Exploring CAMB: Insights into Cosmology and Structure
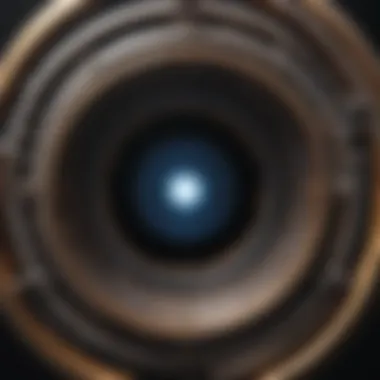
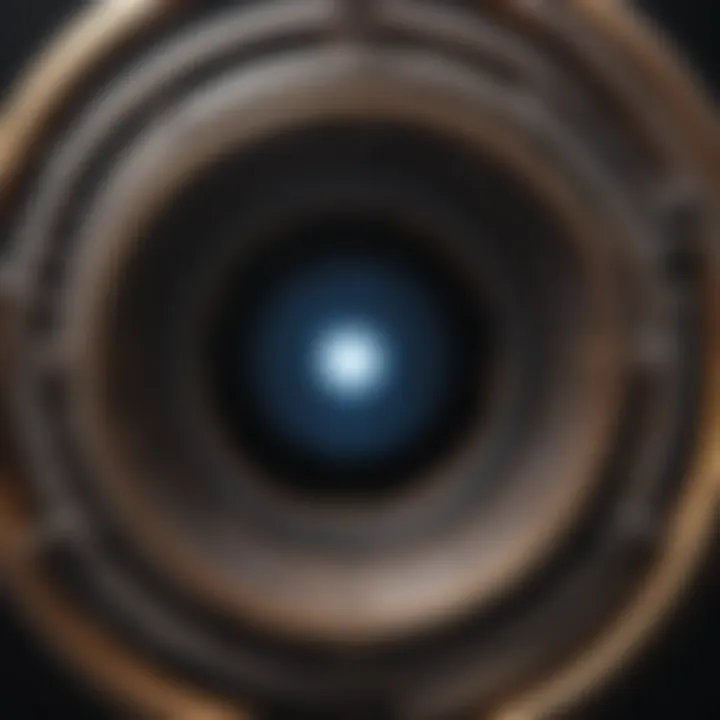
Intro
The world of cosmology is intricate and multifaceted, populated with various models and theoretical frameworks. One of the pivotal tools within this domain is the CAMB model, or Code for Anisotropies in the Microwave Background. Understanding CAMB requires a grasp of both its theoretical foundations and practical applications. As modern astrophysics grapples with the universe's complexities, CAMB stands as a key player in simulating the cosmic microwave background and exploring large-scale structure formation.
In this article, we will unfold the intricacies of CAMB, focusing on its mechanisms and implications. Through this exploration, we aim to provide readers with a comprehensive understanding of how this model informs our views on cosmological phenomena, the academic relevance it holds, and its broader influence on scientific inquiry.
Research Overview
Summary of Key Findings
The CAMB model is primarily used to simulate the cosmic microwave background radiation. This radiation is crucial for understanding how the early universe has evolved over time. Some key findings include an in-depth understanding of the acoustic oscillations that shaped the CMB, the role of dark matter, and the expansion of the universe. By applying CAMB, researchers can produce predictions that align closely with observational data, confirming the model's reliability.
Background and Context
The theoretical foundation of CAMB stems from the physics of the early universe, particularly how quantum fluctuations led to the large-scale structures observed today. Understanding the origins of the cosmic microwave background is essential, as it serves as a reflection of the conditions prevalent shortly after the Big Bang. By analyzing temperature fluctuations and polarization patterns in the CMB, researchers gain insights into the universe's composition, age, and rate of expansion.
Methodology
Experimental Design
The application of the CAMB model involves both computational and observational components. Researchers leverage sophisticated algorithms to solve the complex equations governing cosmic evolution. This arrangement allows them to simulate various cosmological scenarios. For instance, they can examine the effects of different parameters, such as the density of baryonic matter and dark energy.
Data Collection Techniques
Data collection for CAMB simulations predominantly relies on observations from satellites and telescopes, such as the Planck satellite and the Wilkinson Microwave Anisotropy Probe. These instruments capture detailed datasets that inform the model, allowing analysts to refine their predictions continually. The reliability of the model is largely dependent on the accuracy of the initial conditions input into the simulations.
"The CAMB model is not merely a computational tool; it serves as a bridge between theoretical predictions and observational data."
Through the synthesis of these methodologies, the implications of CAMB reach far beyond academic interest. It reverberates into how we perceive and understand the universe's makeup and its underlying mechanics.
Foreword to CAMB
The introduction to CAMB sets the stage for comprehending its role within cosmology. It provides a foundational understanding necessary to grasp more complex concepts discussed in this article. By exploring what CAMB is and how it fits into the larger framework of cosmological studies, readers will appreciate its significance in simulating and analyzing cosmic phenomena. This understanding aids both newcomers and seasoned researchers in navigating the intricacies of the model and leveraging it for various astrophysical applications.
Definition of CAMB
CAMB stands for Code for Anisotropies in the Microwave Background. It is a computational tool used primarily in cosmology for simulating the Cosmic Microwave Background (CMB) and the large-scale structure of the universe. CAMB operates on mathematical principles derived from the standard model of cosmology, particularly the Friedmann-Lemaître-Robertson-Walker metric. The code enables researchers to predict the temperature fluctuations and polarization patterns in the CMB, which are critical for understanding the early universe and its subsequent evolution.
Historical Context
The development of CAMB can be traced back to the advancements in cosmology during the late 20th century. It emerged around the same time as the discovery of the cosmic microwave background radiation in the 1960s. Early cosmological models laid the groundwork, but it was the advancements in computational power and algorithms in the early 2000s that allowed for the effective implementation of CAMB. The code was created to provide a more precise toolkit for cosmologists working with data from missions like the Wilkinson Microwave Anisotropy Probe (WMAP) and the Planck satellite. Understanding this historical context is essential, as it highlights the evolution of cosmological tools and their relevance to empirical observations.
Importance in Cosmology
The importance of CAMB in cosmology cannot be overstated. The model serves several vital functions:
- Data Interpretation: CAMB helps interpret CMB data, which provides insights into the fundamental properties of the universe, such as its age, composition, and rate of expansion.
- Testing Cosmological Models: It allows scientists to test the predictions of different cosmological models against observations, contributing to debates about dark matter and dark energy.
- Framework for Further Research: As a robust tool, CAMB lays a framework for further research in cosmology. Studies involving galaxy formation, structure formation, and cosmic inflation often rely on the predictions made by this model.
Overall, understanding the introduction to CAMB is crucial for delving deeper into the mathematical framework, applications, and implications that follow in this article.
Mathematical Framework of CAMB
The mathematical framework of CAMB (Code for Anisotropies in the Microwave Background) is crucial for comprehending how the model operates. At its core, CAMB uses equations derived from general relativity and considers cosmological parameters such as the density of matter and energy in the universe. This framework not only delineates the relationships between different variables but also elucidates how these variables influence cosmological phenomena like the cosmic microwave background (CMB) and large-scale structures. Understanding this mathematical basis allows researchers to employ CAMB effectively in simulations and theoretical investigations, reinforcing its significance in cosmology.
Basic Equations Utilized
The foundation of CAMB rests upon several fundamental equations. These equations include the Friedmann equations, which describe the expansion of the universe. By expressing the evolution of the scale factor in terms of the density parameters, the model can account for the gravitational forces of various components, like normal matter, dark matter, and dark energy.
Another key equation is the Boltzmann equation, essential for describing photon interactions in the CMB. This equation models how photons are affected by the distribution of baryonic and dark matter in the early universe. These equations together allow CAMB to predict the temperature fluctuations and polarization patterns of the CMB.
Cosmological Parameters
Cosmological parameters define the universe's composition and behavior. CAMB requires several parameters to generate accurate predictions, including:
- Hubble constant (H0): This indicates the current rate of expansion of the universe.
- Density parameters ( ( \Omega_m, \Omega_b, \Omega_\Lambda )): They represent the fractions of matter, baryonic matter, and dark energy, respectively.
- Curvature ( ( K )): This reflects the geometry of the universe, whether it is flat, open, or closed.
These parameters are not just numbers; they shape the outcomes of simulations. Accurate parameterization helps in obtaining predictions that can be compared to observational data, making it an essential component of the CAMB framework.
Computational Techniques Applied
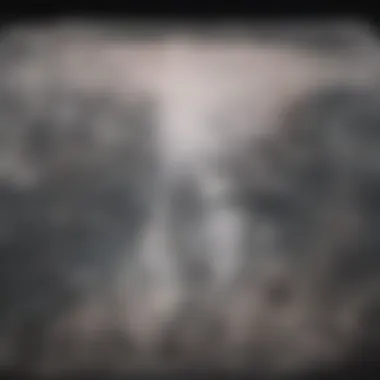
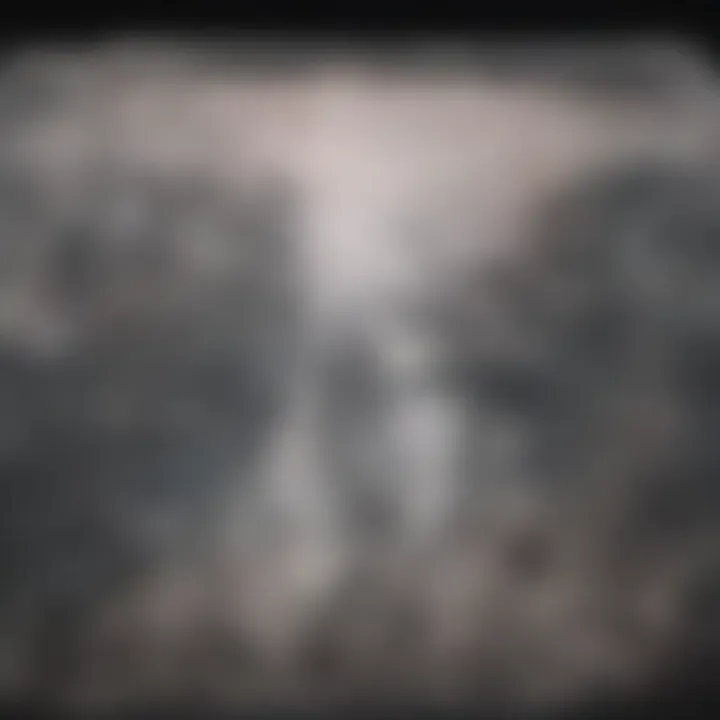
Computational techniques play an integral role in how CAMB functions. The software employs numerical methods to solve the governing equations iteratively. Techniques such as:
- Fast Fourier Transform (FFT): Used for calculating the CMB power spectrum
- Adaptive step-size algorithms: These facilitate the efficient resolution of equations across different scales
These methods allow for the handling of complex calculations that would otherwise be computationally prohibitive. The optimization of these algorithms has greatly enhanced CAMB's efficiency, enabling researchers to run simulations faster and gain insights into cosmological structures and signals. The seamless integration of these techniques highlights CAMB's role in advancing our understanding of the universe.
"The effectiveness of CAMB lies in its ability to translate complex physical phenomena into interpretable results through rigorous mathematical modeling."
In summary, the mathematical framework of CAMB is not merely a set of equations; it is the scaffolding that supports the significant inquiries into cosmology. Understanding the basic equations, parametrization, and computational methods provides a vivid picture of how CAMB contributes to our understanding of the universe.
Applications of CAMB in Research
The Applications of CAMB in Research are critical in the study of cosmology, as this model serves as a foundation for various investigations into cosmic structures and phenomena. The CAMB model, which stands for Code for Anisotropies in the Microwave Background, provides a rich framework for simulating data associated with the cosmic microwave background radiation. This ability to produce accurate predictions and simulations enables researchers to probe various significant areas of cosmology, ranging from the understanding of the early universe to the impact of dark matter and dark energy.
The integration of CAMB in research leads to several specific benefits. Firstly, it allows for the simulation of complex cosmic structures, giving insight into the universe's evolution over time. Secondly, it supports the evaluation of different cosmological parameters, enabling researchers to refine their models based on observational data. The intricate interplay of its applications leads to enhanced understanding and potentially groundbreaking discoveries in astrophysics.
Simulation of Cosmic Microwave Background
The simulation of the cosmic microwave background (CMB) is one of the most prominent applications of the CAMB model. The CMB is remnants of the early universe, essentially the afterglow of the Big Bang, and studying it provides vital information about the conditions of the universe at that time. CAMB allows researchers to create detailed models of the CMB anisotropies, which are minute temperature fluctuations evident in the CMB radiation.
Through the utilization of CAMB, researchers can effectively simulate how different cosmological parameters—such as density fluctuations or the Hubble constant—influence the CMB. This is essential for understanding how the universe expanded and cooled, allowing for predictions that can be tested against observational data from satellites like the Planck satellite. The accuracy of the simulations aids in confirming or refuting ongoing theories about the cosmos.
Modeling Large-scale Structure Formation
Another key application of CAMB is in the modeling of large-scale structure formation in the universe. Large-scale structures consist of galaxies, galaxy clusters, and the vast voids in between them, all of which developed due to gravitational forces acting over billions of years. CAMB helps scientists create simulations that map out how these structures form and evolve under various conditions.
By inputting different cosmological models into CAMB, researchers can study how varying factors, such as dark matter density or baryonic matter interactions, contribute to structure formation. The insights from these models play a pivotal role in understanding the dynamics of galactic formations and their distributions across the universe. Understanding large-scale structures opens pathways to better comprehend dark energy's effects and the universe's overall geometry.
Impact on Galaxy Formation Studies
The impact of CAMB is profound in the context of galaxy formation studies. This area of research seeks to understand how galaxies emerge and grow in their respective environments. CAMB's ability to simulate various scenarios related to cosmic evolution allows researchers to test hypotheses about the formation and behavior of galaxies.
In particular, the simulations derived from CAMB guide the exploration of environmental influences on galactic evolution. By modeling different celestial environments, researchers can evaluate how factors, such as density, temperature fluctuations, and metallicity affect galaxy formation. This aspect provides essential knowledge that complements observations from powerful telescopes, bridging a gap between theoretical predictions and empirical data.
"The applications of CAMB in research are pivotal in shaping our understanding of the cosmos and fostering discoveries that redefine our place within it."
Overall, the utility of CAMB in research is a cornerstone for advancing cosmological knowledge, addressing key questions about the universe's past, present, and future.
Technological Advances in CAMB
Technological advances in the CAMB model play a pivotal role in enhancing our understanding of cosmological phenomena. These advancements are not merely incremental; they significantly influence computation speed, model accuracy, and the capacity for complex simulations. In today’s rapidly evolving scientific landscape, leveraging technology is crucial for addressing the challenges of cosmological modeling. Each aspect of technological advancement can have far-reaching implications in our approach to studying the universe.
Computational Resources Required
The effective use of CAMB hinges on adequate computational resources. Modern simulations necessitate significant processing power and memory. High-performance computing clusters are often employed to manage the heavy calculations involved. The advent of multicore processors and graphical processing units (GPUs) has transformed the computational landscape. These technologies allow researchers to perform large-scale calculations more efficiently.
- Higher Processing Speeds: The availability of advanced processors increases the speed of calculations, allowing for larger datasets to be processed in shorter time frames.
- Parallel Computing: This technique enables multiple computations to occur simultaneously, further enhancing speed and efficiency.
- Cloud Computing: The shift towards cloud-based solutions has also enabled researchers to access computational power as needed, alleviating some of the burden of maintaining physical hardware.
Software Developments and Versions
The software that supports CAMB has gone through several iterations. Each version aims to improve functionality, usability, and efficiency. From user interface improvements to algorithm optimizations, these developments ensure that CAMB remains competitive in the field of cosmology.
Key updates include:
- User-friendly Interfaces: Enhancements in usability allow researchers with varying levels of technical expertise to navigate the software effectively.
- Algorithm Improvements: Frequents updates to the underlying algorithms enhance simulation accuracy and shorten computation times.
- Version Control: Maintaining a clear versioning system helps researchers track changes, allowing for better reproducibility in scientific studies.
Integration with Other Tools
The capacity for CAMB to interoperate with other analytical tools is vital for comprehensive cosmological research. Whether it is integrating with observational data analysis software or other simulations, this interoperability enhances CAMB's utility.
Some important integrations include:
- Connection to Data Analysis Tools: Tools like Python's NumPy and SciPy can be used alongside CAMB outputs for further statistical analysis and data manipulation.
- Compatibility with Simulation Software: Integrating CAMB with other simulation tools, such as those used for large-scale structure formation, provides a broad understanding of cosmological data.
- Database Connections: Linking with databases enables the efficient retrieval and storage of simulation results, making data management more straightforward.
"Technological advancements enhance the capabilities of CAMB, making it an indispensable tool in modern cosmological research."
Comparative Analysis of CAMB with Other Models
In the field of cosmology, understanding different models is crucial. The comparison of CAMB with other computational frameworks provides insights into its efficiency and applicability. This section highlights the basic tenets of CAMB, contrasting it with other models and indicating the specific scenarios where each model excels.
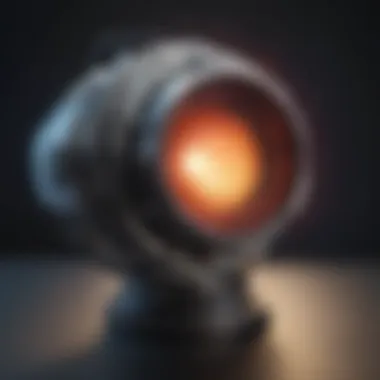
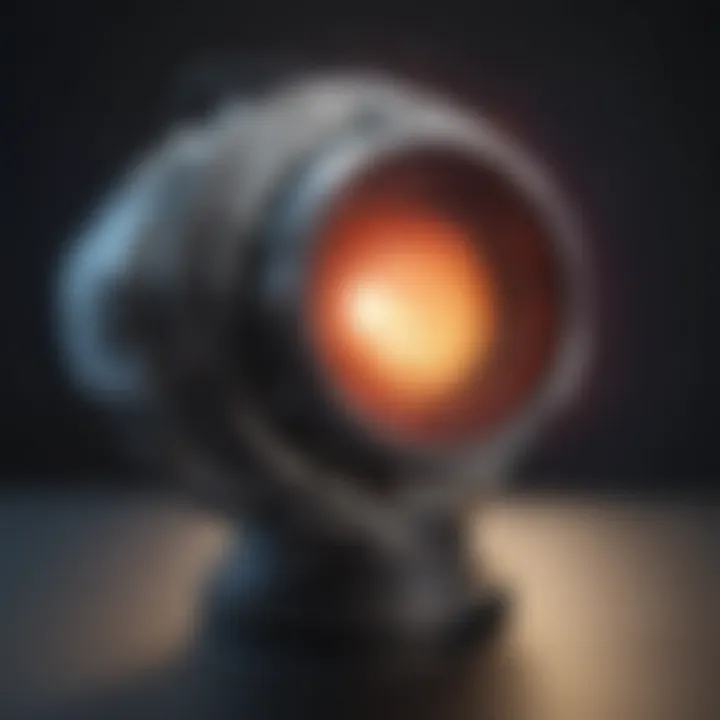
CAMB vs. CLASS
CAMB and CLASS are two prominent models in the cosmological simulation landscape.
CAMB: Primarily focused on calculating the angular power spectrum of the Cosmic Microwave Background (CMB), CAMB generates predictions based on a given set of cosmological parameters. It excels in providing rapid results with high accuracy, particularly in applications concerning CMB anisotropies.
CLASS: Contrarily, CLASS offers a broader range of functionalities. It can handle multiple cosmological phenomena including structure formation and the effects of modified gravity. This flexibility makes CLASS suitable for exploring unconventional theories. Moreover, CLASS integrates seamlessly with Boltzmann solvers, thus enabling a more comprehensive view of cosmic processes.
A key difference lies in the computational techniques; while CAMB is generally favored for its speed in CMB analysis, CLASS is appreciated for its flexibility and adaptability across various scenarios.
Strengths and Weaknesses
Every model has its strengths and weaknesses. CAMB shines in creating quick predictions, but it limits itself to classic cosmological setups. In contrast, its strengths include:
- Efficiency in computation: Rapid calculations lead to quicker results.
- Strong accuracy in standard cosmology: Proven effective in producing reliable power spectra.
However, it might not meet every research requirement. For example, CAMB lacks the ability to adequately address complex scenarios, such as modified gravity theories or interactions beyond the standard model.
In comparison, CLASS provides:
- Versatility in applications: Can model a diverse range of cosmological scenarios.
- Extended theoretical frameworks: Capable of incorporating advanced cosmological theories not addressed by CAMB.
Conversely, its weaknesses are:
- Slower computation: More extensive calculations lead to longer processing times.
- Complexity in usage: Can be challenging for newcomers due to its broad feature set.
Applications in Different Scenarios
Both CAMB and CLASS find applications in various contexts, reflecting their unique capabilities.
- CMB Analysis: CAMB is specifically designed for calculating power spectra of the CMB, making it ideal for projects focused on this area.
- Structure Formation: CLASS's range of features allows it to model the structure formation more comprehensively, considering factors like modifications in gravitational theories.
- Modified Gravity Tests: For researchers examining theories beyond standard cosmology, CLASS offers more tools and methods.
- Education and Training: CAMB’s straightforward design makes it a good starting point for students new to cosmology.
Interpreting Results from CAMB
Interpreting results from CAMB is crucial for appreciating its role as a cosmological computational tool. The outputs generated provide insights into the cosmic microwave background, large-scale structures, and theoretical predictions regarding the universe. By delving into the intricacies of this data, researchers can validate cosmological models and enhance our understanding of fundamental phenomena.
Understanding Output Data
The output data from CAMB typically includes power spectra related to the cosmic microwave background and matter distributions in the universe. To gather meaningful information from this output, it is essential to familiarize yourself with its structure and significance. Key components include:
- Power Spectrum: Displays the distribution of temperature fluctuations in the cosmic microwave background. Analyzing these fluctuations helps researchers deduce important cosmological parameters.
- Transfer Functions: These indicate how perturbations in density evolve over time, affecting the observed structure in the universe. Understanding these functions is vital for linking theory with observational data.
- Likelihood Functions: They assess the probability of observed data under different cosmological models. This is important for model selection and determining the most probable parameters.
Careful examination of these outputs enables accurate interpretations of the universe’s underlying mechanics.
Statistical Analysis of Outputs
Statistical analysis of CAMB’s outputs is indispensable for drawing robust conclusions. Researchers employ various statistical tools and techniques to evaluate these results. Important aspects include:
- Bayesian Inference: Utilizing Bayes' theorem to update the probability of a model as more data becomes available. This framework is essential in refining cosmological models based on observed data.
- Error Estimation: In any analysis, determining the uncertainties associated with the output is crucial. Error bars in power spectra help to assess the reliability of the results.
- Data Comparison: Comparing CAMB outputs against observational data from missions like WMAP and Planck reveals how well the model performs and whether adjustments are necessary.
By applying these statistical methods, researchers ensure their interpretations of CAMB data are grounded in rigor and accuracy.
Applications of Findings
The findings derived from CAMB have profound implications in various astrophysical contexts. Understanding these applications is crucial for appreciating the broader impacts of the research. Notable applications include:
- Cosmological Model Testing: Results from CAMB are fundamental in testing various cosmological theories. They help confirm or refute models like Lambda Cold Dark Matter (ΛCDM).
- Influence on Future Missions: Insights gained from CAMB inform the design and objectives of future observational missions. Data modeling with CAMB helps shape what astrophysical phenomena need further examination.
- Public Perception and Education: Simplified interpretations of CAMB data can bridge the gap between complex scientific concepts and public understanding, fostering interest in cosmology.
Through these applications, findings from CAMB advance our grasp of cosmic phenomena and the evolution of the universe.
Implications of CAMB on Cosmology
The implications of the Cosmological Monte Carlo Background (CAMB) model extend far beyond mere computations. CAMB serves as a foundational component that influences various aspects of cosmological research. By providing robust tools for simulating cosmic phenomena, it clarifies complex mechanisms that govern the universe's expansive behavior. In this section, we will delve into its significance within theoretical frameworks, its connections to dark matter and dark energy studies, and its capacity to enhance our understanding of the early universe.
Influence on Cosmological Theories
The application of CAMB has played a critical role in shaping cosmological theories. Its detailed calculations help refine understandings of cosmic expansion, structure formation, and the overall dynamics of the universe. The model allows researchers to test predictions against observational data from both current and past astronomical surveys.
- Impact on ΛCDM Model:
- Compatibility with Observations:
- Refinement of Parameters:
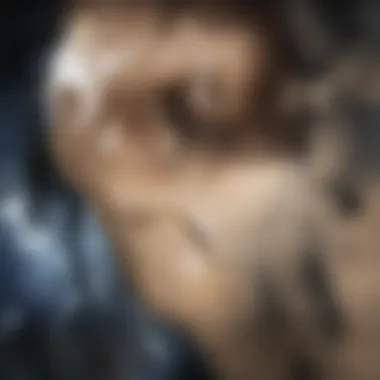
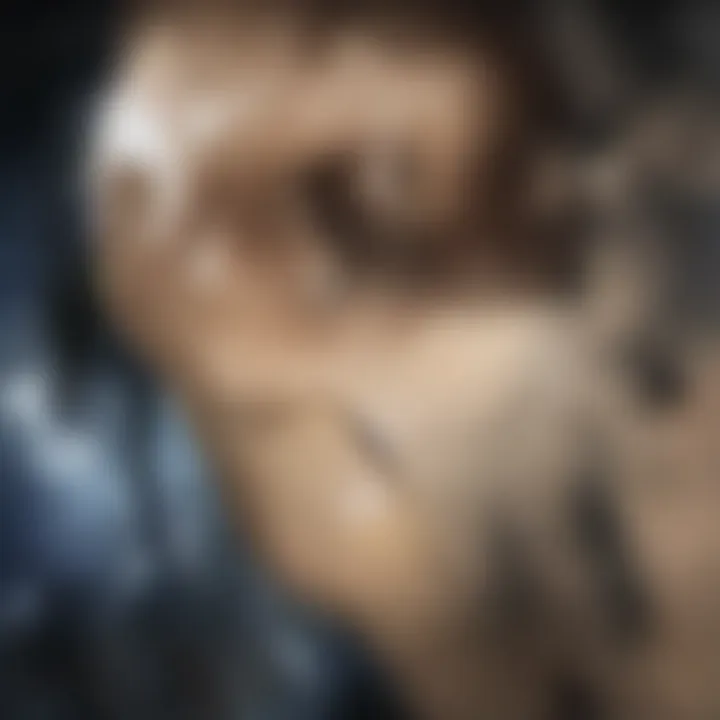
- The Lambda Cold Dark Matter (ΛCDM) model, which is the standard model of cosmology, heavily relies on predictions made by CAMB.
- It assists in analyzing the effects of different parameters on the evolution of the universe.
- By generating precise forecasts, CAMB aids in assessing how well theoretical frameworks align with observations. Its simulated spectra can be directly compared with anisotropies in the cosmic microwave background.
- Continuous usage and improvement of CAMB allow for the adjustment of fundamental parameters. This ongoing refinement feeds back into cosmological theories, enhancing their reliability.
Role in Dark Matter and Dark Energy Research
Dark matter and dark energy represent two of the most significant components in the cosmos. Their elusive nature poses challenges in understanding their effects. CAMB contributes to exploring these aspects in an insightful manner.
- Modeling Dark Matter:
- Understanding Dark Energy Dynamics:
- Predictive Analysis:
- CAMB facilitates the simulation of the structure formation influenced by dark matter. It provides a clearer picture of how dark matter interacts with visible matter, shaping galaxies and clusters.
- The model's ability to incorporate various forms of dark energy, such as quintessence, allows researchers to investigate how these forces affect cosmic expansion.
- The predictions derived from CAMB simulations help identify potential signatures of dark energy in future research, guiding observations and experimental designs.
Insights into the Early Universe
The early universe is a realm that remains largely speculative, yet essential for our understanding of cosmic history. CAMB offers a lens through which to study conditions shortly after the Big Bang.
- Quantum Fluctuations and Inflation:
- Cosmic Microwave Background Studies:
- Simulation of Structure Formation:
- CAMB helps model the quantum fluctuations during the inflationary period, offering insights into the uniform distribution of matter that we observe today.
- Results from CAMB are indispensable for interpreting measurements of the cosmic microwave background radiation, the remnant heat from the Big Bang. Understanding these measurements is crucial for piecing together the universe's early development.
- By simulating how structures emerged in the early universe, CAMB aids in explaining the large-scale organization of the cosmos.
"The CAMB model simplifies complex calculations and makes it easier for researchers to connect theoretical predictions with empirical data."
In summary, the implications of CAMB in cosmology are profound and varied. By influencing cosmological theories, shedding light on dark matter and dark energy, and providing insights into the early universe, CAMB remains an essential tool in the cosmologist's arsenal.
Future Directions in CAMB Research
The field of cosmology is continuously evolving, and the CAMB model is at the forefront of these advancements. Future directions in CAMB research are crucial as they determine how cosmological studies can improve and adapt to new findings and technologies. Several aspects are emerging that promise to enhance the functionality and applicability of CAMB. This section will delve into three key areas: potential upgrades to algorithms, integration with emerging physics, and expanding applications in interdisciplinary research.
Potential Upgrades to Algorithms
Enhancing the algorithms used within the CAMB framework is vital to improve efficiency and accuracy. Researchers can focus on developing numerical methods that reduce computation time while maintaining high precision. Upgrades in algorithms allow for the exploration of more complex models of the universe, facilitating simulations that account for various cosmological parameters such as inflation and perturbative growth. For instance, using methods like adaptive mesh refinement can yield better resolution in specific areas of interest without a proportional increase in computational load. This has significant implications in studying regions where dark matter clumping is a focus. Moreover, revisiting parallel processing techniques can allow CAMB to utilize modern computing resources more effectively. This enhancement is essential as simulations become larger and more complex.
Integration with Emerging Physics
Another significant direction for future CAMB research is its integration with emerging physics. As our understanding of fundamental forces and cosmic components evolves, CAMB must adapt to include these new theories. For instance, incorporating modifications to general relativity or theories involving modified gravity can provide deeper insights into cosmic evolution. Experimental findings from projects exploring dark matter and dark energy might also necessitate updates in CAMB's framework.
By integrating these emerging fields, researchers can produce simulations that reflect more accurately the reality of the universe. This integration could potentially illuminate aspects of the universe previously thought to be beyond comprehension. Keeping CAMB dynamic ensures that it remains a significant tool in cosmology as theories advance.
Expanding Applications in Interdisciplinary Research
Finally, expanding applications of CAMB in interdisciplinary research is a critical path forward. The insights gained from cosmological simulations have broad implications across various scientific domains, including astrophysics, particle physics, and even fields like statistical mechanics. The collaboration of cosmologists with particle physicists can lead to the development of unified theories that help in understanding both cosmic structures and subatomic particles.
Additionally, CAMB could find applications in the analysis of astronomical data, leading to better interpretation of cosmic phenomena. Working alongside other models like the ones used in galaxy formation studies can also synergize findings and promote comprehensive analysis of the cosmic web.
"Interdisciplinary research not only strengthens the fabric of scientific inquiry but also enhances the accuracy and scope of what CAMB can offer to the scientific community."
Closure
The conclusion serves as an essential component in this article, summarizing the discussions surrounding the CAMB model. It crystallizes the significance of the information and insights provided in the various sections, making it easier for readers to grasp the overarching themes and implications.
Summary of Key Points
In our exploration of CAMB, several key elements emerged that highlight its relevance in cosmology:
- Foundational Understanding: CAMB plays a pivotal role in simulating the cosmic microwave background and studying large-scale structures in the universe.
- Mathematical Rigor: Its mathematical framework underlies cosmological predictions, revealing the intricate relationships between various cosmological parameters.
- Technological Innovation: The advances in computational techniques and software developments underscore the continuous progress being made in this field.
- Interdisciplinary Applications: The implications of CAMB extend beyond basic cosmological research, fostering dialogue across different scientific disciplines.
"Understanding CAMB is central not just for cosmologists, but for anyone interested in the fabric of our universe."
Future of CAMB in Cosmology
The future of CAMB is promising. As computational power continues to grow, the potential upgrades to algorithms are likely to enhance the precision and scope of simulations.
- Enhanced Algorithms: Future versions could incorporate new physics and improve the handling of data, yielding insights previously unattainable.
- Integration of Novel Concepts: Emerging physics, such as modifications in our understanding of dark energy, could reshape how CAMB is utilized.
- Broadened Applications: Expanding CAMB's applications to interdisciplinary research increases its impact, bridging gaps between cosmology, physics, and other relevant fields.
In sum, concluding this narrative reinforces the pivotal role CAMB plays in not just explaining the cosmos, but also in shaping the future of cosmological research.