Understanding RNA Sequence Templates and Their Impact
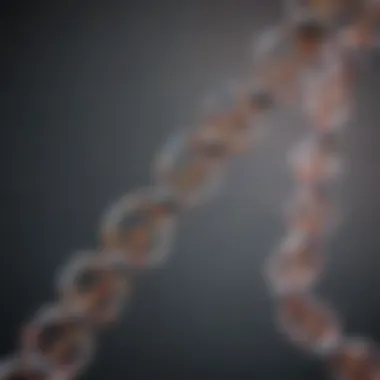
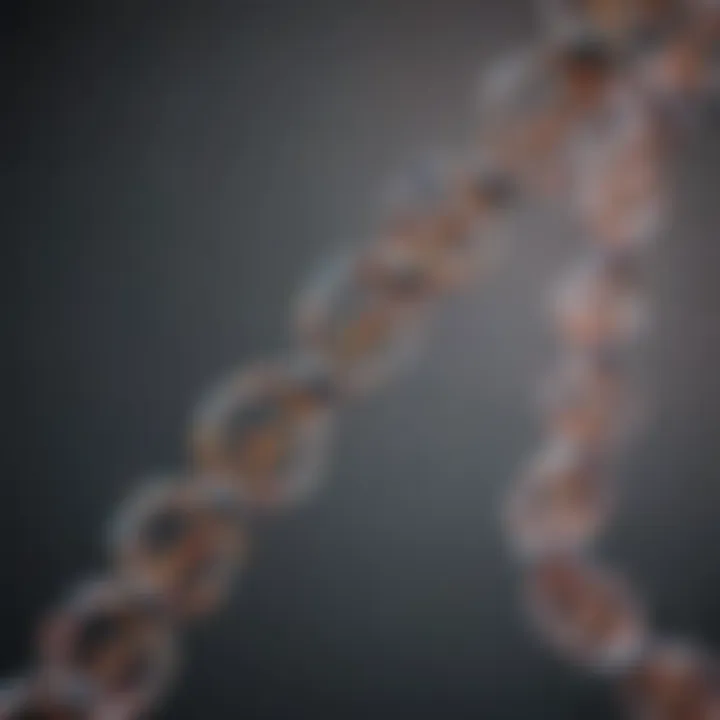
Intro
As we embark on this exploration, we’ll delve into how RNA sequences are formed, the importance of templates in their synthesis, and the methodologies employed in RNA sequencing. Moreover, comparisons with DNA templates will shed light on their unique and overlapping roles within cells. In today's scientific landscape, gaining insight into RNA templates not only enriches our understanding of molecular biology but also opens the door to innovative applications in biotechnology and medicine.
Research Overview
Summary of Key Findings
- RNA is composed of nucleotide sequences that serve as templates for various biological activities.
- Templates are essential for accurate RNA synthesis, influencing how genes are read and interpreted in the cell.
- Novel sequencing techniques have enhanced our ability to analyze RNA in diverse biological contexts, uncovering new roles and mechanisms.
Background and Context
The essence of RNA can often be overshadowed by its DNA counterpart, yet its structural and functional roles are indispensible. RNA, or ribonucleic acid, is typically single-stranded and forms various structures based on its sequences. Unlike DNA, which primarily serves as the repository of genetic instructions, RNA is more versatile. It can act as a template for protein synthesis, a regulator of gene expression, and a contributor to transmission of genetic information through various cellular pathways.
As research has progressed, we have come to recognize the complexities of RNA templates, particularly in the context of transcription and translation. These processes are crucial for cellular functions, and any aberration in RNA templating can lead to various diseases, including cancer and genetic disorders. By understanding these underlying principles, researchers can develop therapeutics aimed at correcting or altering RNA functions to treat or prevent diseases.
Implications in Scientific Research
- Disease Research: Insights gained from RNA sequencing can illuminate the underlying mechanisms of various diseases.
- Biotechnology Applications: RNA templates can serve as a basis for new diagnostic tools and therapeutic strategies.
- Synthetic Biology: Knowledge of RNA dynamics informs the design of novel organisms with specific traits or functions.
"The more we learn about RNA and its templates, the more we unlock the mysteries of life itself."
In grasping these themes, we can better appreciate the significance of RNA in both health and disease, cultivating a greater understanding that ripples through various domains of biology and medicine.
Intro to RNA
The study of RNA plays a pivotal role in modern biology and genetics. It serves as a bridge between the code locked in DNA and the proteins that execute various functions in living organisms. Understanding RNA is essential for grasping the complexities of cellular processes and the underlying mechanisms of genetic expression. With the advent of advanced sequencing technologies, the importance of RNA has surged, placing it at the forefront of genetic research and biotechnological innovations.
What makes RNA particularly significant is its ability to not only copy the genetic information but also to function independently in various biological roles. This versatility means that RNA sequences are not just mere templates; they are dynamic players in regulatory networks. By delving into RNA, this article aims to dissect its many forms and functions, ultimately revealing how RNA template sequences guide life at the molecular level.
Definition of RNA
At its core, RNA, or ribonucleic acid, is a single-stranded molecule essential for several biological roles including coding, decoding, regulation, and expression of genes. Unlike DNA, which carries the stable blueprint of an organism, RNA is often more transient and responds dynamically to the cellular environment.
The structural composition of RNA includes nucleotides, which consist of three primary components: a sugar (ribose), phosphate group, and one of four nitrogenous bases—adenine (A), cytosine (C), guanine (G), and uracil (U). The presence of uracil instead of thymine, found in DNA, is another key distinction that sets RNA apart and is crucial for its functionality.
In different cellular contexts, RNA takes various shapes that determine its roles, ranging from messenger RNA (mRNA) to transfer RNA (tRNA) and ribosomal RNA (rRNA).
Types of RNA
RNA isn't a one-size-fits-all molecule; it exists in several forms, each serving distinct purposes within the cell. The primary types of RNA include:
- Messenger RNA (mRNA): This type carries the genetic information from DNA to the ribosomes, where proteins are synthesized. It's like a script that the cellular machinery reads to create specific proteins needed for various functions.
- Transfer RNA (tRNA): The helper molecule, tRNA transports amino acids to the ribosome, ensuring that the protein is assembled in the right order according to the mRNA script. Without tRNA, proteins could not be formed accurately.
- Ribosomal RNA (rRNA): It forms a key part of the ribosome itself and plays a critical role in the process of translation, where proteins are assembled from amino acids. rRNA is crucial for binding the mRNA and tRNA together during protein synthesis.
- Small nuclear RNA (snRNA): These are involved in the processing of pre-mRNA in eukaryotes, particularly in splicing — removing non-coding sequences from mRNA.
- MicroRNA (miRNA) and Small interfering RNA (siRNA): Both are involved in gene regulation and silencing. They can bind to mRNA and prevent it from being translated into protein.
Each type of RNA contributes to the intricate signaling and regulatory networks that are crucial to cellular function and overall organism health. By exploring these varieties, we can begin to grasp how RNA sequence templates are essential in the wider realm of genetics and molecular biology.
RNA Sequence Basics
Understanding RNA sequence basics lays the groundwork for comprehending complex genetic functions and interactions. Our exploration of this topic starts with an appreciation of the components that make up an RNA sequence. By delving into these elements, we can better appreciate how they operate in concert within biological systems.
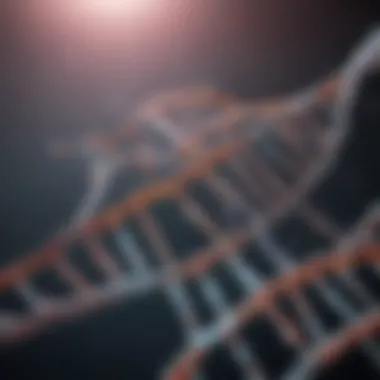
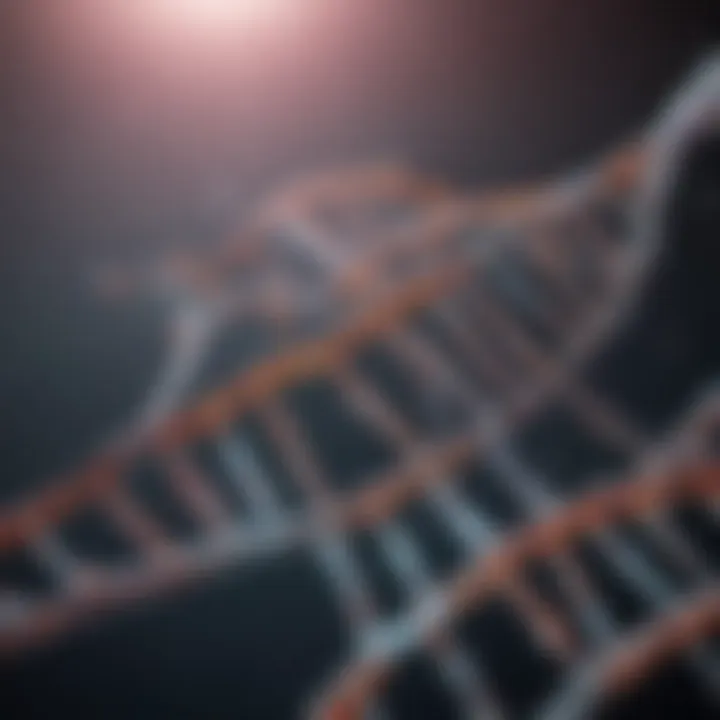
What Composes an RNA Sequence?
At its core, an RNA sequence consists of a linear chain of nucleotides, each of which plays a pivotal role in the synthesis of proteins, serving as the blueprints of cellular function. Nucleotides are comprised of three main components:
- A phosphate group – This component acts as the backbone of the RNA structure, linking together nucleotides through phosphodiester bonds.
- A ribose sugar – This sugar distinguishes RNA from its counterpart, DNA. The presence of an -OH (hydroxyl) group on the 2'-carbon of the ribose sugar makes RNA more reactive than DNA.
- Nitrogenous bases – These can be categorized into two groups:
- Pyrimidines (cytosine and uracil)
- Purines (adenine and guanine)
The sequence of these nitrogenous bases determines the genetic code, crucial for protein synthesis and gene regulation. It's the arrangement of these nucleotides that encodes specific information, influencing everything from cellular response to environmental changes to the overall functioning of organisms. The significance of an RNA's sequence cannot be overstated; even a slight alteration can lead to profound biological consequences.
Nucleotide Structures
The structural complexity of nucleotides significantly influences their functionality within RNA sequences. Each nucleotide’s structure supports its role in various biological processes, particularly during transcription and translation.
- Phosphate Group: The phosphate group connects nucleotides, creating a polymer chain. This connectivity allows RNA to maintain its structure while facilitating interaction with proteins and other molecules.
- Ribose Sugar: As mentioned earlier, ribose is a five-carbon sugar that carries both well-defined structure and specific reactivity. Its -OH group enables hydrogen bonding with other bases and molecules, fostering interactions essential for ribonucleic activity.
- Nitrogenous Bases: The building blocks are critical for base pairing; adenine pairs with uracil, while cytosine pairs with guanine. This selective pairing is vital for accuracy in protein synthesis, and any mismatch during transcription could lead to dysfunctional proteins.
Understanding the significance of nucleotide structure is key to grasping the intricate mechanisms of genetic expression.
Each element within the RNA sequence plays an interdependent role, echoing the synchronized dance of cellular machinery. By gaining insight into the foundations of RNA sequences, readers not only appreciate RNA's function but can also explore its broader implications in genetic regulation, biotechnology, and therapeutic approaches. This understanding sets the stage for intricate discussions about RNA templates and their roles in the life of an organism.
The Role of Templates in RNA
The importance of RNA templates cannot be overstated in the fields of genetics and molecular biology. These templates serve as the backbone for synthesizing RNA molecules, guiding the transcription process meticulously. As we dissect the role templates play, we uncover layers of complexity integral to understanding not only RNA as a molecule but also its broader impact in cellular mechanisms.
Template Definition
In the most straightforward terms, a template in RNA refers to a sequence of nucleotides that serves as a guide for the synthesis of complementary RNA strands. Essentially, during transcription, one strand of DNA is used as the template to create a corresponding RNA molecule. The relationship between the template DNA and the resultant RNA can be likened to that of a road map guiding a traveler through an unfamiliar territory.
To be a bit more technical, templates are instrumental in determining the specific sequence of bases in the RNA strand. This accuracy is not just a trivial detail; it's fundamental to the proper encoding of genetic information. If the template is flawed or the transcription process goes awry, the resulting RNA, and subsequently the protein it encodes, may not function as intended.
Importance of Templates in Transcription
Templates play a crucial role in the transcription mechanism of genes. Without a reliable template, the entire process of gene expression could falter. Here’s why their role is vital:
- Accuracy in Synthesis: The fidelity of RNA synthesis depends heavily on the template. Each nucleotide on the DNA is transcribed to the corresponding RNA nucleotide, ensuring that the genetic code remains intact.
- Regulatory Mechanisms: The template also opens pathways for the intricate regulation of gene expression. Certain sequences within the template can influence how actively a gene is expressed by allowing or blocking the binding of transcription factors.
- Biological Diversity: Variability in templates can lead to the formation of different RNA molecules from the same gene (like mRNA vs. tRNA). This increases the range of proteins that can be produced, significantly contributing to biological diversity and adaptability.
- Error Correction: Because templates direct the synthesis process, they also provide a means of error correction. Cellular mechanisms recognize mismatches during transcription, allowing for corrections that are critical for maintaining the integrity of genetic information.
"Templates are not just mere guides; they are the very blueprints of life, enabling the complex dance of transcription and expression."
RNA Sequencing Techniques
Understanding RNA sequencing techniques is pivotal for grasping the complexities of gene expression and regulation in various biological systems. RNA sequencing allows researchers to decipher the transcriptome—essentially the ensemble of RNA molecules present within a cell at any given time. This can yield insights not just into which genes are active, but also how they vary in different conditions. The field of RNA sequencing is ever-evolving, making it crucial for professionals in the field to stay updated on the latest methodologies and their implications.
Overview of Sequencing Methods
The core techniques in RNA sequencing have evolved substantially. Here are some major methods employed:
- Microarray Analysis: This older method allows for the simultaneous measurement of the expression levels of thousands of genes. While it has its benefits, like quick analysis of many genes, it is limited in terms of detecting low-abundance transcripts and relies on prior knowledge about the genes.
- Sanger Sequencing: While largely replaced by newer methods, Sanger sequencing can still paint a clear picture of specific RNA molecules. It’s precise but not suitable for high-throughput analysis.
- Next-Generation Sequencing (NGS): This has revolutionized RNA studies, allowing for massive parallel sequencing. It can sequence millions of RNA fragments simultaneously, making it a powerful tool for exploring complex transcriptomes.
Each method has its strengths and weaknesses, impacting the type of research one can conduct and the questions that can be answered. For instance, while microarrays are more accessible and cheaper, next-generation sequencing offers flexibility and depth of information.
Next-Generation Sequencing

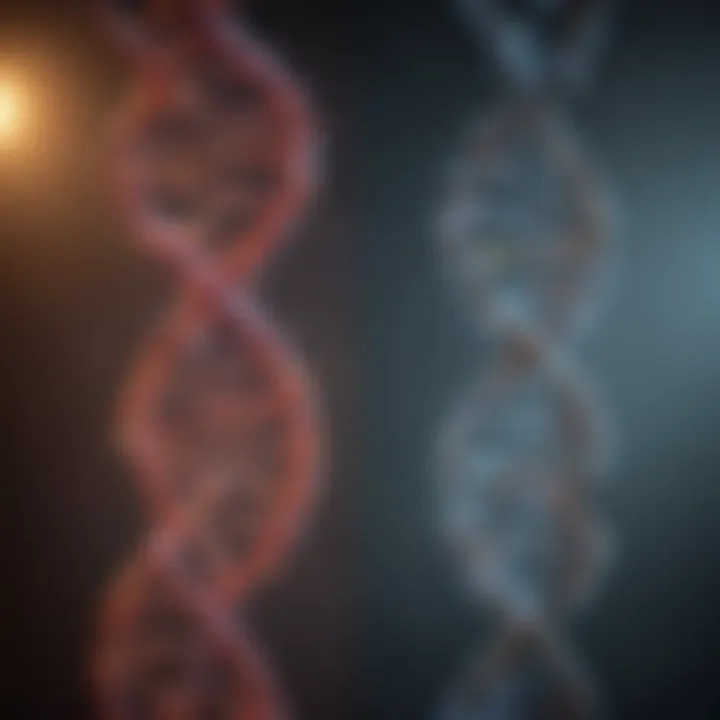
Next-generation sequencing (NGS) is the gold standard in RNA sequencing techniques today. Its ability to generate massive amounts of data at unprecedented speeds has transformed biomedical research.
Benefits of Next-Generation Sequencing:
- High Throughput: NGS can sequence millions of fragments in a single run, providing vast datasets for analysis.
- Sensitivity: It can identify rarer transcripts, which is crucial for studying diseases like cancer where mutations might occur in only a small number of cells.
- Versatility: Researchers can use NGS for many applications, including whole-transcriptome sequencing, targeted sequencing, and single-cell RNA sequencing.
Considerations and Challenges:
While NGS provides unparalleled insights, researchers must also consider potential pitfalls:
- Data Management: The sheer volume of data generated can be overwhelming. Efficient data storage, processing, and analysis are paramount.
- Cost: Although the costs have been decreasing, running an NGS platform can still be expensive, particularly when accounting for consumables and analysis software.
- Bioinformatics: The requirement for sophisticated computational tools and expertise is another layer of complexity that may deter some researchers.
In essence, while RNA sequencing techniques—particularly NGS—hold great promise, they also demand rigorous planning and understanding to ensure that they yield the most informative results. This balance between cutting-edge technology and methodical analysis is where the future of RNA research lies.
"RNA sequencing is not just about sequencing anymore; it’s a multi-faceted journey into understanding life at the molecular level."
Those in the field of genetics, genomics, or biotechnology should familiarize themselves with these techniques, as mastering them is key to pushing boundaries in understanding gene expression and its implications in health and disease.
Comparative Analysis of RNA and DNA Templates
The comparative analysis of RNA and DNA templates serves an essential role in understanding the molecular biology landscape. By dissecting the key structural and functional differences between these nucleic acid types, research can better illuminate how genetic information is processed and regulated within cellular environments. Recognizing the benefits and distinct characteristics of RNA versus DNA impacts many fields, from genetics and biochemistry to therapeutic design.
Structural Differences
To kick things off, let's dive into the structural variances that set RNA apart from DNA. The most glaring difference is the sugar component of their nucleotides. RNA contains ribose sugar, while DNA is made up of deoxyribose, which is essentially ribose minus one oxygen atom. This subtle change impacts stability significantly; RNA is more prone to hydrolysis because of that extra oxygen. So, RNA's relatively fragile structure makes it more suitable for short-lived functions within the cell, while DNA is the sturdy vault of genetic blueprints meant to endure through generations.
Another structural distinction is in the strands. RNA typically exists as a single-stranded molecule, allowing it to fold into various shapes. This flexibility enables it to perform diverse functions, such as catalyzing reactions or binding to other molecules. In contrast, DNA is usually double-stranded, forming a helical structure that not only protects its genetic information but also facilitates accurate replication.
"The differences in the structures of RNA and DNA link to their specific roles in genetic processes, affecting everything from stability to functionality."
The genetic code held within RNA is also notable, as it uses uracil in place of thymine, which is found in DNA. This switch not only simplifies the synthesis process but also reflects the functional dynamics of RNA in protein synthesis and regulation.
Functional Variations
Moving beyond structure, the functional implications of these differences are equally profound. RNA's versatility is on full display through its various types, including messenger RNA (mRNA), transfer RNA (tRNA), and ribosomal RNA (rRNA). Each type serves specific roles during protein synthesis. For instance, mRNA acts as a template that conveys genetic information from DNA to ribosomes, where proteins are synthesized.
On the flip side, DNA primarily serves as a long-term data storage system. It is the blueprint from which RNA is transcribed, but the functionality doesn’t end there. DNA's double helix structure is not just about storage; it also allows for accurate replication during cell division—a key process in growth and repair. However, when it comes to gene regulation, RNA steps in once again. The interaction between RNA and DNA can affect the expression of genes, allowing the cell to respond dynamically to environmental changes.
In summary, the structural and functional nuances of RNA and DNA reveal much about their roles in biology. While DNA’s stability ensures safe storage of genetic information, RNA’s flexibility and transient nature enable a broad range of biological activities, crucial for normal cellular function and adaptation. This comparison allows researchers to appreciate the unique contributions each type of nucleic acid makes to the life science tapestry.
Applications of RNA Sequence Templates
The realm of RNA sequence templates is a vital component in modern genetic research and development. Their applications stretch across various fields, most notably in biotechnology and therapeutic advancements. These templates play a critical role in understanding and manipulating genetic sequences, providing both innovative solutions and valuable insights into complex biological processes.
In Biotechnology
Bioengineering and biotechnology are perhaps the most practical areas where RNA sequence templates find their utility. These templates are key in the development of RNA interference (RNAi) technologies. By leveraging the specificity of RNA molecules, researchers can silence unwanted genes and investigate functions with precision.
A closer look at the applications:
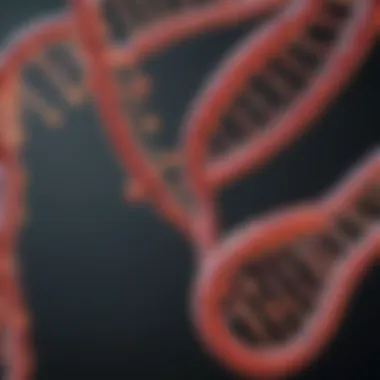
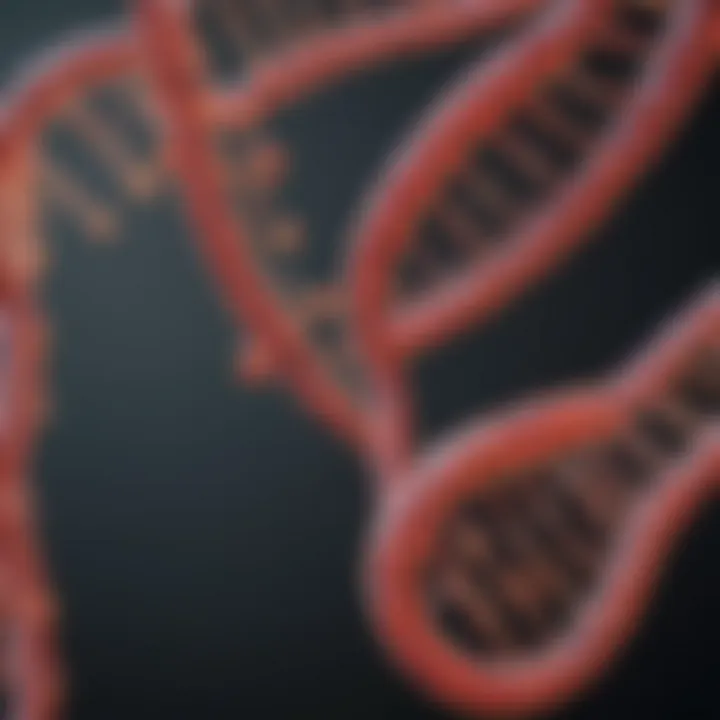
- Gene Editing: Tools like CRISPR utilize RNA templates to guide the editing process, allowing precise cuts at specific gene locations enhancing the accuracy of genetic modifications.
- Synthetic Biology: RNA sequence templates facilitate the creation of synthetic pathways in organisms, enabling them to produce desired outputs, like biofuels or pharmaceuticals. This direction paves the way for sustainable practices in various industries.
- Diagnostics: RNA templates serve as crucial components in molecular diagnostics, helping identify viral infections such as COVID-19 through reverse transcription polymerase chain reaction (RT-PCR) methods. These techniques provide rapid and reliable testing protocols, crucial for public health.
In sum, the versatility of RNA templates in biotechnology can hardly be overstated. They act as versatile tools that scientists can tailor for multiple objectives, clearly showcasing the importance of understanding RNA and its applications in real-world scenarios.
In Therapeutic Development
Advancing into the domain of therapeutic applications, RNA sequence templates are emerging as a groundbreaking tool for the development of new treatments. Notably, mRNA vaccines, prominent during the COVID-19 pandemic, have put a spotlight on how effective RNA technology can be in improving human health.
The key therapeutic applications include:
- Vaccination: The use of RNA templates in vaccine development has changed the landscape of immunization strategies. Instead of injecting inactive viruses, mRNA vaccines incite the body to produce a harmless spike protein, encouraging an immune response robustly.
- Gene Therapy: RNA templates enable the delivery of therapeutic genes into target cells. This approach holds promise for treating genetic disorders by correcting or replacing faulty genes, potentially curing diseases at their source.
- Targeted Therapies: Beyond genetic conditions, RNA sequence templates are paving the way for treatments in oncology. Current research focuses on harnessing RNA technologies to develop targeted therapies that minimize harm to healthy cells, offering a more refined approach compared to conventional treatments.
RNA templates are not just tools; they are unlocking doors to new possibilities in the therapeutic landscape, empowering advancements in medicine that felt like science fiction not so long ago.
Future Directions in RNA Research
As we delve into the dynamic landscape of RNA research, it becomes increasingly apparent that the horizon is dotted with promising advancements. The importance of this topic cannot be overstated, as the evolution of our understanding of RNA sequence templates continues to shape fields such as biotechnology and medicine. Researchers are not merely cataloging existing knowledge, but are actively pushing the boundaries to uncover novel insights. This ongoing exploration opens myriad avenues for new discoveries, ultimately impacting both theoretical and practical applications across various disciplines.
Emerging Technologies
The intersection of RNA studies with cutting-edge technologies presents a thrilling prospect. Techniques such as CRISPR-Cas9 gene editing and advancements in single-cell RNA sequencing are paving the way for breakthroughs. These methodologies facilitate a deeper understanding of RNA's role in gene regulation and expression, offering a clearer view of cellular mechanisms at play.
For example, single-cell RNA sequencing allows for the analysis of gene expression at the individual cell level, which is crucial for understanding complex cellular responses during development or disease progression. Here are some significant technological advancements:
- Nanopore Sequencing: This method allows for real-time analysis of RNA molecules, proving to be beneficial in diverse applications, such as detecting viral RNA quickly in clinical settings.
- Synthetic RNA Circuits: These innovations enable manipulating cellular processes, possibly leading to new therapeutic routes for diseases.
- RNA Therapeutics: Understanding how to effectively design RNA molecules for targeted therapy is a game changer, especially in treating complex diseases like cancer.
As these technologies progress, they not only enhance our ability to study RNA templates but also foster interdisciplinary collaboration—combining molecular biology with computational sciences, engineering, and bioinformatics.
Potential Research Areas
Looking forward, several promising areas of RNA research are ripe for exploration. Each has the potential to contribute to a greater understanding of biology:
- RNA Modifications: The significance of chemical modifications within RNA molecules is becoming clearer. Investigating these changes could yield insights into the regulation of gene expression, particularly in development and disease resistance.
- Exosomal RNA: Research is beginning to reveal the role of RNA contained in exosomes as biomarkers and mediators in intercellular communication. This area holds potential not just for basic science but also for developing diagnostic tools.
- RNA-Protein Interactions: Exploring how RNA interacts with proteins could shine a light on processes such as translation and post-transcriptional regulation, connecting to disease mechanisms.
- Synthetic Biology Applications: Creating synthetic RNA constructs for therapeutic purposes presents an intriguing possibility, allowing for targeted interventions in genetic disorders.
"The future of RNA research is not just about understanding the components, but about uncovering the complexities that govern life itself."
The End
The conclusion serves as a pivotal moment in this article, encapsulating not only the essence of RNA sequence templates but also their far-reaching implications in the world of genetics. The discussion has brought to light various aspects—from the basic structure of RNA to the innovative sequencing techniques that have emerged recently. Understanding these elements is not simply academic; it is the foundation upon which future advancements in biotechnology and therapeutic interventions are built.
Summary of Key Points
As a summary, we have explored:
- Definition and Importance of RNA: RNA is not merely a single-stranded molecule; it plays a critical role in various cellular processes, including protein synthesis and gene regulation.
- Components of RNA Sequences: Nucleotides, the building blocks of RNA, possess unique structures that contribute to its functionality. Each nucleotide carries specific information vital for biological processes.
- Role of Templates in Transcription: RNA templates guide the synthesis of RNA during transcription, underscoring their critical role in genetic expression.
- Sequencing Techniques: Innovations such as Next-Generation Sequencing have propelled our ability to analyze RNA at a granular level, enabling researchers to decode complex biological questions.
- Comparative Analysis with DNA: While RNA and DNA serve complementary roles in genetics, their structural and functional differences confer distinct advantages in various contexts.
- Applications in Science and Medicine: The implications of RNA templates extend into biotechnology and therapeutic development, illustrating their importance in advancing human health.
These points collectively illustrate the complexity and significance of RNA sequence templates in genetic science.
Final Thoughts on RNA Templates
In closing, the exploration of RNA sequence templates sheds light on an incredibly dynamic field that continues to evolve. Future research holds promise for unearthing new pathways for treating diseases, enhancing our understanding of genetic disorders, and possibly leading to breakthroughs in personalized medicine.
The implications of these templates are profound; they offer insights not just into the functioning of cells, but possibly the key to next-generation therapeutic strategies. As we look ahead, embracing the intricacies of RNA and its templates will be crucial. The future landscape of biotechnology and genetic medicine will likely be shaped by the knowledge we cultivate today.
" What we learn about RNA today will pave the way for innovations tomorrow."
By continuing to deepen our understanding of these molecular templates, we remain at the frontier of biological sciences, where every discovery may hold the potential for remarkable advancements.