Exploring the Structure and Applications of TCEP
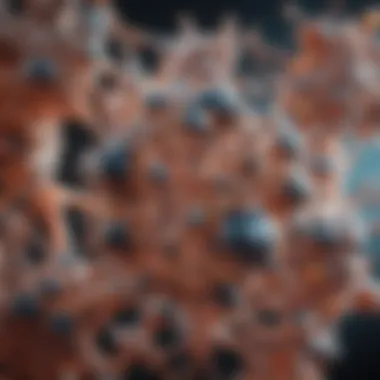
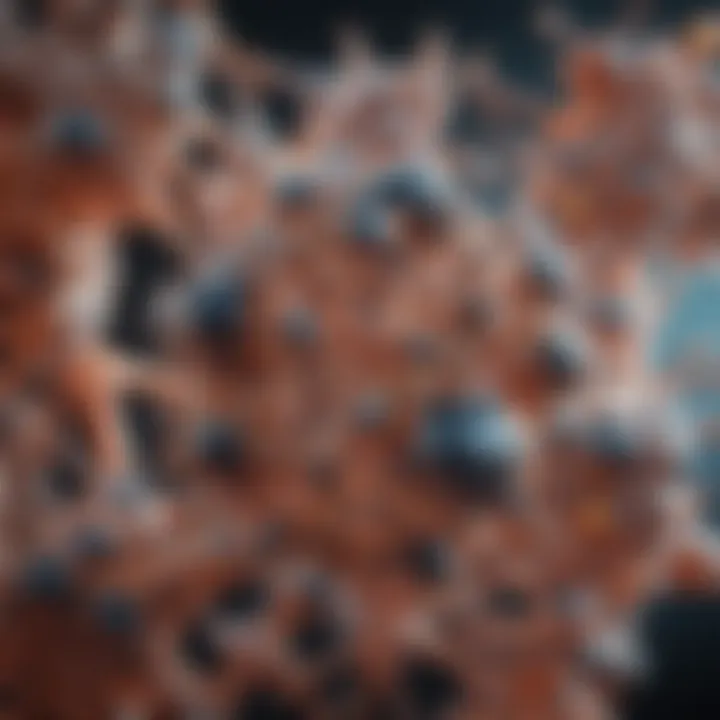
Intro
Tris(2-carboxyethyl)phosphine, commonly referred to as TCEP, has gained significant traction in biochemical and chemical research communities. TCEP is well-regarded for its effectiveness as a reducing agent, which enables it to maintain a stable form in various experimental conditions. Through this article, we aim to explore its structure comprehensively, highlighting both its theoretical significance and practical applications.
Research Overview
Summary of Key Findings
This article reveals the intricate structural properties of TCEP, detailing its molecular composition and unveiling the implications of its unique architectural features. The core findings illustrate that TCEP consists of three carboxyethyl groups bonded to a phosphorus atom, which imparts not only its reducing capabilities but also its solubility in aqueous environments. The ability to function under mildly acidic or neutral conditions makes TCEP a versatile choice for scientists.
Background and Context
The usage of reducing agents like TCEP is foundational in various fields, particularly when it comes to protein chemistry and material science. Prior to TCEP's popularization, agents such as dithiothreitol (DTT) were commonly employed. However, TCEP's advantageous properties, such as its stability and non-volatile nature, make it a favorable alternative in many laboratory settings. As research continues to evolve, understanding TCEP’s structure will provide insight into novel applications that can potentially benefit scientific studies.
Chemical Structure of TCEP
TCEP contains a central phosphorus atom that is covalently bonded to three carboxyethyl groups. This triadic arrangement is crucial for its reactivity. The presence of adjacent carboxylate groups enhances its nucleophilicity, enabling reductions of disulfides and other reactive groups in biological molecules.
Key Structural Features
- Phosphorus Atom: Central to its reducing ability.
- Carboxyethyl Groups: Provide steric bulk that helps prevent oxidation.
- Charged Nature: This contributes to its solubility in biological systems.
In summary, a thorough understanding of TCEP's structure not only elucidates its role as a reducing agent but also opens avenues for its application across a spectrum of scientific inquiries.
"The molecular architecture of TCEP embodies a crucial interplay between stability and reactivity, making it a preferred choice across various scientific fields."
Methodology
Experimental Design
Investigating the characteristics of TCEP involves studying its effectiveness in reducing conditions, usually set against benchmarks like DTT or mercaptoethanol. Various assays may be employed to observe its reaction kinetics and by-products produced during reductions.
Data Collection Techniques
Collecting data often involves spectroscopic methods such as NMR or mass spectrometry to confirm the identity and purity of TCEP in experimental setups. Furthermore, chromatography can be used to analyze the reaction products, ensuring that the understanding of TCEP's behavior is both accurate and comprehensive.
Prelims to TCEP
Tris(2-carboxyethyl)phosphine, commonly known as TCEP, serves a significant role in both biochemical and chemical research. Understanding TCEP's structure and applications is essential. This knowledge greatly impacts researchers and professionals in various fields, including life sciences and material sciences.
The relevance of TCEP lies in its effectiveness as a reducing agent, particularly in the stabilization of proteins and in reactions that require controlled environments. It is crucial to comprehend its molecular structure, which directly influences its chemical behavior. By exploring both its theoretical aspects and practical applications, we can better appreciate TCEP's contribution to modern scientific methods.
TCEP is favored for its stability under a wide range of conditions, making it suitable for delicate biochemical experiments. Its low toxicity further enhances its appeal, ensuring safety during laboratory use. Understanding TCEP allows us to utilize its properties to their fullest potential, leading to more efficient research outcomes. This section lays the foundation for a detailed exploration of TCEP's structure, mechanisms, and multifaceted applications.
Overview of TCEP
TCEP is a small-molecule phosphine compound, known for its ability to reduce disulfide bonds in proteins and peptides. The molecular formula of TCEP is C₆H₁₄O₆P, indicating the presence of carboxyethyl groups attached to a phosphorus center. These groups contribute to its high solubility in aqueous solutions, making it ideal for biological systems.
A few critical features of TCEP include:
- Redox potential: Capable of efficiently converting disulfide bonds to free thiols.
- Chemical stability: Does not degrade in presence of heat or light, unlike other reducing agents.
- Compatibility: A minimal impact on enzymatic activity, which preserves the functionality of proteins in experimental settings.
Historical Context
The discovery and development of TCEP occurred in the late 20th century, addressing a need for more reliable reducing agents in laboratory settings. Researchers sought alternatives to existing agents such as dithiothreitol (DTT), which, while effective, posed challenges in terms of stability and periodic toxicity.
TCEP emerged as a solution, providing an option that ensured enhanced performance, particularly regarding protein stability during chemical reactions. Its importance in reducing disulfide bonds quickly became evident, leading to its adoption in numerous protocols. Over time, studies have continued to further explore TCEP's advantages and applications across different scientific domains, establishing it as a staple in biochemical and chemical research.
Chemical Composition of TCEP
The chemical composition of Tris(2-carboxyethyl)phosphine, commonly known as TCEP, plays a significant role in its function and applications within biochemical and chemical research. Understanding its molecular structure helps elucidate how TCEP acts as a reducing agent and what properties make it suitable for various experiments and industrial processes. It is critical to examine the specific elements that comprise TCEP, as well as the implications of its unique structure on its stability, solubility, and reactivity.
Molecular Formula and Structure
TCEP is chemically represented by the molecular formula C₆H₁₄O₆P. Its structure features a central phosphorus atom surrounded by three 2-carboxyethyl groups. Each of these groups comprises a carboxyl functionality that enhances the compound’s ability to participate in redox reactions. The arrangement of atoms offers some insight into the electronic characteristics that make TCEP effective in reducing overly oxidized molecules.
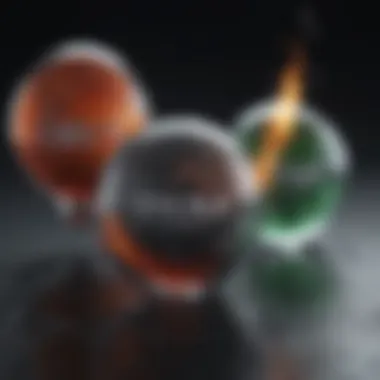
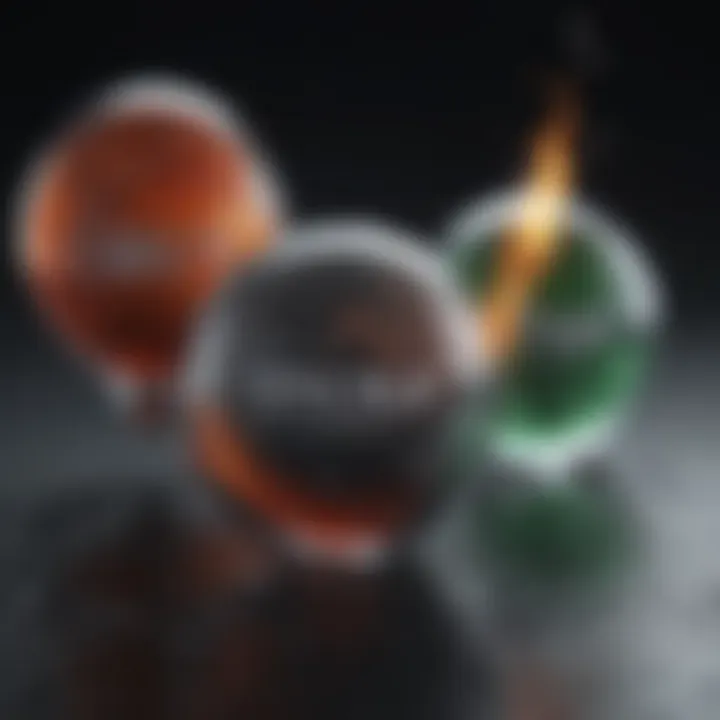
The spatial configuration of TCEP is noteworthy. The steric hindrance from the bulky carboxyethyl groups provides a certain level of protection to the phosphorus center. This structure enhances its reactivity towards disulfide bonds, making it particularly useful in biochemical applications.
Physical Properties of TCEP
The physical properties of TCEP encompass stability, solubility, and reactivity, all of which contribute to its effectiveness as a reducing agent.
Stability
Stability is a crucial aspect when evaluating chemical agents. TCEP is notably stable under a variety of conditions, including exposure to air and light. This resistance to oxidation is essential, as it prevents the compound from converting to less effective forms during storage or usage. Another key characteristic is TCEP's performance over a range of pH levels. This broader stability translates to versatility in experimental setups across different biological systems.
Advantages of TCEP’s stability include:
- Reduced loss of efficacy over time.
- Compatibility with sensitive biochemical assays.
- The capability to function effectively in both acidic and basic environments.
However, it is important to consider that not all experiments can maintain optimal stability, especially where extreme pH adjustments are needed.
Solubility
Solubility is another critical physical property that influences TCEP's practical applications. TCEP is soluble in water and various organic solvents, which makes it suitable for a wide array of formulations and methodologies. The high solubility contributes to its ease of use in laboratory settings, allowing quick preparation of stock solutions.
This aspect has several beneficial implications:
- Facilitates incorporation in biochemical assays involving proteins and nucleic acids.
- Ensures rapid reduction of disulfide bonds in aqueous environments.
On the downside, the solubility might present challenges in purely organic systems as it may not perform as well without an adequate polarity in the solvent.
Reactivity
Reactivity is the cornerstone of TCEP’s function as a reducing agent. It exhibits a strong capability to cleave disulfide bonds in biomolecules. This property is pivotal for applications such as protein denaturation and enzyme activity studies. The reactivity of TCEP is generally high, which allows for rapid interaction with target molecules.
Significant traits include:
- Effective at low concentrations, making it cost-efficient for laboratory use.
- Rapid reaction kinetics, which allows for efficient experimental timelines.
Nevertheless, TCEP can be selective in its reactivity, which may limit its utility in certain reactions where other functionalities are present.
In summary, the chemical composition, physical properties, and specific features of TCEP underlie its effectiveness as a reducing agent. Understanding these properties empowers researchers and practitioners to leverage TCEP optimally in various experimental contexts.
Synthesis of TCEP
The synthesis of Tris(2-carboxyethyl)phosphine, or TCEP, is vital due to its implications in both biochemical and industrial contexts. Understanding the methods and approaches used to create TCEP highlights its versatility and efficiency as a reducing agent. This section focuses on the chemical synthesis methodologies and purification techniques, emphasizing their importance in ensuring the quality and effectiveness of TCEP for various applications.
Chemical Synthesis Methods
The development of TCEP largely stems from two synthesis methods: phosphorylation and carboxylation. Each offers unique advantages and considerations that influence their adoption in different scenarios.
Phosphorylation Techniques
Phosphorylation techniques are essential in the formation of TCEP. These methods typically involve introducing phosphate groups into organic compounds, helping to form the core structure of TCEP. A key characteristic of phosphorylation is its ability to yield TCEP with high purity and specificity. This not only streamlines the process but also ensures the final product's effectiveness in applications.
The phosphorylation routes often utilize readily available starting materials. This accessibility makes the method both efficient and cost-effective. Additionally, one unique feature of these techniques is the possibility of fine-tuning the reaction conditions to optimize the yield.
However, drawbacks exist. Some phosphorylation methods may require specific catalysts or conditions that complicate the process, leading to variable results. Despite these challenges, the overall benefits position phosphorylation as a popular choice in TCEP synthesis.
Carboxylation Processes
Carboxylation processes play a significant role in generating the carboxyethyl groups found in TCEP. This technique involves the addition of carboxyl groups to organic substrates, which is crucial for the chemical identity of TCEP. A notable characteristic of carboxylation is its straightforward execution, which often results in high yields of product. This makes it an appealing option for laboratories seeking efficiency.
The unique aspect of carboxylation is its compatibility with a variety of reagents, leading to more flexible synthetic pathways. However, there are limitations. Some reactions may produce byproducts that necessitate additional purification steps, slightly complicating the synthesis.
In summary, both phosphorylation techniques and carboxylation processes contribute significantly to TCEP synthesis. While each has its strengths and weaknesses, their combined use allows for the production of TCEP that meets the demands of diverse applications.
Purification Techniques
Purification is a critical step following the synthesis of TCEP. Ensuring the removal of impurities is necessary to maintain the effectiveness of TCEP as a reducing agent. Common purification techniques include crystallization and chromatography.
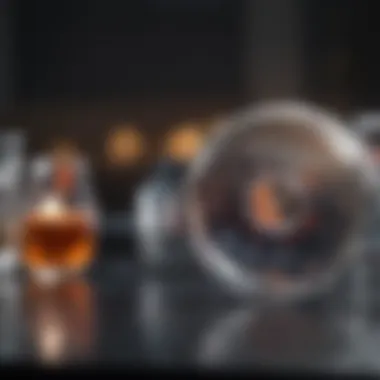
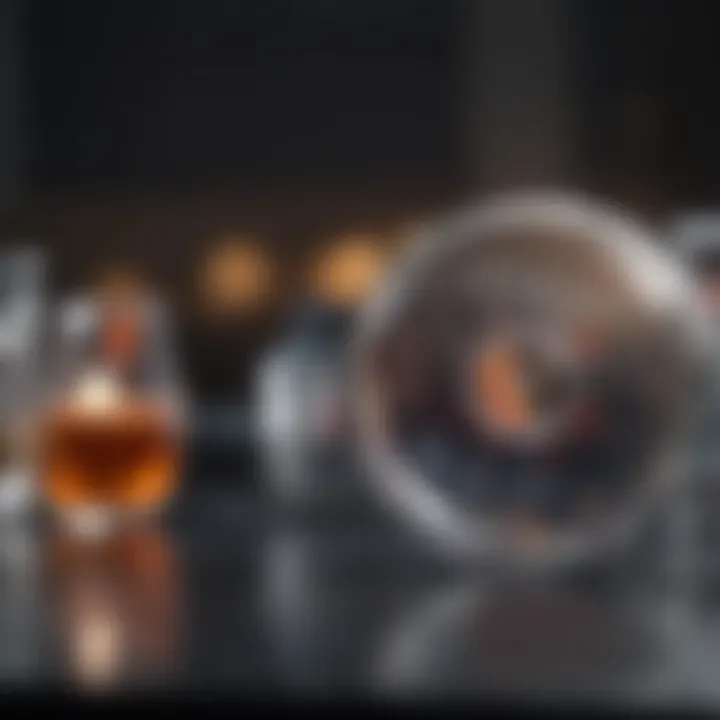
Crystallization is often employed due to its simplicity and effectiveness, allowing for the separation of TCEP from unwanted substances based on solubility differences. In contrast, chromatography provides higher resolution, making it suitable for cases where impurities are closely resembling TCEP in structure. Both methods emphasize the need for meticulous attention to detail in the purification phase, as the quality of the end product directly impacts its application in biochemistry and materials science.
The synthesis of TCEP exemplifies the careful balancing of chemical engineering techniques and the nuanced understanding of molecular behavior. As researchers continue to innovate in this area, ongoing studies into synthesis and purification techniques will further enhance the overall contributions of TCEP in various fields.
Mechanism of Action
Understanding the mechanism of action of Tris(2-carboxyethyl)phosphine (TCEP) is essential for grasping its role as a potent reducing agent in both biochemical and chemical applications. TCEP primarily functions through its ability to transfer electrons in redox reactions, thereby altering the oxidation state of other molecules. This property signifies its importance in various experiments where the reduction of disulfide bonds is necessary. Recognizing how TCEP interacts with target molecules provides critical insight into its efficacy and versatility as a reagent.
Redox Chemistry
Redox chemistry is central to TCEP's functionality. The term "redox" implies simultaneous reduction and oxidation reactions. When TCEP interacts with substrates containing disulfide bonds, it donates electrons to those bonds, breaking them and reducing them to free thiol groups. This characteristic is particularly significant because free thiols are often more reactive and can undergo further chemical modifications.
TCEP is favored due to its stability compared to other common reducing agents like dithiothreitol (DTT). Its stable structure ensures reliable performance in various conditions, including differing pH levels and temperatures. Moreover, TCEP does not form adducts with thiols, avoiding potential complications in biochemical analyses.
Reaction Pathways
The reaction pathway of TCEP involves several key steps. Initially, TCEP targets the disulfide bond, where it interacts with the sulfur atoms. TCEP then undergoes its own oxidation, facilitating the release of electrons. This reaction can be diagrammatically expressed, though it is often described in qualitative terms in practical applications.
- TCEP Initiates Reaction: TCEP encounters a substrate with disulfide bonds.
- Electron Transfer Occurs: Electrons from TCEP are transferred to the disulfide bond.
- Formation of Free Thiols: The disulfide bond is cleaved, yielding two thiol groups.
- Oxidized TCEP: Following the reaction, TCEP becomes oxidized, completing the cycle of redox interaction.
This understanding of TCEP's reaction pathways highlights its utility in biochemical research, particularly in protein chemistry, where controlled reduction of disulfide bonds is crucial for studying protein structure and function.
In summary, the mechanism of action of TCEP, rooted in redox chemistry and defined pathways, illustrates its essential role in manipulating molecular structures in both laboratory and industrial settings. Its advantages over other reducing agents and clear reaction pathways solidify its prominence in scientific research.
Applications of TCEP
TCEP, or Tris(2-carboxyethyl)phosphine, has several important applications spanning biochemical research and industrial uses. The significance of these applications lies in TCEP's unique properties, which facilitate various chemical reactions while maintaining stability across a broad range of conditions. Understanding these applications can enhance the effectiveness of experiments and processes that rely on TCEP as a reducing agent.
Use in Biochemical Research
In biochemical research, TCEP serves as a vital tool in many applications, especially in protein biochemistry and molecular biology. Its efficacy is particularly noted in protein reduction and disulfide bond cleavage.
Protein Reduction
Protein reduction using TCEP involves converting disulfide bonds in proteins into free thiols. This process is essential for various research methods, such as mass spectrometry, where it is crucial to analyze polypeptides accurately. TCEP's key characteristic is its ability to reduce these bonds without forming reactive byproducts, making it a favorable choice over alternatives like dithiothreitol (DTT). The unique feature of TCEP is its stability under directly physiological conditions, allowing for prolonged use in sensitive experiments. Its advantages include non-toxic byproducts and lack of reactivity with amines, which supports cleaner biochemical applications. However, one of the disadvantages is the necessity of careful handling due to its phosphine nature.
Disulfide Bond Cleavage
The cleavage of disulfide bonds is another critical application in which TCEP excels. This process rejuvenates the functional qualities of proteins by enabling them to refold correctly or be modified without interference. The capability to cleave these bonds efficiently positions TCEP as a beneficial compound in many workflows, especially where restructuring proteins is essential. Its prominent feature is the controlled and efficient cleavage it provides, allowing researchers to achieve their objectives with fewer side reactions. Although its high cost compared to traditional methods could be a consideration, the quality and reliability it delivers often justify the expense for high-stakes applications.
Industrial Applications
TCEP also proves itself valuable in various industrial applications, particularly in polymer chemistry and nanotechnology. Its properties make it conducive for use in areas that demand precision and reliability.
Polymer Chemistry
In the realm of polymer chemistry, TCEP acts as a reducing agent during the synthesis and manipulation of various polymers. Its properties allow for effective reduction processes necessary for enhancing polymer stability and performance. This characteristic elevates TCEP's role as a favorable agent in developing advanced materials, enabling finer control over polymer structures. Notably, while TCEP is beneficial due to its efficacy, its relatively higher cost and specific handling requirements could be a disadvantage when compared to more traditional reducing agents.
Nanotechnology
Within nanotechnology, TCEP plays a fundamental role in the creation of nanomaterials. It aids in modifying surfaces and enhancing the properties of nanosystems. The key aspect of TCEP in this field is its ability to facilitate reductions without introducing contamination or altering the properties of nanostructures adversely. This unique feature positions it well in applications demanding high purity and stability. However, the challenge remains in the scalability of processes involving TCEP, which may limit its use in massive production scenarios.
TCEP's versatility as a reducing agent is crucial in both biochemical and industrial applications, underlining its relevance in continuous research and development efforts.
In summary, the applications of TCEP are diverse and serve critical roles in the advancement of biochemical processes and industrial methodologies. Its attributes exemplify the ongoing need for reliable reducing agents in contemporary science.
Advantages of TCEP over Other Reducing Agents
TCEP (Tris(2-carboxyethyl)phosphine) is recognized for its superior qualities when compared to traditional reducing agents such as dithiothreitol (DTT). Understanding these advantages is essential in various practical applications, especially in biochemical and chemical research. This section dives into the specific elements that make TCEP a preferred choice among researchers and professionals.
Stability Compared to DTT
One notable advantage of TCEP is its exceptional stability under varied conditions. Unlike DTT, which can undergo oxidation over time, TCEP remains stable for extended periods. This stability allows researchers to store solutions longer without losing reducing capacity.
TCEP is effective across a broad pH range and does not react negatively with reactive oxygen species. This characteristic reduces the risk of unwanted side reactions that could compromise experimental outcomes. Furthermore, TCEP does not readily form thiol esters or disulfides, which are common issues with DTT. This reliability in stability translates to more consistent experimental data, crucial for any research involving protein chemistry or molecular biology.
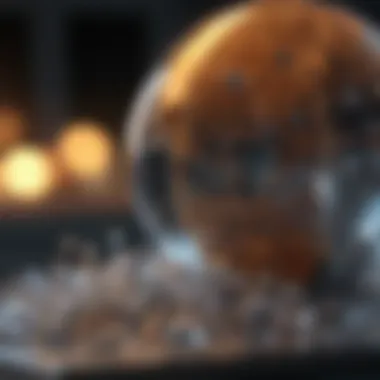
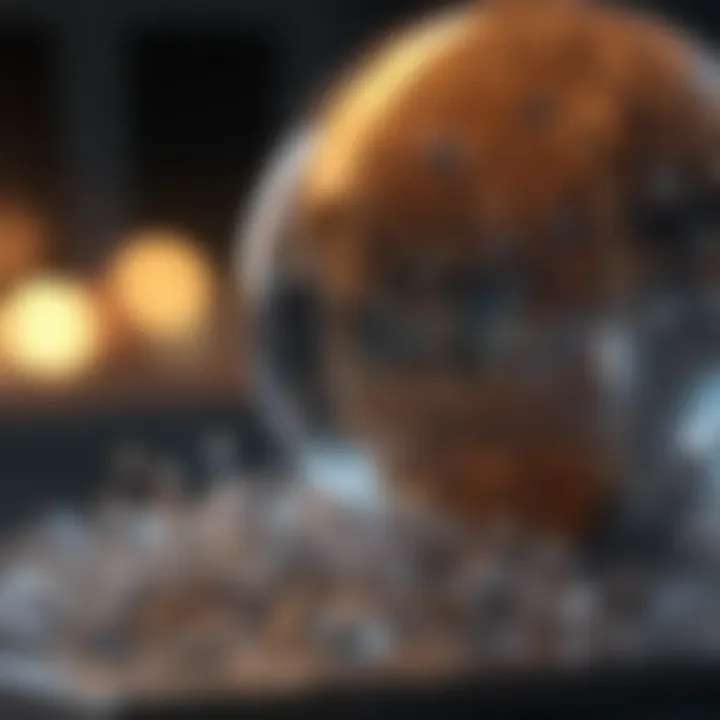
Compatibility with Biological Systems
TCEP's compatibility with biological systems is another significant benefit. Many reducing agents interfere with biological processes, but TCEP is less likely to cause issues in sensitive environments. For example, TCEP does not affect enzyme activity or the structure of target proteins adversely. This feature makes TCEP suitable for applications in live cell studies or delicate in vitro systems.
Additionally, TCEP is non-toxic to cells at the concentrations typically used in research. It does not pose the same safety concerns associated with other reducing agents, such as DTT, which can exhibit cytotoxicity. Researchers working in a biological context will find TCEP easier to integrate into protocols without the added worry of toxic by-products
TCEP's use in various protocols—ranging from protein refolding to disulfide bond cleavage—demonstrates its versatility and importance. These traits contribute to a thorough understanding of protein structures and functions with minimal interference from the reducing agent itself.
TCEP offers a blend of stability and biological compatibility that outclasses traditional reducing agents.
Safety and Handling of TCEP
Understanding the safety and handling procedures of Tris(2-carboxyethyl)phosphine (TCEP) is essential for anyone working with this reducing agent. Not only does TCEP serve vital functions in biochemical and industrial applications, but it also carries specific safety considerations. Proper management of TCEP minimizes risks while maximizing its benefits in research and production.
Toxicological Information
TCEP is regarded as having a favorable safety profile relative to other reducing agents. However, this does not mean it is devoid of toxicological risks. Studies indicate that TCEP may cause irritation upon dermal or ocular contact. Inhalation exposure could also lead to respiratory discomfort. Research has shown that TCEP can have adverse effects in biological systems, prompting several investigations into its toxicological properties.
It is crucial to understand that TCEP is classified as a potential irritant. Long-term exposure, particularly in poorly ventilated areas, could pose health risks. See above for detailed studies on the chemical's effects on human health and environmental safety for better context.
When utilizing TCEP, ensure to keep the product well-labeled and stored appropriately to prevent accidental exposure. Some recommended safety limits may note a permissible exposure level, which should always be observed to ensure safety.
Recommended Safety Protocols
When handling TCEP, specific safety protocols must be implemented to protect users and laboratory environments. Key recommendations include:
- Personal Protective Equipment (PPE): Wear gloves, safety goggles, and lab coats to minimize risk of exposure.
- Ventilation: Conduct work involving TCEP in a fume hood to ensure proper air exchange and reduce inhalation risks.
- Storage: Store TCEP in tightly sealed containers in a cool, dry place away from incompatible substances.
- Disposal: Follow local regulations for chemical disposal. TCEP should not be released into the environment, and waste disposal methods should be appropriately managed.
- Emergency Procedures: Familiarize yourself with first aid protocols for exposure incidents. In case of contact with skin or eyes, rinse immediately with plenty of water and seek medical attention if irritation persists.
"Proper understanding and management of TCEP's safety guidelines can prevent accidents and ensure a safer laboratory environment."
Following these safety protocols enhances the safe application of TCEP and mitigates any potential hazards related to its use. Establishing such measures allows for productive research activities without compromising health and safety.
Future Directions in TCEP Research
Research on TCEP, specifically its future directions, is crucial. Understanding its potential in various fields can lead to significant advancements in both theory and application. The versatility of TCEP provides opportunities to explore novel methods, advanced techniques, and innovative applications. Researchers aim to uncover efficiencies in biochemical processes and manufacturiing that TCEP could enhance.
Innovative Applications
TCEP's role as a reducing agent is well established, but its innovative applications merit deeper exploration. For instance, TCEP can facilitate more efficient synthesis processes in the pharmaceutical industry. By applying TCEP in the synthesis of complex molecules, researchers may overcome challenges such as excessive reaction times and low yield.
Moreover, the compatibility of TCEP with various solvent systems enhances its utility. Investigating its performance in non-aqueous mediums can yield new pathways in organic synthesis. Some examples include its potential utilization in creating new polymers and nanomaterials. These materials hold promise for advanced technologies like drug delivery systems and sensors, which could benefit from TCEP’s properties.
In summary, TCEP can drive numerous innovations in diverse scientific fields. As the research community persists in uncovering these potentials, new applications will undoubtedly emerge.
Continued Investigations
Continued investigations into TCEP require systematic studies to evaluate its effectiveness and safety in different contexts. One approach involves focused research on its toxicological profile. Understanding how TCEP interacts with biological systems is vital for advancing its applications in life sciences. By establishing reliable protocols for TCEP's use in laboratories, researchers can ensure safe practices and mitigate risks.
Additionally, the development of new analytical methods would greatly benefit the study of TCEP. Improved techniques can provide insights into TCEP's behavior under various conditions, such as temperature and pH. These examinations can lead to better strategies in utilizing TCEP in both academic research and industrial applications.
In summary, sustained focus on TCEP through both theoretical and experimental frameworks will broaden its application spectrum. By continuing to investigate its properties, researchers can unearth valuable information that contributes to scientific advancement across multiple sectors.
Culmination
The conclusion of this article is pivotal in encapsulating the key insights and discussions surrounding Tris(2-carboxyethyl)phosphine, or TCEP. Bringing together various aspects of TCEP, from its structural intricacies to its vast applications, this section underscores the larger significance of TCEP in both biochemical research and material sciences.
Summary of Key Points
In summarizing, we have explored the following critical components:
- TCEP's molecular structure and chemical composition.
- Its stability and compatible use within biological systems, contrasting with other reducing agents like DTT.
- The various synthesis and purification methods that yield high-quality TCEP for research applications.
- The broad spectrum of applications which include biochemical research and its role in industries, from polymer to nanotechnology.
- Safety measures and handling protocols necessary for working with TCEP in the lab environment.
These points collectively highlight the role TCEP plays as a necessary tool for researchers who utilize its reducing properties, thereby enabling the advancement of studies in protein interactions and material innovations.
Final Thoughts on TCEP
TCEP's significance cannot be overstated in modern scientific research. It provides a robust alternative to traditional reducing agents, characterized by its stability and lack of interference in biological environments. As research evolves, TCEP may find even more innovative applications that extend beyond current practices.
Moreover, as we look to the future, further investigations into TCEP's capabilities can lead to enhanced methodologies in both life sciences and materials engineering. Integrating TCEP in diverse applications not only streamlines research processes but may also foster groundbreaking discoveries.
"TCEP has emerged as an indispensable tool in the toolkit of modern biochemists, reshaping how we think about and conduct experiments."