Exploring Biomedical Engineering in Neuroscience
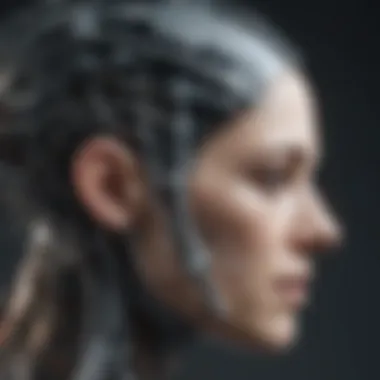
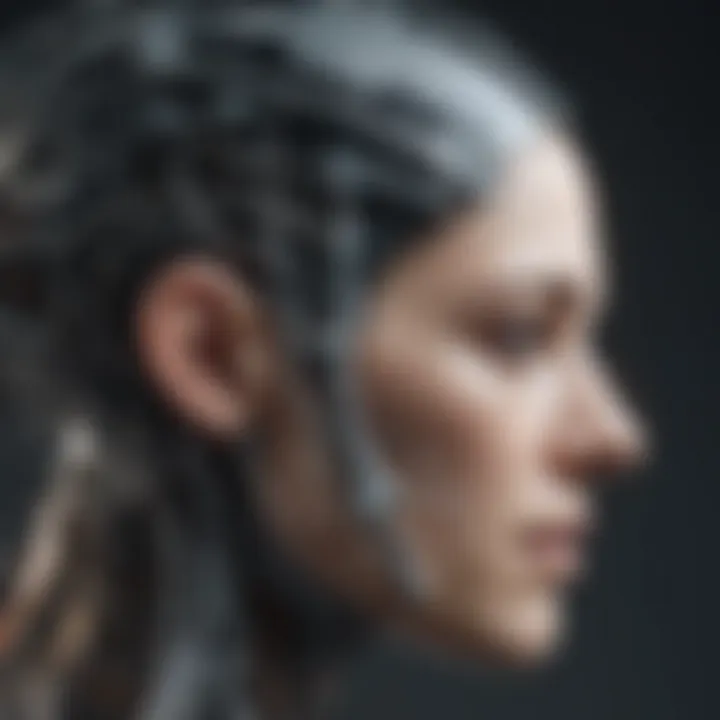
Intro
The fields of biomedicine and neuroscience have traditionally operated in separate silos. However, recent developments reveal a profound interplay between these disciplines. Biomedical engineering applies engineering principles to healthcare, while neuroscience delves into the functioning of the nervous system. By merging these areas, we uncover pathways to innovative medical treatments, advanced technologies, and novel insights into brain function.
This article provides a detailed examination of this intersection, guiding the reader through crucial topics such as neural prosthetics and brain-computer interfaces. Understanding these technologies is essential for both medical professionals and researchers alike.
Research Overview
Summary of Key Findings
The integration of biomedical engineering in neuroscience has led to significant advancements. Key findings include:
- Neural prosthetics have emerged to assist individuals with motor disabilities.
- Brain-computer interfaces allow direct communication between the brain and external devices.
- Neuroimaging technologies enhance both diagnosis and treatment of neurological conditions.
These advancements not only improve patient care but also offer deeper understanding of the brain's complex functions.
Background and Context
For decades, neuroscience has been primarily focused on understanding the underlying mechanisms of the nervous system. Simultaneously, biomedical engineering has developed tools to tackle complex medical problems. The convergence of these two areas has been driven by the need for effective treatment options and technology that can interact with the nervous system.
The realization that engineering solutions can significantly enhance neuroscience applications has paved the way for groundbreaking techniques. Prototypes of devices such as neural stimulators are now routinely tested in clinical settings. This growing synergy signals a shift toward more collaborative research models.
Methodology
Experimental Design
Research in this field commonly utilizes interdisciplinary approaches. Studies often incorporate:
- Control groups to measure the effectiveness of new technologies.
- Longitudinal designs that track patient outcomes over time.
- Cross-sectional studies to assess correlations between variables, such as technology use and improvement in motor skills.
These designs provide a comprehensive view of the impacts of biomedical engineering on neurological health.
Data Collection Techniques
Data collection varies based on the nature of the research but typically includes:
- Surveys and questionnaires to gather patient feedback and experiences.
- Clinical assessments, involving tests administered by medical professionals to evaluate improvements in neurological function.
- Imaging techniques, such as MRI and CT scans, used to visualize changes in the brain.
These methods facilitate a multi-faceted understanding of how engineering innovations influence neuroscience.
"The fusion of biomedical engineering and neuroscience holds transformative potential, ushering in new technological paradigms for neurological therapies."
Through this exploration, the article will discuss specific applications, ongoing research, and ethical considerations that arise in this rapidly evolving field.
Preamble to Biomedical Engineering
Biomedical engineering sits at the pivotal junction of applied sciences, mathematics, and health care, making it a critical area of study in the context of this article. It is a field that integrates principles from engineering, biology, and medicine to create solutions that address complex health challenges. This integration is particularly significant as it enhances our understanding of the human body, facilitating the development of advanced medical technologies. By focusing on the creation of devices or innovations meant to improve patient care, biomedical engineering not only influences therapeutic approaches but also redefines patient interactions with medical equipment.
Definition and Scope
Biomedical engineering can be defined as the application of engineering principles to the biological and medical fields. It encompasses a broad range of activities that include the design, analysis, and development of medical devices, diagnostic equipment, and therapeutic interventions. The scope extends to areas such as biomaterials, medical imaging, and even tissue engineering. Through this interdisciplinary approach, professionals aim to innovate tools and methodologies that can enhance diagnostic procedures and treatment methodologies. This collaborative effort leads to improved overall health outcomes, making it an essential component in contemporary medical advancements.
Historical Context
The historical evolution of biomedical engineering can be traced back to innovations such as the artificial heart and the development of imaging technologies. These pioneering efforts laid the groundwork for future breakthroughs. The field has grown significantly since the 20th century, driven by advancements in technology and a deeper understanding of human physiology. Early achievements, like organ transplantation and non-invasive imaging, shaped the landscape and set bold visions for future innovations. A significant milestone emerged in the late 1970s, when regulatory processes began to formalize the evaluation of medical devices, which pushed the boundaries of safety and efficacy in biomedical engineering.
Relation to Other Engineering Fields
Biomedical engineering is inherently interdisciplinary, borrowing concepts from various engineering fields such as mechanical, electrical, and materials engineering. For instance, mechanical engineering contributes to the design of prosthetic limbs and rehabilitation devices by providing insights on biomechanics and material characteristics. Electrical engineering plays a crucial role in the development of imaging technologies, such as MRI and CT scans, facilitating the visualization of internal structures without invasive procedures. This cross-disciplinary framework ensures that biomedical engineers can tackle complex health issues holistically, leveraging diverse engineering principles to create effective solutions that improve patient care outcomes.
An Overview of Neuroscience
Neuroscience is a critical domain that helps us understand the complex workings of the brain and nervous system. This discipline combines biology, psychology, and engineering principles to explore how these systems influence human behavior and cognition. Given the profound impact of neuroscience on our understanding of health and disease, it provides foundational knowledge necessary for advancements in biomedical engineering.
Fundamental Concepts
At its core, neuroscience studies the structure and function of the nervous system. Key concepts include:
- Neurons: The fundamental building blocks of the nervous system, responsible for transmitting signals throughout the body.
- Synapses: These are the junctions between neurons where communication occurs, showcasing the complexity of neural networks.
- Neurotransmitters: Chemical messengers that facilitate communication between neurons, influencing many physiological processes.
Understanding these concepts is essential for those in biomedical engineering. It allows for the development of devices and treatments that interact with the nervous system, enhancing therapeutic outcomes.
Neural Systems and Functions
Neuroscience examines various neural systems and their functions. These include:
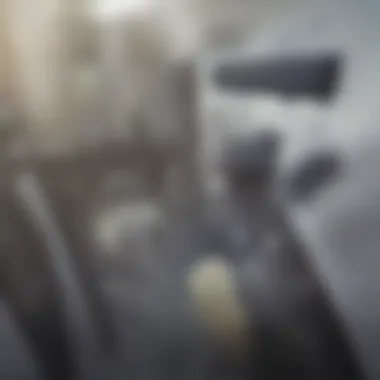
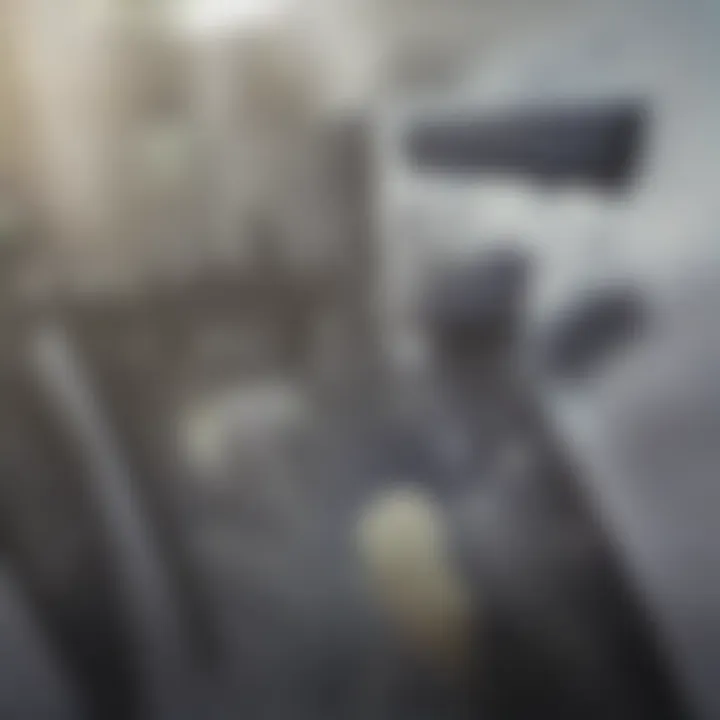
- Central Nervous System: Comprising the brain and spinal cord, it processes sensory information and directs responses.
- Peripheral Nervous System: This system connects the CNS to limbs and organs, facilitating communication between the brain and the rest of the body.
- Autonomic Nervous System: Controls involuntary actions, such as heartbeat and digestion, playing a vital role in maintaining homeostasis.
By understanding these systems, biomedical engineers can design interventions, such as neural prosthetics, that support or mimic lost functions, aiding in rehabilitation and recovery processes.
Current Trends in Neuroscience Research
Research in neuroscience is evolving rapidly, with several promising trends shaping the field, including:
- Neuroimaging Technologies: Advancements in imaging techniques, like fMRI and PET scans, allow scientists to visualize brain activity in real-time.
- Neuroplasticity Studies: Investigating how the brain adapts and reorganizes itself in response to injury or learning highlights therapeutic possibilities.
- Artificial Intelligence Integration: Utilizing AI to analyze brain data enhances the understanding of complex brain functions and disorders.
Such trends reveal the potential for interdisciplinary collaboration. By merging neuroscience with biomedical engineering, we can create innovative solutions for neurological diseases and improve patient care.
The synergy between neuroscience and biomedical engineering is essential for advancing our comprehension of the brain and developing effective treatments.
The Synergy of Biomedical Engineering and Neuroscience
The convergence of biomedical engineering and neuroscience represents a crucial evolution in both fields. This synergy leads to groundbreaking innovations and opens pathways to enhanced medical treatments and improved patient outcomes. The integration of engineering principles within neuroscience enables us to address complex biological systems more effectively. It encourages interdisciplinary collaboration that can yield novel solutions to longstanding medical challenges. Furthermore, this relationship provides insights that help us understand the intricate workings of the brain while developing systems that can interact with it.
Applications in Medical Devices
Biomedical engineering has led to the creation of sophisticated medical devices that work based on principles drawn from neuroscience. These devices range from implantable technologies to wearable systems, all designed to monitor and manipulate neural processes. The development of such devices has substantial implications in various medical sectors.
- Neurostimulators: These devices can modulate brain activity to alleviate symptoms in conditions like Parkinson's disease or epilepsy.
- Wearable EEGs: These allow for continuous monitoring of brain activity outside clinical settings.
- Neural recording devices: They capture signals from individual neurons, useful for advancing research and developing targeted therapies.
The significance of these devices in clinical settings cannot be understated. They not only help in diagnosing various neurological conditions but also serve therapeutic purposes. For instance, neurostimulators can improve patients' quality of life by directly targeting the areas of the brain responsible for specific symptoms.
Smart Prosthetics for Neural Damage Rehabilitation
Smart prosthetics are another remarkable outcome of the intertwining of biomedical engineering and neuroscience. These devices are designed not merely as artificial limbs but as advanced systems that can restore a degree of normal function to individuals with neural damage. They leverage neural inputs to allow for intuitive, volitional control.
- Seamless integration: Smart prosthetics are equipped with sensors that detect muscle signals, translating them into actions. This creates a more natural experience for the user.
- Feedback systems: Advanced models provide sensory feedback. This allows the user to have a sense of touch and proprioception, critical for adapting to new prosthetic limbs.
These innovations have transformed rehabilitation approaches, allowing people to regain autonomy and improve their quality of life. However, these technologies often require rigorous testing and validation to ensure safety and efficacy.
Neuroengineering and Its Impact on Treatments
Neuroengineering sits at the crossroads of biomedical engineering and neuroscience, focusing on the design of devices that can improve neural health and function. This specialization has immense potential for developing new treatments.
- Brain-computer interfaces (BCIs): These devices offer direct communication pathways between the brain and external devices, aiding those with severe mobility impairments.
- Neurostimulation techniques: These include transcranial magnetic stimulation (TMS), a non-invasive method that has shown promise in treating depression and other mental health disorders.
- Targeted delivery of therapeutic agents using nanotechnology systems that can release drugs at specific sites within the nervous system.
The impact of these developments leads to more targeted, effective treatments that address the underlying causes of disorders rather than just alleviating symptoms.
"The merging of these two fields is essential for fulfilling the promise of personalized medicine in neurology."
In summary, the synergy of biomedical engineering and neuroscience fosters innovation across various applications, from advanced medical devices to neurorehabilitation strategies. As research in these fields evolves, we can anticipate significant advancements that will continue to affect patient care profoundly.
Neural Prosthetics: A Case Study
Neural prosthetics represent a critical link between biomedical engineering and neuroscience. This field involves devices that are designed to replace or enhance the functionality of lost brain functions due to injury, disease, or congenital defects. Their significance cannot be understated, as they have the potential to restore independence and improve the quality of life for many individuals. In this section, we will examine the development, clinical applications, and the challenges faced in this evolving field.
Development and Design
The development of neural prosthetics requires a thorough understanding of both engineering principles and neuroscience. The design process typically begins with identifying the specific needs of the patients. For example, if a patient suffers from paralysis, a neural prosthetic may be designed to interpret signals from the brain and translate them into movements. The engineering phase involves materials selection, device miniaturization, and connectivity design with neural tissues, often requiring a combination of flexible electronics and bio-compatible materials.
A few notable components include:
- Electrodes: These are crucial for interfacing with neural tissue, gathering signals, and stimulating neurons. The choice of electrode design directly impacts their performance and the body’s acceptance of the device.
- Signal Processing Units: These units filter and decipher complex neural signals. Advanced algorithms can convert raw data into actionable outputs for movement or stimulation.
- Power Source: Long-lasting and safe power sources are vital as they must operate continuously without frequent replacement.
Clinical Applications and Success Stories
Neural prosthetics have already shown significant promise in clinical settings. Notable applications include:
- Cochlear Implants: These devices have transformed the lives of individuals with profound hearing loss by providing auditory perception. They convert sound into electrical signals that stimulate the auditory nerve.
- Retinal Implants: Similar to cochlear devices, retinal implants help restore vision for those suffering from conditions like retinitis pigmentosa.
- Brain-Computer Interfaces: One compelling case involves a paralyzed patient who regained the ability to control a computer cursor using thoughts alone, with neural signals captured and decoded by the device.
Success stories like these underscore the potential of neural prosthetics to significantly restore lost functions, revolutionizing treatments in rehabilitation.
Limitations and Challenges
Despite the advancements, neural prosthetics face several limitations and challenges. Some include:
- Complexity of Neural Signals: The brain's intricacies make it difficult to decode signals accurately, leading to imperfect functionality of devices.
- Invasive Procedures: Many neural prosthetics require surgeries for implantation, posing risks to patients and often leading to complications.
- Adaptation and Learning Curve: Users may need time to adapt to the devices, requiring extensive training and therapy to maximize functionality.
- Ethical Considerations: There are significant ethical discussions around privacy, data management, and consent, especially when interfacing closely with the nervous system.
In summary, neural prosthetics represent a fascinating convergence of engineering and neuroscience. The development and application of these devices showcase the potential benefits they offer, while also highlighting the limitations and challenges that need to be addressed to pave the way for greater success in restoring brain functionality.
Brain-Computer Interfaces: Innovations and Insights
Brain-computer interfaces (BCIs) represent a significant innovation within the merging realms of biomedical engineering and neuroscience. These systems establish a direct communication pathway between the human brain and external devices, allowing for unprecedented interaction with technology. This topic is crucial for understanding how engineering principles can be applied to enhance human capabilities. The benefits of BCIs can be numerous, ranging from rehabilitation solutions for individuals with neurological disorders to augmenting cognitive functions.
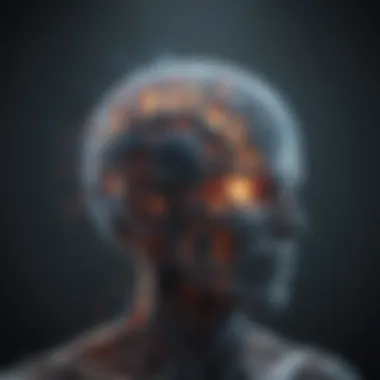
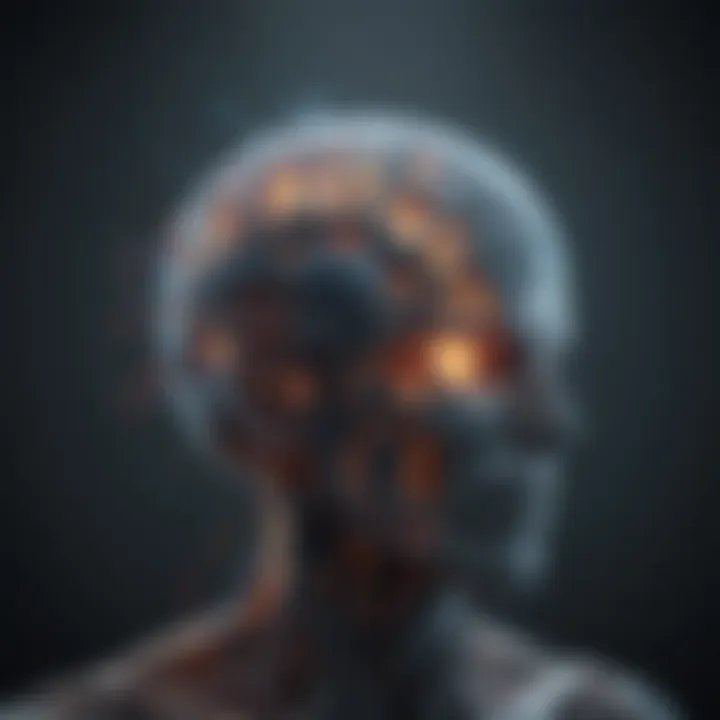
Conceptual Framework
The conceptual framework of BCIs involves understanding how brain signals can be captured and translated into actionable commands. At the core, BCIs rely on neural activity that can be monitored through various techniques such as electroencephalography (EEG), which records electrical activity along the scalp. Other methods include functional magnetic resonance imaging (fMRI) or invasive techniques that involve implantable devices.
Key aspects include:
- Signal Acquisition: Capturing neural signals using advanced sensors.
- Signal Processing: Analyzing and decoding brain signals to interpret user intentions.
- Device Control: Translating interpreted commands into device actions, such as moving a cursor on a computer screen.
Technical Fundamentals
Understanding the technical fundamentals of BCIs is essential for their development and application. BCIs operate on several critical components:
- Signal Acquisition: This is the first step in the process, where data from the brain is collected using various methods. EEG is the most common, but methods like implanted electrodes can provide more detailed readings.
- Data Processing: Once acquired, signals must be cleaned and processed to filter out noise. This often employs algorithms that enhance the signal quality.
- Pattern Recognition: Advanced algorithms are utilized to identify patterns in the processed signals that correlate with specific thoughts or intentions.
- Output Interface: This component translates the recognized patterns into commands for external devices.
The integration of these technologies creates a system that can enable users to control devices through thought alone. This development could significantly transform how rehabilitation and assistive technologies approach treatment.
Ethical Considerations in BCIs
The rise of BCIs brings along a host of ethical considerations that need to be addressed. Particularly, three key areas require careful scrutiny:
- Informed Consent: Individuals undergoing BCI implementations must fully understand the risks and benefits. Clear communication regarding what the technology can and cannot do is critical.
- Privacy: The potential for monitoring and interpreting brain activity raises significant concerns regarding privacy. Safeguarding mental data becomes paramount to prevent misuse by outside entities.
- Social Implications: As BCIs become more widespread, there are concerns about enhancing cognitive functions leading to societal divides. Access to such technologies could become a privilege rather than a right, creating new forms of inequality.
"Brain-computer interfaces stand at the forefront of merging neuroscience and technology, yet they also challenge our notions of privacy and ethics."
Understanding these concerns will be essential for future development and public acceptance of BCIs. As the field progresses, addressing these ethical implications ensures that innovations can be beneficial while respecting individual rights.
Advancements in Neuroimaging Technologies
Advancements in neuroimaging technologies play a crucial role in expanding our understanding of the human brain and enhancing clinical practices. These technologies not only provide insights into brain structure and function but also facilitate the development of new therapies for neurological disorders. This section will delve into the techniques and tools, applications in research and diagnostics, and future prospects associated with neuroimaging.
Techniques and Tools
Neuroimaging encompasses a variety of techniques, each with its unique strengths and applications. Among the most prominent methods are MRI, fMRI, PET, and CT scans.
- Magnetic Resonance Imaging (MRI): Utilizes strong magnetic fields and radio waves to generate detailed images of the brain's anatomy. It is crucial for detecting structural abnormalities.
- Functional Magnetic Resonance Imaging (fMRI): Extends traditional MRI by measuring brain activity through blood flow changes. This technique allows researchers to observe brain functions in real-time.
- Positron Emission Tomography (PET): Involves injecting a tracer to visualize metabolic processes in the brain. It is useful for diagnosing conditions like tumors or Alzheimer’s disease.
- Computed Tomography (CT): Combines X-ray images taken from different angles to create cross-sectional views of the brain. It is often used in emergency settings due to its rapid application.
These tools are essential for studying neural connectivity and understanding the complex workings of the brain.
Applications in Research and Diagnostics
Neuroimaging significantly facilitates research and diagnostics, impacting various fields including psychology, neurology, and psychiatry. Some notable applications include:
- Early Diagnosis: Neuroimaging aids in the early diagnosis of conditions such as multiple sclerosis, epilepsy, and stroke, enabling timely interventions.
- Researching Brain Disorders: It helps researchers explore the underlying mechanisms of disorders like depression or schizophrenia, allowing them to identify biomarkers for these conditions.
- Therapeutic Monitoring: Neuroimaging is valuable for assessing the effectiveness of treatments, such as monitoring changes in brain connectivity during therapy for anxiety disorders.
By enhancing the diagnostic landscape, these techniques improve patient outcomes and drive bedside-to-bench research.
Future Prospects in Neuroimaging
The future of neuroimaging technologies promises to be transformative. Several emerging trends and innovations suggest that we are on the precipice of significant advancements:
- Integration with Artificial Intelligence: AI algorithms can enhance image analysis, reduce interpretation errors, and provide more refined data interpretations, thus speeding up research.
- Portable Devices: Development of portable neuroimaging technologies will allow for assessments in non-clinical settings, improving accessibility.
- Personalized Medicine: Advancements will enable more tailored treatment plans, as neuroimaging provides insights into individual patients’ brain functions and responses.
An increased focus on ethical considerations and patient privacy will accompany these advancements, ensuring that benefits are maximized while minimizing potential risks.
"Neuroimaging technologies are not just tools—they are gateways to understanding the brain's complexity and treating its disorders."
In summary, advancements in neuroimaging technologies are integral to both research and clinical practice. They enhance our understanding of neural processes, refine diagnostic capabilities, and ignite future innovations in healthcare.
Impact on Cognitive Neuroscience
Understanding Brain Function through Engineering
Biomedical engineering contributes significantly to our understanding of brain functions. Through various techniques, such as neuroimaging and electrophysiology, researchers can observe brain activities in real time. Various neuroimaging tools like functional Magnetic Resonance Imaging (fMRI) allow scientists to visualize blood flow and oxygen consumption in active brain regions, offering insights into cognitive tasks, emotions, and decision-making processes. By employing engineering principles, we can develop more sophisticated models of brain activity. This innovative geometry supports more accurate predictions about cognitive behaviors tied to specific neural pathways.
Behavioral Interventions
Another important aspect is the application of engineering in behavioral interventions. Tools such as neurofeedback systems are emerging as ways to train individuals to regulate their brain activity. These interventions are promising in scenarios like anxiety management or attention regulation. For instance, in conditions like Attention Deficit Hyperactivity Disorder (ADHD), these approaches have shown potential to enhance focus by teaching patients how to modulate their neural responses.
Additionally, behavioral therapies may be enhanced through real-time feedback garnered from brain data. The ability to quantify cognitive states allows for more tailored strategies, improving how interventions engage with individual patient needs.
Long-Term Implications for Mental Health
The integration of psychiatric care and biomedical engineering carries significant long-term implications for mental health. Personalized treatment plans are becoming a reality, as data from brain imaging and behavioral responses inform practitioners about individual patient profiles. This information can lead to the customization of therapy and medication, ideally improving outcomes.
However, one must remain cognizant of the ethical considerations involved. Privacy concerns regarding sensitive neural data are paramount. How data is handled affects not only patient trust but also the broader societal implications of biomedical advancements in mental health.
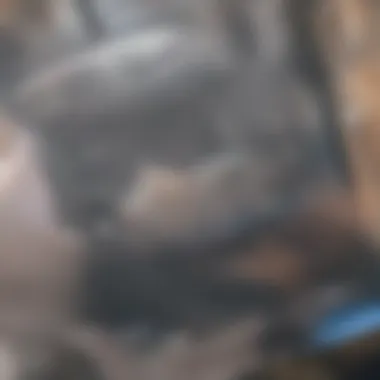
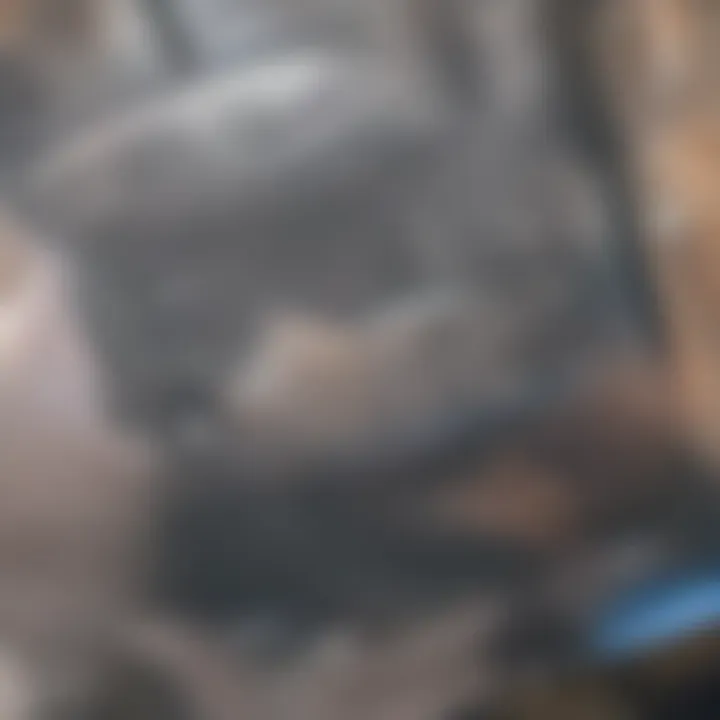
The potential is tremendous, as the merging of these disciplines promises not just enhanced understanding but innovation in treatments and interventions. The journey, however, continues to evolve, demanding ongoing dialogue among stakeholders in engineering, neuroscience, ethics, and mental health.
Collaborative Research Initiatives
Interdisciplinary Approach to Challenges
The interdisciplinary approach is essential for solving multifaceted problems in healthcare. By integrating knowledge and techniques from both biomedical engineering and neuroscience, researchers can develop comprehensive solutions. For instance, when tackling issues related to neural prosthetics, interdisciplinary teams can optimize device functionality while considering the intricacies of brain function. This melding of disciplines allows for the creation of smarter, more effective medical devices.
Moreover, this collaboration often leads to improved research methodologies. Techniques from engineering, such as data analysis and modeling, can enhance neuroscience studies. Similarly, neuroscience insights can guide the design of engineering solutions tailored to brain function.
Research Institutions Leading the Way
Many prominent research institutions are paving the way for collaboration between biomedical engineering and neuroscience. For example, the Massachusetts Institute of Technology (MIT) has established cross-disciplinary labs that focus on neuroengineering. Such institutions foster environments where researchers can work together on innovative projects, from developing BCIs to advanced imaging techniques.
In addition, universities often enter partnerships with hospitals and tech companies. This further enhances collaborative efforts by combining academic research with practical applications. This collective expertise accelerates the pace of advancements, translating theoretical knowledge into real-world clinical solutions.
Funding and Support for Innovations
Funding plays a vital role in supporting collaborative research initiatives. Without sufficient financial support, many innovative projects may never get off the ground. Agencies like the National Institutes of Health (NIH) and private foundations provide grants specifically aimed at interdisciplinary research, recognizing its importance.
Additionally, some firms and venture capitalists are increasingly interested in funding projects at the intersection of neuroscience and biomedical engineering. They understand that breakthroughs in this area can lead to significant advancements in patient care. Their investment can facilitate the development and testing of new technologies.
Support from these avenues not only provides monetary resources but also offers institutional backing, enhancing the credibility of research projects.
By combining the efforts of various stakeholders, the field continues to grow, demonstrating the power of collaboration in pushing the boundaries of what is possible in neuroscience and engineering.
"Collaborative research is not just a trend; it's a necessity for modern science to thrive."
Ethical Implications of Merging Disciplines
The integration of biomedical engineering and neuroscience raises numerous ethical concerns that require thoughtful consideration. As these two fields increasingly collide, the significance of understanding ethical implications cannot be overstated. This discussion is vital for ensuring responsible practices, protecting individual rights, and promoting the integrity of scientific research. The intersection of engineering and neuroscience has given rise to innovative technologies, but these applications bring not only benefits but also challenges that need addressing.
Informed Consent in Neuroengineering
Informed consent remains a cornerstone of ethical research and practice. Within neuroengineering, patients often interact with complex technologies that directly influence brain functions. Therefore, it is imperative to ensure that individuals fully comprehend what these interventions entail. This includes understanding potential risks, benefits, and the extent of data collection by devices. Researchers and practitioners must communicate clearly and effectively, avoiding overly technical language that might confuse patients. Building trust is critical.
For instance, if a neural implant can enhance memory but poses risks, the individual should be able to weigh these factors. Transparency about the nature of the technology and its potential consequences is not just advisable but necessary.
Privacy Concerns in Neural Data Usage
As neuroengineering evolves, the collection and storage of neural data have raised severe privacy issues. Neurotechnological devices can gather sensitive information about a person’s thoughts and feelings, making it crucial to establish strong protection measures. There is a risk that unauthorized access to this data could lead to misuse, identity theft, or discrimination.
It is essential for developers and users of neural technologies to implement strict data protection protocols. Confidentiality and security must be prioritized to safeguard individual privacy rights. Proper regulations must be set in place to ensure that data is used solely for intended purposes in a way that respects users' autonomy and dignity.
Social Implications of Neurotechnologies
The societal impact of merging neuroscience and biomedical engineering extends beyond individual privacy and consent. With the rise of advanced neurotechnologies, there are concerns about inequality and access. Not everyone may afford or have access to these innovations, leading to a divide in healthcare quality.
Furthermore, the potential for cognitive enhancements raises issues of fairness and ethical boundaries. If some individuals can improve their cognitive abilities through technology, will this result in societal pressures to conform or enhance? These questions are not easy to answer.
"As we advance, we must remain vigilant about the social fabric that holds us together. Navigating these fields needs dialogue among engineers, neuroscientists, ethicists, and society at large."
Future Directions in Biomedical Engineering and Neuroscience
The integration of biomedical engineering and neuroscience presents numerous avenues for future exploration. Understanding how we can drive advancements in these fields is critical for both medical and technological sciences. Emerging technologies hold the promise of transforming diagnostics and treatment options, thus improving patient outcomes.
The significance of future directions in this intersection cannot be overstated. Advancements could lead to breakthroughs in treatment for neurological disorders, brain injuries, and more. As the fields evolve, it becomes essential to monitor specific trends and innovations that can pave the way for improved therapies and devices.
Emerging Technologies to Watch
Several technologies are on the forefront of this revolution. Artificial intelligence (AI) is one of them. AI has potential to greatly enhance neuroimaging analysis, making it more precise and efficient. This can result in better assessments and targeted interventions.
Another area to keep an eye on is 3D printing. This technology might change how prosthetics are designed and manufactured. Custom-made devices can ensure better fit and functionality for individual patients, leading to improved rehabilitation outcomes.
- Wearable devices are also gaining traction. They can monitor neural activity and other physiological metrics in real time, offering insights that can lead to personalized treatments.
- Gene therapy technologies might allow for a more targeted approach in tackling genetic disorders affecting neural pathways.
- Lastly, developments in microelectronic devices are enabling more effective brain-computer interfaces, bridging the gap between physical devices and neural signals.
Potential for Personalized Medicine
Personalized medicine is another crucial area. With the combination of biomedical engineering insights and neuroscientific research, the potential to tailor treatments to the individual is growing. Each patient’s unique genetic makeup and neural structure can be considered. This leads to targeted therapies that are more effective and have fewer side effects.
For example, by understanding the neural responses to certain drugs, physicians can prescribe medications that are optimized for individual reactions. This tailored approach is especially promising for those suffering from conditions such as depression or epilepsy. As technologies for analysis and treatment advance, the ability to craft individualized strategies becomes more feasible.
Closing Thoughts on Interdisciplinary Innovation
As we look to the future, collaboration between disciplines will be essential. The merging of biomedical engineering and neuroscience is not merely a trend; it’s a movement toward more innovative and effective healthcare solutions.
"The future of healthcare lies at the intersection of engineering and biological sciences."
Interdisciplinary approaches can lead to comprehensive solutions that address complex medical challenges. Support from academic institutions, industries, and non-profit organizations will be vital. Funding and resources should focus on collaborative research initiatives that explore this convergence.
Moreover, ongoing discussions about the ethical implications of these technologies should not be overshadowed. Understanding and addressing issues like informed consent and privacy will be key to ensuring that technological advancements benefit society as a whole.
The future promises a landscape rich with possibilities in biomedical engineering and neuroscience. A commitment to collaboration and innovation is necessary to realize these benefits effectively.