The Role of Carbon Metal in Modern Science and Industry
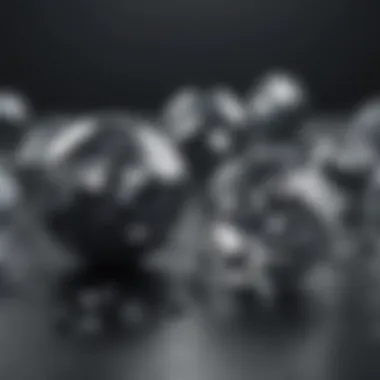
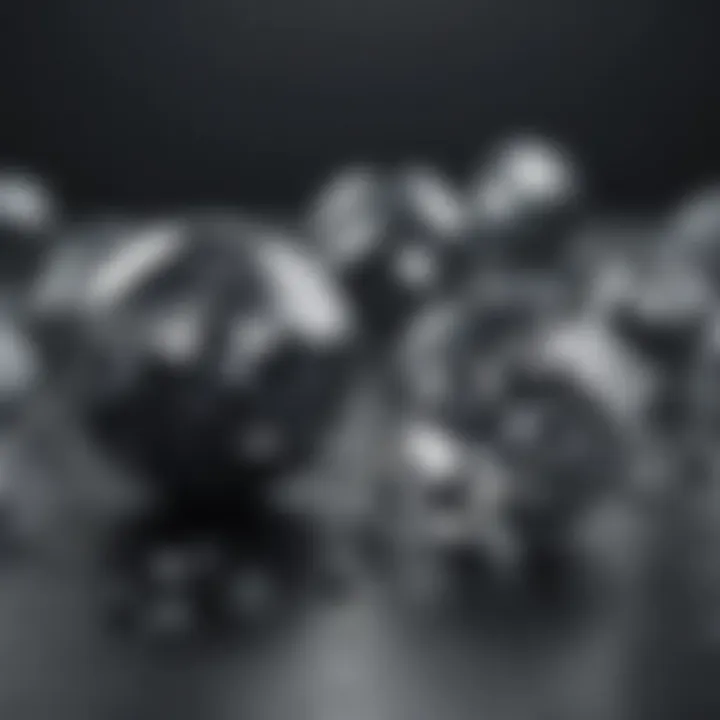
Intro
Carbon is everywhere, from the air we breathe to the diamonds you might unearth. In science and industry, its significance cannot be overstated. This article investigates the multifaceted world of carbon metal, also known as carbon allotropes, focusing on properties like strength, structure, and various applications.
One of the most intriguing aspects of carbon is its ability to exist in unique forms like graphite, diamond, and graphene. Each of these structures exhibits different behaviors and applications, making carbon a versatile element worthy of study and exploration.
Through the exploration of this material, we aim to shed light on its vital role in innovation and advancement across numerous fields. With a diverse audience in mind—students eager to learn, professionals in the field, and enthusiasts curious about contemporary science—we strive to deliver insights that are both enlightening and substantial.
Foreword to Carbon Metal
Understanding carbon metal is key to unlocking a treasure trove of scientific and industrial advancements. This subsection provides insight into carbon as a material, emphasizing its multifaceted nature and crucial role in today's high-tech landscape.
Definition and Composition
Carbon metal, often referred to as carbon in its metallic state or as metal-like carbon, represents a fascinating branch of chemistry. At its core, carbon is one of the most versatile elements known to humanity. This means it can arrange itself into different atomic structures, leading to various allotropes like graphite and diamond.
The composition of carbon metal is quite intriguing. It primarily consists of pure carbon atoms, which can bond in several configurations. The covalent bonding exhibited in diamond results in an incredibly hard substance, while the planar sheets of graphite allow layers to slip over each other, making it a good lubricant. Each of these forms offers unique properties that make carbon invaluable across numerous applications.
Historical Context and Discovery
The journey of carbon's discovery dates back to ancient times, yet its industrial significance burgeoned only in the last couple of centuries. The historical narrative is rich and nuanced—carbon was originally identified by early chemists as black soot from burning organic materials. However, it wasn’t until the late 18th century that chemists like Antoine Lavoisier began to classify carbon as an element, recognizing its crucial role in the organic composition.
The Industrial Revolution acted as a catalyst for deeper exploration into carbon’s possibilities. The invention of the Bessemer process in the 19th century, which converted pig iron into steel by blowing air through it, opened up new avenues accentuating carbon’s importance in metallurgy. With innovations such as carbon arc lamps and various carbon allotropes coming to light, researchers began to harness its potentials for technological prowess.
As science progressed, so did the interest in carbon-based nanomaterials in the late 20th century, leading to the discovery of carbon nanotubes and graphene—materials that promise revolutionary changes in electronics, materials science, and beyond.
The ongoing exploration and utilization of carbon in its myriad forms reflect an intricate connection between our historical quest for understanding and today’s relentless pursuit of innovation.
"From the ancient soot of our ancestors to the sophisticated nanomaterials of today, carbon metal has woven itself into the fabric of scientific discovery and technological evolution."
Physical Properties of Carbon Metal
The physical properties of carbon metal are the keystone of its relevance in contemporary science and industry. Understanding these properties helps in grasping how carbon can be adapted for diverse applications, from electronics to material sciences. The intrinsic characteristics of carbon allow it to shine brightly in its various forms. Let’s break down these properties into manageable sections for clearer understanding.
Structure and Bonding
The structure of carbon atoms, with their ability to form four covalent bonds, is crucial. This bonding capacity facilitates a variety of configurations, leading to the creation of different allotropes like graphite, diamond, and graphene. The way carbon atoms connect affects the material’s strength, chemical behavior, and interactions with other materials. As for the bonding angles and types, they directly influence how carbon behaves under stress and high temperatures.
The robustness of these bonds accounts for carbon's extensive versatility. For instance, the layered structure of graphite contrasts sharply with the tetrahedral arrangement found in diamond, leading to significant differences in physical characteristics.
Mechanical Characteristics
Strength
Strength is perhaps the most prominent feature when discussing carbon metal. Its tensile strength, particularly in forms such as carbon fiber, is remarkable. This high strength-to-weight ratio is a boon in various applications, particularly aerospace and automotive industries. Carbon's resistance to deformation under stress means structures can be designed lighter yet endure significant stress, enhancing durability without the trade-off of weight.
One of the unique features of carbon’s strength is its elasticity in materials like graphene. While maintaining high strength, graphene provides flexibility which opens doors for innovative uses in technology and other fields. However, such strength can lead to brittleness in certain configurations, necessitating careful consideration in application.
Elasticity
Elasticity, or the ability of a material to return to its original shape after deformation, is another crucial mechanical characteristic. Carbon's elastic properties allow it to absorb shocks without permanent damage. This factor plays a significant role in applications like sporting goods, where resilience is needed.
The unique feature of elasticity in different forms of carbon allows for creative designs in products like flexible electronics or even wearable technologies. However, too much flexibility might compromise the overall structural integrity in some cases, which needs careful engineering.
Toughness
Toughness, representing a material's ability to absorb energy and plastically deform without fracturing, is essential for many applications, particularly in the field of bomb-proof materials and protective gear.
Carbon metal showcases toughness in composites where it's combined with other materials, creating products that resist impacts, an important characteristic in safety equipment or construction. The unique feature of carbon toughened with polymers leads to materials that struggle to break. On the flip side, certain applications may not benefit from excessive toughness, as they may require a balance between toughness and softness for optimal performance.
Thermal and Electrical Conductivity
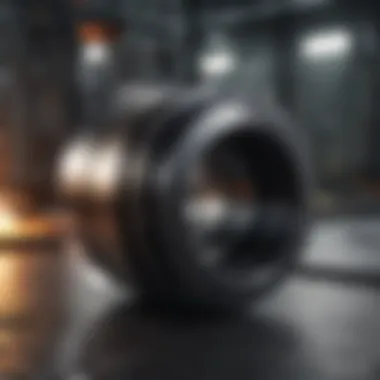
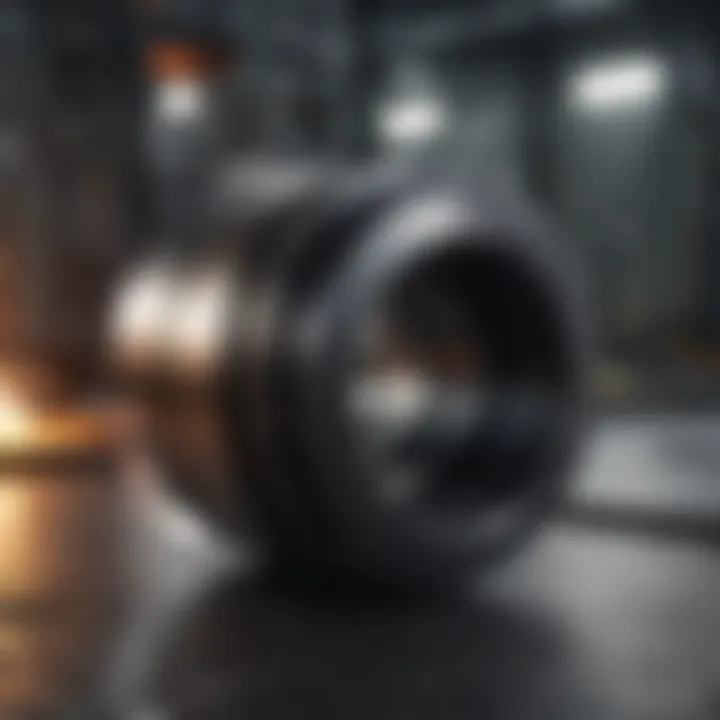
Carbon's thermal conductivity varies widely among its forms. For instance, graphite is an excellent conductor of heat while diamond is an insulator. This variability makes carbon invaluable for thermal management in high-tech equipment. The ability to engineer materials that conduct heat effectively leads to innovations in electronics, where managing temperature is critically important.
When it comes to electrical conductivity, materials like graphene are at the forefront, displaying exceptional conductivity, which opens avenues in electronics and battery technologies. The ability of carbon's allotropes to carry electricity allows for the development of faster, more efficient electronic devices, which remain a hot topic in research and product development.
In summary, the physical properties of carbon metal are a vital cog in the machine of scientific and industrial advancements. These characteristics not only define its current applications but also provide a glimpse into its potential future role in material sciences.
Forms of Carbon Metal
Carbon metal exists in several distinct forms, each with unique properties that pose different implications for science and industry. Understanding these forms is vital since they dictate how carbon can be utilized across various applications—from electronics to materials science. These diverse forms include graphite, diamond, amorphous carbon, carbon nanotubes, and graphene. Each form offers specific benefits and challenges, making it crucial to explore their unique characteristics in detail.
Graphite
Graphite is one of the most well-known forms of carbon, commonly recognized for its use in pencils and lubricants. Its structure consists of stacked layers of graphene, which can slide over each other, providing graphite with its lubricating properties. This characteristic makes it valuable in the manufacturing of batteries, where it serves as an anode material. Furthermore, its ability to conduct electricity has spurred research into its potential applications in various electronic devices.
- Key apects of graphite include:
- Excellent thermal conductivity
- High electrical conductivity
- Resistance to oxidation
Graphite also has a significant role in energy storage, particularly in lithium-ion batteries. These batteries' efficiency can be substantially improved by incorporating high-quality graphite, highlighting its importance in today’s tech-driven society.
Diamond
Diamond, while often associated with luxury and jewelry, holds immense value in scientific and industrial settings due to its unparalleled hardness and optical clarity. Its rigid lattice structure contributes to its incredible strength, making it an essential component in cutting tools, drills, and abrasives. Moreover, diamonds have unique optical properties, leading to their application in high-performance optoelectronic devices.
- Noteworthy properties of diamond include:
- Exceptional thermal conductivity
- Impressive mechanical strength
- Transparency in a wide spectrum of wavelengths
The use of synthetic diamonds is on the rise. These artificially created gems serve not just in high-end goods but also in advanced technological applications, pushing the boundaries of what we can achieve with materials science.
Amorphous Carbon
Amorphous carbon presents a fascinating departure from the structured forms of graphite and diamond. Lacking a definite crystalline structure, this form is less ordered, making its properties highly variable. Popular in many applications, such as protective coatings, and as filler materials in plastics, amorphous carbon is often used in electronics for thin films and transistors. Its versatility is rooted in its ability to be tailored for specific applications.
- Characteristics of amorphous carbon include:
- variable hardness
- customizable electrical conductivity
This adaptability allows for the development of advanced materials that harness the unique properties of this carbon form, enabling innovation in sectors like nanotechnology and surface protection.
Carbon Nanotubes
Carbon nanotubes are cylindrical structures that are a marvel of modern materials science. They possess extraordinary mechanical strength—much stronger than steel—alongside exceptional electrical and thermal conductivity. Their tube-like formation allows for high tensile strength while maintaining a lightweight profile, invaluable in aerospace and automotive industries.
- Highlights of carbon nanotubes include:
- High aspect ratio
- Exceptional strength-to-weight ratio
- Good electrical conductivity
These qualities render carbon nanotubes suitable for applications ranging from nanoelectronics to composite materials. As the research in this area progresses, their impact on technology continues to grow.
Graphene
Often hailed as a revolutionary material, graphene is a single layer of carbon atoms arranged in a two-dimensional honeycomb lattice. Its remarkable strength and flexibility, combined with outstanding electrical and thermal conductivity, set it apart from other carbon forms. Researchers are excited about graphene's potential in various fields:
- Key potentials of graphene include:
- Next-generation electronics
- Advanced materials and nanocomposites
- Energy storage applications
The future of graphene research is promising. As scientists delve deeper into its properties, new applications are likely to surface, cementing its place in the advancing landscape of technology and materials science.
"Graphene is significantly influencing advancements across a multitude of fields, providing a new lens through which we can contemplate materials science and engineering."
In a nutshell, the forms of carbon metal play a critical role in shaping the landscape of contemporary science and industry. Each form comes with unique traits that cater to specific needs, illustrating carbon's versatility and importance in our evolving technological landscape.
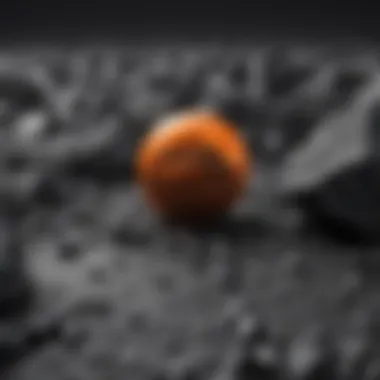
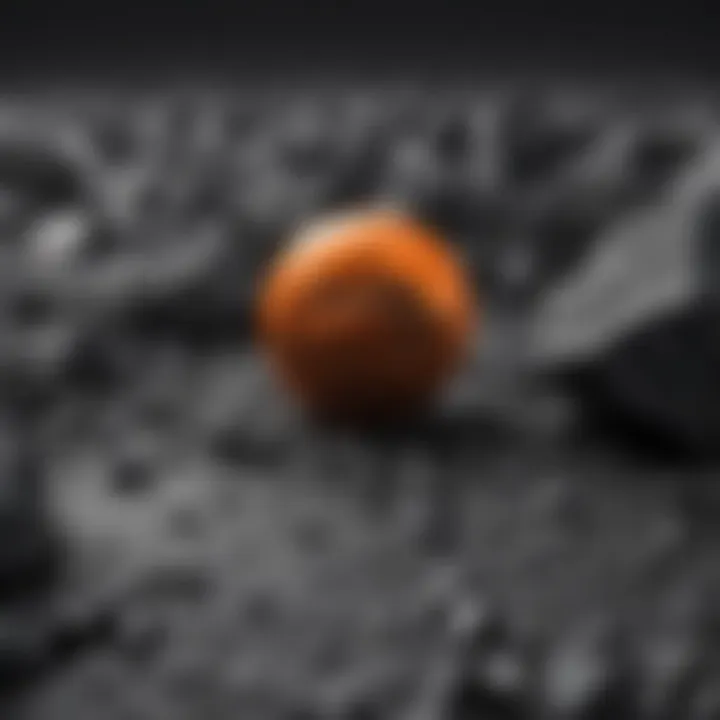
Production and Synthesis Techniques
The production and synthesis of carbon metal is crucial in understanding its vast applications across various fields. The techniques employed not only determine the availability of carbon in its different forms but also influence its properties, which can be tailored for specific applications. Whether through natural processes or advanced industrial methods, the synthesis approaches impact everything from the strength of materials to their conductivity. Let’s delve deeper into the two main categories: natural processes and industrial manufacturing techniques.
Natural Processes
Natural processes of carbon production primarily involve geological and biological means. Over millions of years, the accumulation of organic matter results in the creation of coal and other forms of carbonaceous materials. These processes demonstrate how carbon is sequestered in the Earth’s crust, leading to valuable resources. Such formations can yield energy and serve as a raw material for various products. However, their extraction and use also tie into environmental concerns, making sustainable methods essential.
Industrial Manufacturing Techniques
The industrial segment offers a plethora of methods for synthesizing carbon metal, significantly enhancing its availability and versatility. Among these, three techniques stand out:
Chemical Vapor Deposition
Chemical Vapor Deposition (CVD) is a fundamental method widely regarded in the industry. It allows for the creation of thin films and intricate structures with precision. Essentially, carbon-containing gases react on a substrate, leading to a thin layer of carbon being deposited. This is particularly valuable for applications in electronics and nanotechnology. One key characteristic of CVD is its ability to produce high-purity materials, making it a reliable choice for high-performance applications.
However, while the merits are substantial, CVD does come with its cons. The equipment required can be expensive, and the process also often requires strict control over temperature and pressure. Overall, though, the precision and versatility of CVD make it a favored technique in today's manufacturing landscape.
Mechanical Exfoliation
Mechanical Exfoliation, on the other hand, brings a different flavor to carbon production. This technique involves physically peeling layers off materials like graphite. This method has gained traction for producing graphene—one of the strongest materials known—due to its unique properties. The simplicity of the method is a major boon, as it can be done with basic materials and equipment, making it accessible.
Yet, Mechanical Exfoliation is not without its challenges. The yield often varies and the quality can be inconsistent compared to more controlled methods. Still, its contribution to the field of materials science, especially in graphene production, marks it as a significant player in the carbon synthesis game.
High-Pressure High-Temperature Synthesis
Lastly, we can’t overlook High-Pressure High-Temperature (HPHT) Synthesis. This technique mimics the natural conditions under which diamonds form, allowing for the artificial creation of diamonds in a lab setting. The main advantage of HPHT lies in its ability to produce high-quality diamonds quickly and in a controlled environment. This is essential for both industrial uses and gem-grade diamonds.
Conversely, the process is energy-intensive and requires specialized equipment, making it less suitable for large-scale production compared with other methods. Nevertheless, the unique properties of HPHT diamonds—especially their clarity and hardness—make this method vital in both the jewelry market and various technological applications.
Applications of Carbon Metal
Carbon metal has woven itself into the fabric of contemporary science and industry. Its versatility is not merely a function of its composition but indeed, arises from its multifaceted nature that allows it to find use in a wide array of applications. The exploration of these applications showcases how carbon metal has steadily become a linchpin across various technological domains, while also raising considerations about sustainability and environmental impact.
Electronics and Semiconductors
In the realm of electronics, carbon metal—especially in the form of graphene—has revolutionized the way we approach circuit design and semiconductor manufacturing. Its atomic structure provides high electrical conductivity, enabling faster and more efficient electronic devices. Devices utilizing graphene offer benefits, such as reduced overheating and better battery life, making them indispensable for smartphones and laptops.
Moreover, graphene’s mechanical strength vastly surpasses that of traditional materials. For instance, a graphene layer is incredibly thin, yet it holds extraordinary tensile strength. This blend of resilience and conductivity positions carbon metal as a pivotal player in designing next-generation electronic components. With ongoing research, its potential in flexible electronics is something to keep an eye on, as it can lead to innovative applications in wearable tech.
Energy Storage and Conversion
Energy storage has always been a concern in the pursuit of sustainable solutions. Carbon metal stands at the forefront of this quest. Its ability to store energy efficiently is accentuated in both batteries and supercapacitors.
Batteries
When it comes to batteries, carbon materials are essentially game changers. Lithium-ion batteries, which have become the standard in portable electronics, significantly incorporate carbon. Carbon nanofibers, for instance, serve to enhance the performance of these batteries. They contribute to a higher charge capacity and faster charging times. What makes them particularly attractive is their lightweight nature, crucial in reducing the overall weight of electronic devices and electric vehicles.
However, there are challenges too. As the demand for batteries grows, ensuring sustainable practices in mining materials is a key concern. The environmental footprint associated with the production of batteries can’t be ignored. Addressing this through recycling and responsible sourcing remains a priority.
Supercapacitors
Supercapacitors represent another vital application of carbon metal in energy storage. Their distinct characteristic is the ability to charge and discharge quickly, which is indispensable for applications requiring rapid bursts of energy. For example, equipment in electric vehicles and power tools greatly benefit from the quick-rechargeable nature of supercapacitors.
In contrast to batteries, supercapacitors offer a longer lifespan, obtaining high-cycle durability without degrading over time. Nonetheless, a major drawback is their generally lower energy density compared to traditional batteries. This can impact their readiness as a standalone solution for long-term energy supply.
Materials Science Innovations
The future of materials science is heavily intertwined with carbon metal applications. Novel composites that incorporate variations of carbon, like graphene or carbon nanotubes, open doors to enhancements in strength, weight, and versatility of materials. Structural frameworks in buildings or vehicles could see a reduction in weight without compromising strength, thus enhancing fuel efficiency and reducing resource consumption.
In areas like medicine, carbon-based materials are developing into biodegradable alternatives for drug delivery systems. This innovative approach shows promise in reducing the long-term environmental impact associated with medical materials. Ultimately, the integration of carbon into diverse fields signifies an evolutionary step in material development, pushing boundaries towards a more sustainable future.
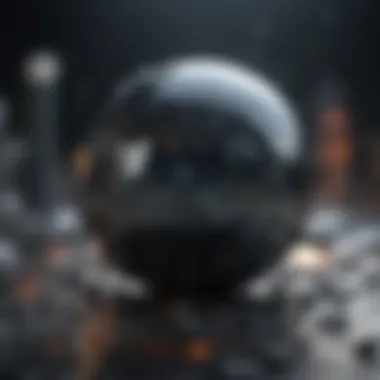
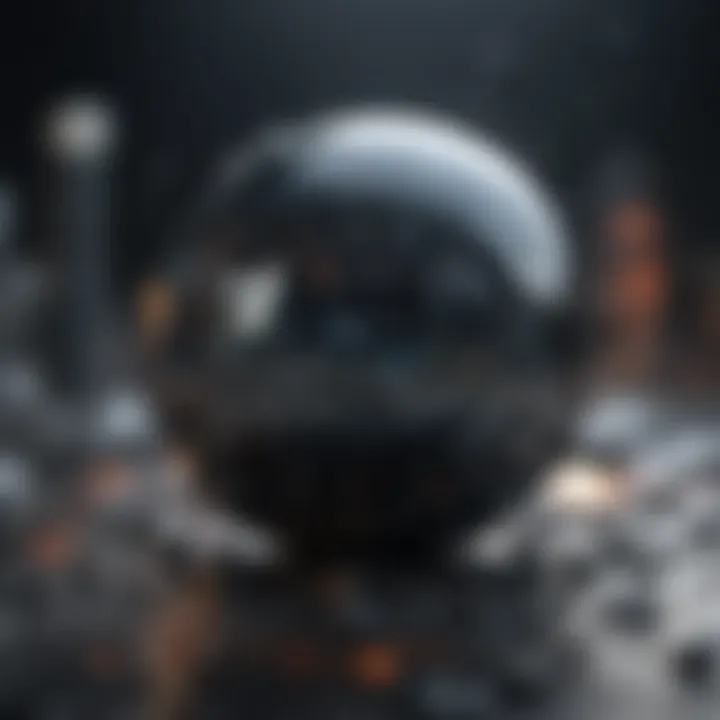
"Carbon metal is not just an element; it’s the backbone of technological advancement in our modern world."
In summary, the applications of carbon metal underline its essential role across various industries. As we continue to explore its potential, the balance between progress and sustainability will be a key narrative in the ongoing dialogue about its uses.
Environmental Impact and Sustainability
The relevance of environmental considerations in the context of carbon metal cannot be overstated. As the global community increasingly grapples with the consequences of industrial actions on the environment, the sustainability practices linked to carbon metal production and utilization are becoming focal points. Understanding the environmental impact of carbon metal is not just about recognizing its effects; it’s about exploring pathways to mitigate those impacts and emphasizing sustainable innovation.
Carbon Footprint of Production
The journey of carbon metal from extraction to application is labyrinthine, often associated with significant carbon emissions. The production process—whether it’s the mining of graphite or the synthesis of graphene—demands substantial energy and can lead to a considerable carbon footprint.
- Energy Consumption: Major production methods require large amounts of power, predominantly sourced from fossil fuels. This reality increases the greenhouse gas emissions associated with carbon metal, making energy efficiency a critical need in this sector.
- Pollutants: Extraction and processing can release particulate matter and other pollutants into the environment. This pollution affects not only the immediate area but can also have far-reaching consequences for air and water quality.
Mitigating the Footprint
Acknowledging the carbon footprint entails proactivity in research and policy. Efforts are underway to develop more sustainable extraction processes, such as:
- Utilizing renewable energy sources in manufacturing.
- Increasing the efficiency of existing processes to lower energy consumption.
"Carbon metal’s environmental journey is intertwined with technological advancements, and as awareness grows, so does the potential for transformative practices."
This understanding is vital not just for companies looking to improve their sustainability credentials but also for researchers focused on developing alternative materials with lower environmental impacts.
Recycling and Life Cycle Analysis
The recycling aspect of carbon metal introduces another layer of sustainability. Materials like graphite can be reused, leading to significant energy savings and a reduction in the need for raw material extraction. Here's how recycling contributes:
- Material Reuse: Various forms of carbon, particularly graphite, can be collected and processed. This reduces the demand for fresh resources, echoing a shift toward circular economy principles.
- Lifecycle Considerations: Conducting life cycle analyses enables industries to assess the environmental impacts of carbon metal from cradle to grave. Understanding the total impact highlights areas for improvement and encourages the adoption of better practices throughout the production chain, from resource extraction to product design that facilitates end-of-life recycling.
Future Directions in Research
As we look ahead, the significance of carbon metal in advancing the fields of science and industry becomes increasingly evident. The study of carbon, particularly its diverse allotropes and compounds, is poised to unlock new realms of innovation. With a keen eye on emerging technologies and advancements in nanotechnology, researchers are setting the stage for transformative changes across numerous sectors. This section delves into these directions, spotlighting their relevance and the multitude of benefits they present.
Emerging Technologies
The focus on emerging technologies involving carbon is turning heads in various fields. From augmented reality to energy solutions, carbon metal is stepping into the limelight. For instance, the integration of graphene-based components in electronics shows tremendous promise. Graphene not only enhances the conductivity of devices but also reduces their weight and energy consumption. This can lead to more efficient gadgets, making lives smoother and greener.
Some key areas where emerging technologies are making strides include:
- Wearable Technology: Carbon fibers are revolutionizing fitness devices, enhancing flexibility and durability.
- Biomedical Applications: Carbon nanomaterials in drug delivery systems are enhancing the efficacy of treatments by enabling targeted delivery.
- Aerospace Engineering: Strong yet lightweight carbon composites are also opening doors for advancements in airplane design, increasing fuel efficiency.
The potential of carbon in these technologies is profound and expanding. Continued research could lead to breakthroughs we have yet to dream of.
Nanotechnology and Beyond
Nanotechnology represents a frontier in research that leverages the remarkable properties of carbon at the atomic scale. The manipulation of carbon atoms enables the creation of materials that can heal themselves or adapt to their environments. This goes beyond mere theoretical concepts; practical applications are emerging in the world of medicine and electronics. For example, nanoscale carbon materials are being explored for targeted cancer therapies, promising better patient outcomes.
Some considerations for future research in nanotechnology include:
- Scalability: Ensuring that the production of nanoscale carbon materials is cost-effective and sustainable to meet industrial demands.
- Safety and Regulation: Assessing the environmental and health implications associated with the use of nanoscale materials is critical for responsible innovation.
- Interdisciplinary Approaches: Collaboration between chemists, physicists, and engineers will be essential in establishing comprehensive frameworks for utilizing nanotechnology.
"The future of carbon research stands at the brink of groundbreaking discoveries, influencing everything from energy solutions to healthcare innovations."
End
Summary of Key Points
- Diverse Forms: Carbon metal appears in several forms such as graphite, diamond, and graphene, each with distinct attributes suited to specific applications.
- Production Methods: Understanding the synthesis techniques, whether through natural occurrence or industrial manufacture, reveals how carbon can be tailored for different uses.
- Wide Applications: From energy storage solutions to materials science innovations, carbon metal serves as a foundation for groundbreaking advancements.
- Environmental Considerations: The carbon footprint associated with its production and the significance of recycling are imperative for sustainable development.
- Future Directions: Emerging technologies in nanotechnology and novel applications suggest a continual evolution in the utilization of carbon metal.
The Role of Carbon Metal in the Future
Looking ahead, the role of carbon metal seems poised for expansion. Innovative technologies such as quantum computing may leverage the unique properties of carbon allotropes for enhanced performance. Additionally,
"The integration of carbon-based materials in renewable energy systems could drive significant improvements in efficiency and sustainability."
Emerging research is uncovering new ways to exploit carbon nanotubes in various fields, including medicine and environmental science, making it a focal point for future research. As battery technology advances, carbon metal will likely play a crucial part in optimizing energy storage systems, ensuring that we can meet the demands of an increasingly energy-hungry world.
In summary, carbon metal is not merely a material of interest; it is a cornerstone for future innovations across countless domains, underpinning sustainable practices and technological advancements.