Understanding Mitochondria Isolation Buffers in Research
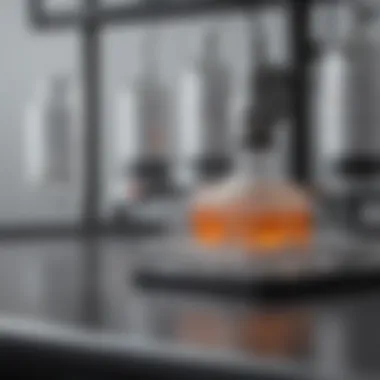
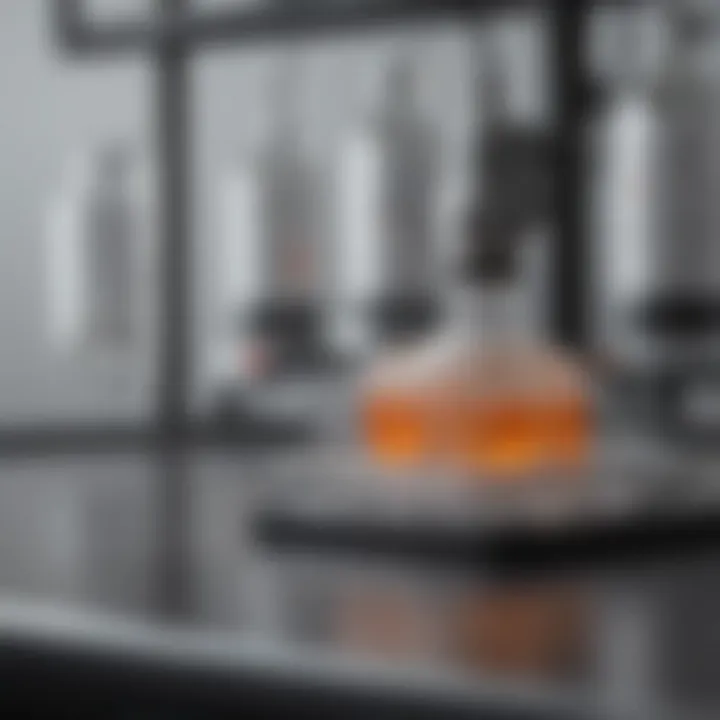
Intro
The significance of mitochondria in cellular function cannot be overstated; they are often referred to as the powerhouses of the cell. Understanding how to isolate these organelles is crucial for various biological and biomedical studies. The isolation process allows researchers to examine mitochondrial functions in detail, assess their role in metabolism, and investigate how they contribute to overall cell health.
Mitochondria isolation buffers provide the means to isolate these structures effectively while preserving their function. This article delves into the composition, preparation, and application of mitochondria isolation buffers. It is aimed at students, educators, researchers, and professionals in the field of cellular biology. A comprehensive understanding of these buffers is an essential skill for anyone looking to advance their work in this area.
By focusing on essential topics such as buffer formulations, preparation techniques, and potential challenges in the isolation process, this guide aims to equip the reader with detailed knowledge. The narrative will also consider quality control measures, ensuring that isolated mitochondria are viable for subsequent research. As mitochondria continue to play a pivotal role in cellular metabolism and pathology, understanding their isolation will be a significant advantage for those engaged in biological research.
The methodologies used for mitochondria isolation will be examined in depth, alongside background information that provides context for the significance of each aspect. A thorough overview will set the stage for discussing practical applications, challenges faced during the isolation process, and future directions in the field.
Preamble to Mitochondria Isolation
Mitochondria are fundamental organelles found in most eukaryotic cells. Their isolation is critical for various types of biological research, including studies on cellular respiration, energy production, and metabolic disorders. This section underlines why the proper isolation of mitochondria is essential not only for understanding cellular functions but also for exploring potential therapeutic interventions.
Mitochondria isolation allows for precise analysis of these organelles. Isolated mitochondria provide a medium for investigating their enzymes, genome, and other components in a controlled environment. This makes them indispensable for experiments aimed at understanding how disturbances in mitochondrial functions can lead to diseases such as diabetes, neurodegenerative disorders, and certain cancers.
In summary, understanding mitochondria isolation is crucial. It informs researchers about the operational dynamics and health of cells, embedding its significance in cellular biology.
Defining Mitochondria and Their Role in Cells
Mitochondria are often referred to as the powerhouses of the cell. They generate adenosine triphosphate (ATP), the main energy currency in cellular processes. Their structure is intricate, consisting of an outer membrane, inner membrane, intermembrane space, and matrix. Each section plays a role in metabolic activities.
In addition to energy production, mitochondria are involved in several other cellular processes. They regulate the apoptosis pathway, influence the calcium ion balance, and contribute to the generation of reactive oxygen species. Their participation in these functions makes them vital not only for energy metabolism but also for maintaining cellular homeostasis.
Importance of Mitochondria in Cellular Metabolism
Mitochondria are pivotal in metabolic pathways, primarily through oxidative phosphorylation, where they convert biochemical energy from nutrients into ATP. Their role extends to catabolic and anabolic processes, making them central to both energy release and biosynthesis of macromolecules. Understanding this dual role is essential for interpreting how cells generate and utilize energy.
Their dysfunction can lead to metabolic syndrome and contribute to aging. Many chronic diseases have roots in mitochondrial impairment, highlighting the importance of examining mitochondrial function and structure through isolation protocols. Investigating isolated mitochondria helps establish clear connections between mitochondrial health and various metabolic states, providing a pathway for developing targeted treatments.
"Mitochondria are not just powerhouses; they are hubs of metabolic pathways essential for life."
Through proper isolation methods, researchers can explore how mitochondrial dysfunction relates to metabolic diseases, thus showcasing the broader implications of mitochondrial research for health and disease management.
Overview of Isolation Buffers
In biological research, isolation buffers play a critical role in the purification and study of mitochondria. The effectiveness of experiments often hinges on the quality of these buffers. Their formulation must be carefully considered to ensure maximal cell compartment isolation. Isolation buffers are tailored to maintain the viability of mitochondria while minimizing contamination from other cellular components. Therefore, understanding the overview of isolation buffers is vital for researchers working with mitochondria.
Definition and Purpose of Isolation Buffers
Isolation buffers are specialized solutions used to create an optimal environment for isolating specific cellular components. The primary purpose of these buffers is to facilitate the separation of mitochondria from other cellular debris and organelles. They achieve this by providing necessary ionic strength and pH levels while maintaining the overall integrity of the mitochondria.
A well-prepared isolation buffer can significantly enhance the yield and purity of isolated mitochondria. This can lead to more reliable experimental results in downstream applications, such as enzymatic assays and functional studies. By controlling the physiological conditions, researchers can ensure that isolated mitochondria retain their functional properties, offering insights into cellular metabolism and energy production.
Not only do isolation buffers support the physical separation of mitochondria, but they also play a role in stabilizing mitochondrial function during the isolation process. This is essential for understanding the specific roles of mitochondria in various biological contexts, including metabolic disorders and other disease states.
Types of Isolation Buffers
Several types of isolation buffers exist, with each formulated for different experimental goals. The choice of buffer may depend on factors such as the source of cells and the intended downstream applications. Here are some commonly used isolation buffers:
- Mitochondria Isolation Buffer (MIB): This buffer is typically used for isolating mitochondria from various tissues while maintaining their functional integrity.
- Sucrose-based Buffers: Sucrose gradients are often utilized for density gradient centrifugation, which separates mitochondria based on their buoyancy.
- HEPES-buffered Solutions: These solutions help maintain pH stability during mitochondrial isolation, ensuring the functionality of isolated organelles.
- Bio-specific Buffers: Customized buffers may include specific substrates or inhibitors that provide additional support for specific mitochondrial functions being studied.
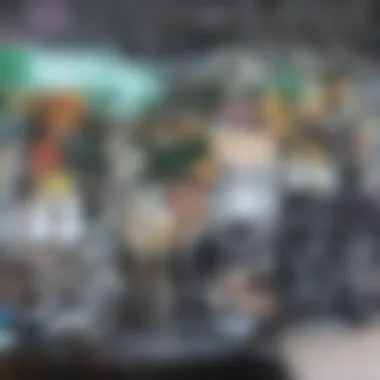
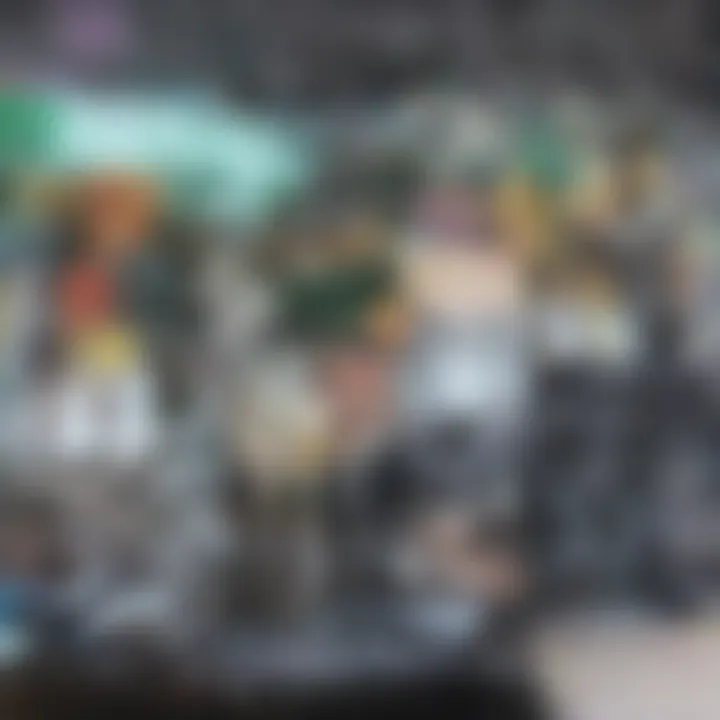
Choosing the right type of isolation buffer is essential for ensuring successful mitochondrial recovery. Researchers must consider the specific requirements of their experiments to select the most suitable formulation.
By understanding the different types and purposes of isolation buffers, researchers can enhance their experimental designs and derive quality insights from mitochondrial studies.
Components of Mitochondria Isolation Buffer
The components of a mitochondria isolation buffer are crucial for obtaining high-quality and functional mitochondrial preparations. These components influence the overall yield and integrity of the isolated mitochondria, ultimately impacting subsequent experimental outcomes. Understanding these elements not only aids in establishing effective methodologies but also ensures that research into mitochondrial functions remains reliable and precise.
Ionic Composition and Its Importance
The ionic composition of the isolation buffer plays a pivotal role in maintaining mitochondrial membrane potential and, consequently, their functionality. The primary ions found in these buffers usually include potassium, sodium, and magnesium.
- Potassium ions help in stabilizing the membrane potential, facilitating proper functioning of the inner mitochondrial membrane.
- Sodium ions contribute to maintaining osmotic balance and cellular homeostasis during the isolation process.
- Magnesium ions are essential for various enzymatic reactions that occur within the mitochondria, such as those involved in ATP synthesis.
The precise concentration of these ions should be optimized based on the cell type being used for isolation. If the ionic balance is not correctly implemented, it can lead to mitochondrial damage or loss of function, rendering the samples unfit for analysis. Researchers must pay careful attention to the composition of the buffer to ensure that it promotes the viability and functionality of the isolated mitochondria.
Common Additives and Their Functions
In addition to ionic components, various additives are incorporated into mitochondria isolation buffers to fine-tune their effectiveness. Understanding these additives and their functions is essential for achieving optimal results. Some common additives include:
- Bovine Serum Albumin (BSA): This protein stabilizes mitochondrial membranes and prevents aggregation during isolation.
- Sucrose: This sugar provides an isotonic environment, protecting mitochondria from osmotic stress.
- Protease Inhibitors: These compounds are critical for preventing protein degradation, which can occur during isolation.
Each additive serves a specific purpose that assists in preserving mitochondrial structure and function. By carefully selecting and combining these components, researchers can significantly enhance the quality of the isolated mitochondria.
In summary, both the ionic composition and common additives in mitochondria isolation buffers are instrumental in determining the viability and functionality of isolated mitochondria, thereby affecting subsequent analyses. The careful selection of these components ensures that the resulting mitochondrial preparations serve as reliable sources for understanding their biological roles.
Preparation of Mitochondria Isolation Buffer
The preparation of mitochondria isolation buffer holds significant importance in the context of biological research. A correctly formulated buffer can maximize the yield and purity of isolated mitochondria, which is crucial for subsequent experiments and analyses. This section offers insight into the fundamental procedures and considerations necessary for preparing an effective isolation buffer. By understanding the key aspects involved, researchers can enhance the reliability of their findings and improve the overall quality of their experiments.
Basic Protocol for Buffer Preparation
The basic protocol for buffer preparation typically involves selecting appropriate components that create a supportive environment for mitochondrial stability. A common buffer used is the REB (Respiration Buffer). Here is a basic preparation outline:
- Buffer Base: Start with a Tris-HCl buffer, which will help maintain the pH. Ideally, aim for a pH of around 7.4.
- Ionic Composition: The inclusion of potassium chloride (KCl) and sodium chloride (NaCl) is vital. This helps to maintain osmotic balance.
- Calcium and Magnesium Ions: Adding calcium chloride (CaCl₂) and magnesium sulfate (MgSO₄) supports mitochondrial function.
- Additives: Common additives like BSA (Bovine Serum Albumin) can be introduced to prevent protein aggregation.
The combination of these ingredients ensures that the buffer mimics the physiological environment of mitochondria. Follow this protocol with careful measurements to avoid errors that may compromise mitochondrial integrity.
Adjustments for Specific Cell Types
Not all cell types behave the same way, and recognizing this is crucial. Adjustments to the standard buffer can be beneficial based on the source of the mitochondria being isolated. Below are some considerations that should be taken into account:
- Cell Type: Different tissues may require distinctive ionic strengths or pH levels. For instance, hepatic cells often tolerate a higher concentration of potassium.
- Specific Additives: Adding unique components may reflect the specific requirements of the target mitochondria. For example, brain mitochondria may benefit from supplements like cytochrome c.
- Optimization Procedures: Testing different buffer components through trial-and-error can help tailor the buffer to enhance mitochondrial recovery from specific cells.
Understanding how to adjust the buffer based on the cell type ensures high-quality mitochondria isolation. Each of these factors contributes to the efficiency of extraction and ultimately influences the quality of research output.
"A well-prepared mitochondria isolation buffer can provide the necessary support for effective mitochondrial function during experiments."
Methods of Mitochondria Isolation
Understanding the methods of mitochondria isolation is crucial for researchers aiming to explore mitochondrial function and its implications in various biological processes. The efficiency and quality of the isolation process directly influence subsequent experiments involving mitochondrial studies. Choosing the right method not only ensures good yield but also helps in maintaining the integrity of the isolated mitochondria. The two primary techniques discussed here are Differential Centrifugation and Vesicle/Density Gradient methods. Each method has specific advantages, drawbacks, and applications.
Differential Centrifugation Technique
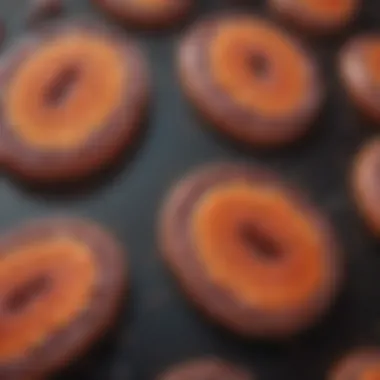
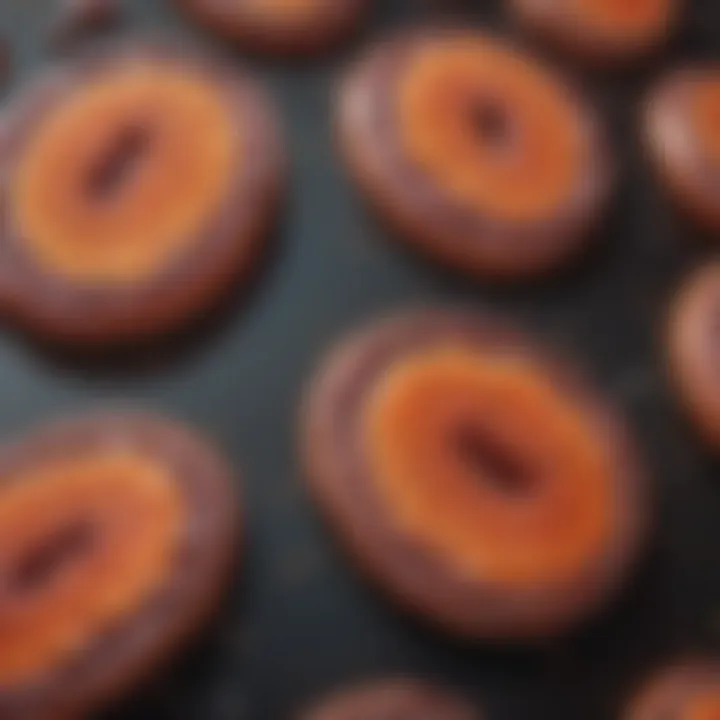
Differential centrifugation is one of the most common techniques used for mitochondria isolation. This method utilizes the principle of particle sedimentation based on size and density. The basic idea is to apply increasing centrifugal force in a series of spins, which helps separate cellular components based on their sedimentation rates.
The process begins with cell lysis to release cellular contents, followed by a low-speed centrifugation step. This initial spin removes large debris such as unbroken cells and nuclei. Subsequently, a high-speed centrifugation step isolates the mitochondria from the other organelles like lysosomes and endoplasmic reticulum. This method is praised for its simplicity and ability to yield a relatively pure mitochondrial fraction. However, it is important to monitor the speed and duration carefully since excessive spinning can damage the mitochondria.
Key considerations in this technique include:
- Temperature Control: Maintaining a low temperature during the isolation process is essential to preserve mitochondrial function.
- Buffer Selection: The isolation buffer must be optimized to keep mitochondria intact and functional post-isolation.
- Sedimentation Effects: Different cell types may require adjustments in centrifuge speed and time to achieve optimal results.
Vesicle and Density Gradient Methods
Vesicle and density gradient methods represent another approach for isolating mitochondria. These techniques utilize gradients of varying densities to facilitate a more effective separation. In this context, the vesicle method often involves the use of synthetic or biological vesicles that encapsulate mitochondria, enabling separation through buoyancy.
The density gradient centrifugation uses materials like sucrose or Percoll to create a gradient in a centrifuge tube. When cellular homogenates are subjected to centrifugation, organelles, including mitochondria, migrate to their respective buoyancy densities. This method often results in higher purity levels of isolated mitochondria compared to standard differential centrifugation.
Some advantages of this method are:
- Increased Purity: The density gradients effectively separate mitochondria from other cellular components.
- Reduced Physical Stress: It applies less mechanical stress on organelles.
- Versatility: Useful for isolating mitochondria from various cell types, including specialized tissues.
However, it can be more time-consuming and technically challenging than differential centrifugation. Careful optimization of gradient conditions is essential to ensure optimal separation and yield.
"The choice of method can significantly impact the quality and functionality of isolated mitochondria, with each protocol presenting its own sets of benefits and challenges."
Understanding these techniques aids in advancing mitochondrial research, which has implications spanning from fundamental cellular biology to potential therapies for mitochondrial diseases.
Challenges in Mitochondria Isolation
Isolating mitochondria can be a complex process, fraught with challenges that researchers must navigate. Understanding these challenges is vital for anyone involved in cellular biology. The issues not only affect the quality of the isolated mitochondria but can also have significant implications for subsequent experiments.
Identifying common pitfalls can enhance the efficiency of isolation protocols. Moreover, recognizing the importance of quality control ensures reproducibility and reliability in research findings.
Common Pitfalls in Laboratory Protocols
The isolation of mitochondria often involves specific laboratory techniques that can easily go awry. Here are some of the most notable pitfalls:
- Incompatible buffer conditions: If the buffer used does not match the needs of the specific cell type, it can lead to suboptimal conditions for mitochondrial preservation.
- Improper centrifugation: Too high or too low centrifugation speeds can cause either damage to the mitochondria or incomplete isolation.
- Contamination: External factors such as environmental issues or cross-contamination with other cellular organelles can lead to misleading results.
- Temperature fluctuations: Mitochondria are sensitive to temperature variations. Failing to maintain consistent temperatures during isolation can impact their functionality.
Each of these aspects can skew the results of experiments aimed at understanding mitochondrial function, thus emphasizing the importance of meticulous protocol execution.
Quality Control Measures
Ensuring quality during mitochondria isolation involves implementing several control measures, which are essential for obtaining viable mitochondria samples. Such measures include:
- Standardized procedures: Utilizing a consistent protocol for isolation increases reproducibility across different experiments. This includes specifying buffer formulations and centrifugation settings.
- Regular calibration: Instruments used for centrifugation should be calibrated regularly to ensure accuracy in speed and temperature.
- Validation assays: Conduct functional assays post-isolation to confirm that the mitochondria remain intact and functional. This may involve measuring oxygen consumption or assessing respiratory chain activity.
- Documentation of all steps: Keeping detailed records of every isolation attempt can help identify potential issues in protocols that need adjusting, allowing for improvements over time.
Quality control is not just a good practice; it is a necessary component of reliable mitochondrial research.
Incorporating these quality control measures helps mitigate the risks associated with common pitfalls, leading to more reliable and meaningful results in mitochondrial studies. Researchers must pay attention to these challenges, as they directly influence the quality and applicability of the data gathered.
Applications of Isolated Mitochondria
Isolated mitochondria play a critical role in revealing the complexities of cellular metabolism and energy production. The study of these organelles helps scientists understand a range of biological processes, making their applications particularly significant in various research domains.
Investigating Mitochondrial Function
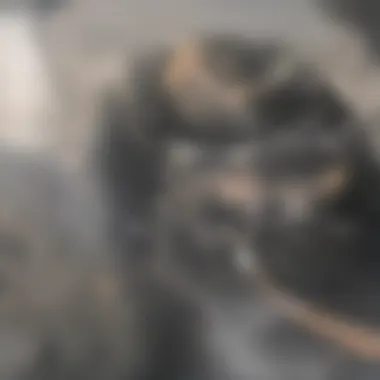
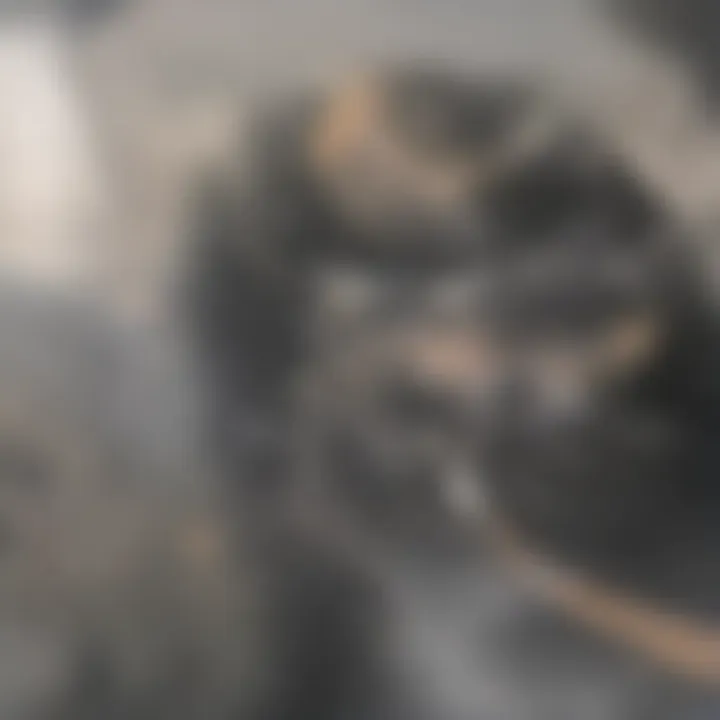
Understanding the specific functions of mitochondria is vital in cellular biology. Research often involves evaluating parameters such as respiration rates, substrate utilization, and reactive oxygen species generation.
Analyzing isolated mitochondria allows researchers to:
- Assess the efficiency of oxidative phosphorylation.
- Determine mitochondrial membrane potential changes.
- Investigate mitochondrial permeability transition, which is essential for understanding apoptosis.
This investigation can lead to new insights into disease mechanisms. For example, abnormal mitochondrial function is implicated in conditions such as diabetes and neurodegenerative diseases. Evaluating isolated mitochondria can therefore inform potential therapeutic strategies and intervention measures.
Disease Models and Therapeutic Exploration
Research into disease mechanisms often benefits from the application of isolated mitochondria. Using these organelles, scientists can model various diseases, including conditions like Alzheimer's, Parkinson's, and cardiac dysfunction.
This area of study can bring forward several advantages:
- Pathway Analysis: Isolated mitochondria facilitate the investigation of specific biochemical pathways affected by diseases. This helps in identifying targets for drugs.
- Drug Testing: Before clinical applications, potential therapeutics can be tested on isolated mitochondria to assess efficacy and toxicity.
- Personalized Medicine: Insights gained can be utilized in developing tailored treatment strategies based on mitochondrial function variations in individuals.
Mitochondrial dysfunction often leads to a cascade of downstream effects that worsen the clinical symptoms. Therefore, the ability to isolate mitochondria and investigate their role in disease could pave the way for innovative therapeutic approaches.
Ultimately, understanding mitochondrial applications is crucial for advancing our knowledge in cellular biology and improving future healthcare strategies.
Future Directions in Mitochondrial Research
Understanding the future of mitochondrial research is critical for advancing biomedicine and therapeutic approaches. As our knowledge of cellular metabolism and mitochondrial function deepens, the potential to discover novel treatments and personalized medicine options enhances. Mitochondria are more than energy producers; they play roles in apoptosis, oxidative stress, and disease pathology. Thus, focusing on emerging research directions can lead to significant breakthroughs in our comprehension and manipulation of these organelles.
Emerging Technologies and Techniques
Advancements in technology continually reshape the landscape of mitochondrial research. Several cutting-edge techniques are making a significant impact in this field:
- High-Throughput Sequencing: This technology has revolutionized genomics. In mitochondrial research, it allows for detailed analysis of mitochondrial DNA variations and the mutation landscape.
- CRISPR-Cas9 Gene Editing: With this tool, scientists can make precise edits to mitochondrial genes. This offers potential pathways to correct mutations that lead to mitochondrial diseases.
- Metabolomics: This approach focuses on the study of metabolic pathways. By analyzing metabolites, researchers can understand mitochondrial function and its role in various diseases, leading to better diagnostic tools.
- Fluorescent Microscopy: New imaging techniques enhance our ability to visualize mitochondrial dynamics in living cells. This helps measure changes in morphology and function in real-time, providing insight into mitochondrial behavior under physiological and pathological conditions.
The integration of these emerging technologies will likely propel mitochondrial research into new areas, unlocking efficient methods for studying mitochondrial diseases.
Potential for Personalized Medicine Applications
The link between mitochondria and personalized medicine is gaining traction. Mitochondrial dysfunction is implicated in numerous diseases, including metabolic disorders, neurodegenerative diseases, and cancer. By addressing how individual mitochondrial profiles correlate with health outcomes, tailored therapeutic strategies can be developed:
- Patient-Specific Treatments: Analyzing a patient’s mitochondrial DNA can inform individualized treatment plans, especially in conditions like mitochondrial myopathy or certain cancers.
- Pharmacogenomics: Understanding how drugs affect mitochondrial function can lead to personalized drug therapies that minimize side effects and enhance efficacy for different individuals based on their mitochondrial genotype.
- Predictive Health Monitoring: With advancements in biomarker discovery, mitochondrial metrics can serve as indicators for disease susceptibility, allowing early and targeted interventions.
"The future of mitochondrial research promises not just an enhanced understanding of cellular processes but an opportunity to revolutionize how we approach therapy in personalized medicine."
Culmination
The conclusion of this article emphasizes the critical role of mitochondria isolation buffers in biological research. These buffers are essential for preserving mitochondrial integrity and function during isolation and study. A thorough understanding of their composition and preparation is paramount for researchers aiming to explore mitochondrial biology.
Recap of Mitochondria Isolation Importance
Mitochondria are central to cellular energy metabolism. Their isolation enables the study of various physiological processes, including ATP production and apoptosis. By employing effective isolation buffers, researchers can ensure that isolated mitochondria maintain their functionality, which is crucial for accurate experimental results.
- Mitochondria isolation contributes significantly to understanding metabolic diseases.
- Proper buffers minimize damage to mitochondrial structures and functions.
- Reliable isolation protocols support reproducibility in experiments.
The ability to investigate mitochondrial action can lead to deeper insights, particularly in the context of disease mechanisms and potential therapies.
Final Thoughts on Mitochondrial Studies
This article illustrates the challenge and innovation involved in mitochondrial isolation. As methods and technologies evolve, new opportunities to delve beyond basic mitochondrial functions arise. Future research could unveil more about their roles in health and disease. Furthermore, personalized medicine initiatives may benefit from enhanced understanding of individual mitochondrial profiles.
Research in this domain is critical for elucidating mitochondrial dysfunctions in various conditions, such as neurodegenerative diseases and metabolic syndromes. Thus, continued exploration of mitochondria will undoubtedly pave the way for breakthroughs in both basic and applied biological research.
In summary, the pursuit of knowledge regarding mitochondria through effective isolation techniques is a frontier in science that holds the promise of transforming our understanding of cellular health.