by environmental factors such as radiation or chemical agents.
- Evolutionary Role: They serve as a source of genetic variation, fueling the process of natural selection and adaptation.
- Health Impact: Many genetic disorders and cancers arise from specific mutations, thus highlighting the importance of genetic research in promoting health.
- Technological Advances: Cutting-edge technologies like CRISPR have revolutionized how mutations can be manipulated, opening new pathways in treatment and genetic engineering.
Implications for Science and Society
The understanding of DNA mutations transcends basic science and delves deeply into societal implications. In health care, recognizing how mutations contribute to diseases leads to better treatments and preventive strategies. For example, identifying mutations in oncogenes can inform cancer therapies.
Furthermore, the ethical considerations surrounding gene editing technologies, like CRISPR, raise critical questions about how far humanity should go in modifying life. The conversations around these topics must be informed by scientific understanding, ensuring that society balances innovation and ethical responsibility.
Overall, the study of mutations provides foundational knowledge for various fields including genetics, medicine, evolutionary biology, and biotechnology. As research evolves, so too will our understanding, encouraging ongoing dialogue among scientists, ethicists, and the public.