Mastering HDR CRISPR: A Detailed Exploration
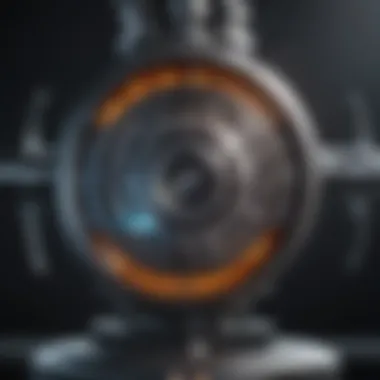
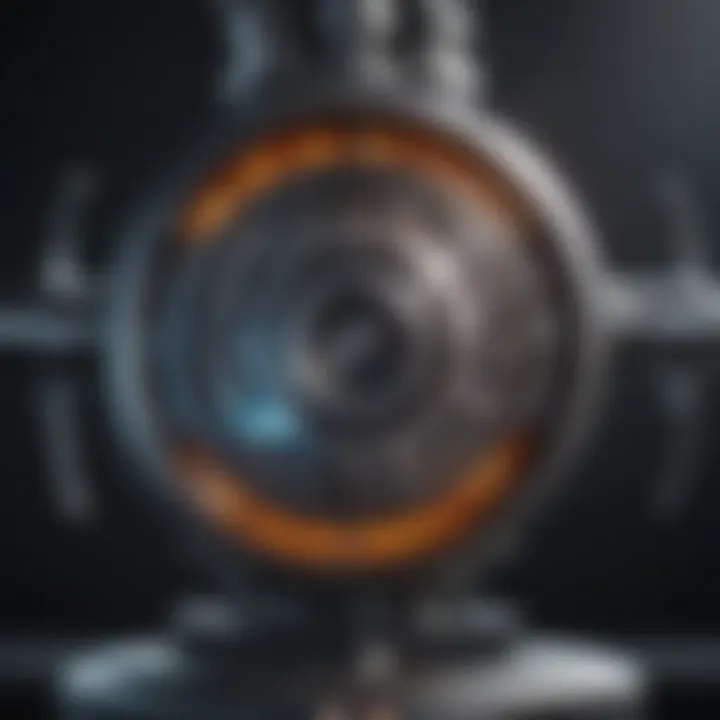
Intro
The Homology-Directed Repair (HDR) CRISPR protocol is a cornerstone in the field of genetic engineering. Researchers increasingly recognize the importance of precise genome editing in various applications, from creating disease models to developing novel therapies. This comprehensive guide will explore the HDR CRISPR protocol, its mechanisms, applications, and the advancements that are shaping its future.
Understanding HDR is essential for anyone working in genetics or biotechnology. The HDR process utilizes a specific repair pathway in cells, allowing for high fidelity in repairing double-strand breaks in DNA. This method stands in contrast to more random repair processes, firmly positioning HDR as a critical tool for making targeted changes in an organism's genetic material.
Research Overview
Summary of Key Findings
Throughout our exploration of HDR CRISPR, several key insights emerge:
- HDR offers a precise approach to genome editing, enhancing the accuracy of modifications.
- Technical advancements, such as improved donor template design, significantly increase HDR efficiency.
- HDR combines with other CRISPR methods, broadening its applications across different organisms.
Background and Context
The significance of HDR can be traced back to its roots in molecular biology. Initially, researchers sought to understand DNA repair mechanisms, eventually leading to the understanding that these processes could be harnessed to introduce specific genetic modifications. The CRISPR system, known for its utility in genome editing, discovered a synergistic relationship with HDR, offering unprecedented control over the genetic landscape of organisms.
As the field continues to evolve, an in-depth grasp of HDR and its practical relevance becomes increasingly crucial for researchers and practitioners alike. With the rise of synthetic biology and therapeutic advancements, HDR represents a transformative shift in how we manipulate genetic information.
Methodology
Experimental Design
This guide utilizes a qualitative approach to synthesize current literature on HDR CRISPR protocols. By reviewing peer-reviewed articles and ongoing research, we delineate the mechanisms that underlie HDR, alongside the pragmatic applications of this protocol.
Data Collection Techniques
Data for this article was gathered from various reputable sources, including scholarly journals and academic databases. The focus lies on recent advancements and research findings that elucidate the HDR CRISPR protocol's potential.
Accurate and consistent methodological designs are pivotal to producing reliable data in genetic engineering. As such, a robust experimental framework must always be in place.
"The beauty of HDR lies in its potential to marry precision with efficiency, opening doors to possibilities previously thought unattainable."
This exploration will unfold chronologically, delving into the developments that underscore the HDR CRISPR protocol, thus catering to the interests of students, researchers, educators, and professionals.
Preface to HDR CRISPR Protocol
The HDR CRISPR protocol represents a crucial advancement in genetic engineering. Understanding this protocol is essential for researchers who aim to achieve precise genome editing. The significance of HDR, or Homology-Directed Repair, lies in its ability to facilitate targeted modifications to DNA, enabling scientists to correct genetic mutations or introduce new sequences effectively. This section will lay the groundwork for understanding the relevance and nuances of HDR within the broader scope of CRISPR technology.
Understanding CRISPR Technology
CRISPR, or Clustered Regularly Interspaced Short Palindromic Repeats, is a powerful tool for gene editing. It operates by using the Cas9 protein, guided by a specific RNA sequence, to cut DNA at desired locations. This cutting action allows for various forms of genomic alterations, such as inserting, deleting, or modifying genes. The ease of use and versatility of CRISPR make it a preferred choice for genetic modification across multiple fields, including medicine and agriculture.
The CRISPR technology framework also encapsulates its ability to integrate with HDR mechanisms. When CRISPR creates a double-strand break in the DNA, the cell's natural repair systems spring into action. This is where HDR plays a pivotal role, directing accurate repair by utilizing a DNA template. It enhances the precision of gene editing and minimizes the uncertainties related to random insertion events that often occur in other methods like non-homologous end joining (NHEJ).
Significance of HDR in Genome Editing
HDR is distinct because it allows for the integration of precise genetic modifications alongside existing genomic sequences. This specificity is vital, particularly when the aim is to correct specific alleles or introduce precise mutations without disrupting other essential gene functions. The advent of HDR CRISPR protocols have transformed the landscape of genome editing by offering unprecedented levels of control over genetic outcomes.
Moreover, the ability to customize gene sequences opens doors for considerable advancements in treating genetic disorders, developing new therapeutics, and enhancing crop resilience in agriculture.
HDR is viewed as a critical method to achieve precision in genome editing. Its application is pivotal for both research and therapeutic advancements.
However, working with HDR requires a clear understanding of the components involved, the workflow necessary for implementation, and an awareness of the associated challenges. This introductory section sets the stage for a deeper dive into these important elements.
Mechanisms of HDR
Understanding the mechanisms of Homology-Directed Repair (HDR) is crucial for anyone exploring the HDR CRISPR protocol. This knowledge not only enriches foundational comprehension but also informs applications in genome editing. HDR is essential for making precise changes to DNA, particularly where accuracy is paramount, such as therapeutic gene editing. The intricate mechanisms involved in HDR allow for greater control over genetic outcomes, which can lead to significant advancements in both research and clinical applications.
Molecular Basis of Homology-Directed Repair
Homology-Directed Repair is a natural DNA repair pathway that utilizes a homologous sequence as a template for repair. This mechanism is particularly important when double-stranded DNA breaks occur. In HDR, the broken end of the DNA molecule seeks a homologous template, which can be derived from either the sister chromatid or an exogenous donor template. This process is tightly regulated and involves several key proteins, including Rad51, which play a major role in forming the nucleoprotein filament essential for strand invasion.
- Steps in HDR Process:
- Recognition and processing of the double-strand break.
- Formation of the Rad51 nucleoprotein filament.
- Homology search and strand invasion.
- DNA synthesis using the homologous template.
- Resolution of joint molecules, leading to correctly repaired DNA.
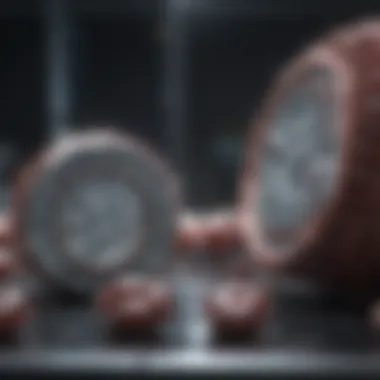
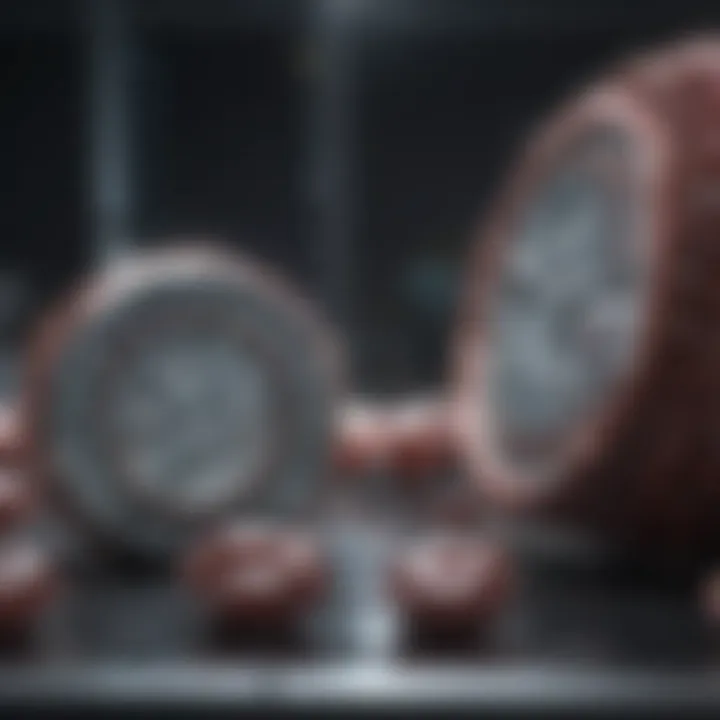
Moreover, HDR exhibits distinct phases. The initial recognition of the break by the cell machinery leads to extensive resection of DNA ends, generating singles-stranded DNA regions. These regions then promote the recruitment of Rad51. After finding a complementary sequence, an exchange can occur, resulting in a high-fidelity repair.
HDR allows for precise genome modifications, minimizing errors that might otherwise lead to mutations, a significant advantage over other repair mechanisms.
Comparison with Other DNA Repair Mechanisms
HDR exists alongside other DNA repair mechanisms, namely Non-Homologous End Joining (NHEJ) and Single-Strand Annealing (SSA). The primary difference between these methods lies in their fidelity and the pathways they choose for restoring DNA integrity. While NHEJ tends to be more error-prone, often resulting in insertions or deletions, HDR stands out for its ability to maintain the original sequence with minimal alteration.
- Non-Homologous End Joining:
- Single-Strand Annealing:
- Functions rapidly to repair breaks.
- Does not require a homologous template.
- More prone to errors, which can disrupt gene function.
- Utilizes repeated sequences flanking the break.
- Can lead to deletions if sequences are homologous.
These comparisons underline HDR's significance, especially in contexts where precision is essential, like therapeutic gene targeting and genetic enhancements.
In summary, understanding the mechanisms underlying HDR is vital for harnessing its potential in genetic engineering. Researchers can apply this knowledge to design experiments that leverage the strengths of HDR over other DNA repair mechanisms. By optimizing protocols and conditions that favor HDR, the field of genetic editing can advance toward greater effectiveness in achieving desired genetic alterations.
Components of the HDR CRISPR Protocol
The HDR CRISPR protocol is pivotal for precise genome editing. It is essential to understand its key components, which enable the successful application of this technique in a variety of fields such as medicine and agriculture. The protocol centers around specific elements that work in synergy to achieve high precision in genetic modifications.
CRISPR-Cas9 System Overview
The CRISPR-Cas9 system is a groundbreaking tool in genetic engineering. It consists of two main components: the Cas9 enzyme and a guide RNA. The Cas9 enzyme acts as molecular scissors that cut DNA at designated locations. The guide RNA directs Cas9 to the specific sequences of DNA that need editing. This targeted approach is crucial, as it ensures that modifications occur only at intended sites, minimizing unintended changes.
Using the CRISPR-Cas9 system offers several benefits:
- Precision: It allows for accurate targeting of specific DNA sequences for modifications.
- Versatility: It can be applied across various organisms, making it suitable for diverse research areas.
- Efficiency: The process can be performed relatively quickly compared to other genome editing techniques.
This system simplifies the engineering of organisms, contributing to advancements in fields such as genomics and synthetic biology. Researchers can precisely knock out genes or replace them with desired sequences. This flexibility makes CRISPR-Cas9 an essential platform in HDR applications.
Designing HDR Donor Templates
The design of HDR donor templates is another critical component of the HDR CRISPR protocol. HDR relies on these templates to guide the repair of the DNA after the Cas9 cuts it. The templates must be carefully crafted to include homologous sequences flanking the cut site. These homologous sequences facilitate the integration of new genetic material into the target genome.
Key considerations when designing HDR donor templates include:
- Length of Homology Arms: The length of the homologous sequences can affect the efficiency of HDR. Typically, longer arms enhance the chance of successful integration.
- Sequence Identity: Donor templates must match the target sequence closely to direct accurate repair.
- Avoiding Unwanted Mutations: Care should be taken to prevent introducing unintended mutations within the donor sequence.
Effective donor template design increases the chance of successful HDR outcomes. With well-defined templates, researchers can enhance the precision of their genetic interventions. It is through these elements that the HDR CRISPR protocol achieves its aim of precision in genome editing.
Experimental Workflow
The experimental workflow is fundamental in the HDR CRISPR protocol, as it outlines the strategic steps for successful gene editing. This process not only ensures the effective application of CRISPR technology but also maximizes the potential for successful gene modifications. Understanding each phase in the workflow helps researchers to refine techniques, reducing errors and improving outcomes.
Thorough planning in this workflow is paramount. The stages of cell line selection, transfection methods, and screening for HDR efficiency must be executed with precision. This improves not just the quality of results, but also the reliability of data generated during experiments, allowing for replication and further studies.
Cell Line Selection and Preparation
Choosing the appropriate cell line is the first and perhaps the most critical step in the HDR CRISPR protocol. Different cell lines exhibit varying responses to gene editing techniques, so selecting one that aligns with the research goals is crucial.
Commonly used cell lines include HEK293T for human studies and various cancer cell lines for oncological research. The selected cell lines should ideally be well-characterized to ensure reproducibility in results. Once a cell line is chosen, proper preparation involves maintaining optimal growth conditions. This ensures that the cells are in the best state for transfection, improving the chances of successful HDR.
Transfection Methods for HDR
Transfection is a process through which genetic material is introduced into cells. Several methods can be utilized, including lipofection, electroporation, and viral transduction, each having unique advantages. Lipofection is often favored for its simplicity and efficiency in many laboratories.
- Lipofection: Involves using lipid-based reagents to facilitate the uptake of plasmid DNA into cells.
- Electroporation: Applies an electric field to create temporary pores in the cell membrane, allowing DNA to enter.
- Viral Transduction: Uses modified viruses to deliver genetic material into cells, achieving higher transfection rates, especially in hard-to-transfect cells.
The choice of method can impact HDR efficiency significantly. Therefore, researchers should consider the specific characteristics of the target cells and desired outcomes when selecting a transfection method.
Screening for HDR Efficiency
After transfection, evaluating HDR efficiency is critical to validate the success of the gene editing process. Various methods exist for this purpose. One common approach is to set up a selection strategy that allows researchers to identify successfully edited cells. This generally involves creating a reporter system where edited genes produce a visible or selectable marker.
Techniques such as PCR, Sanger sequencing, and next-generation sequencing can be employed to quantify HDR events at a molecular level.
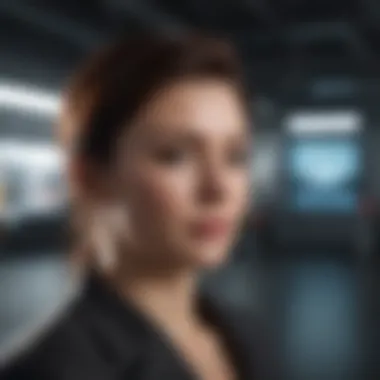
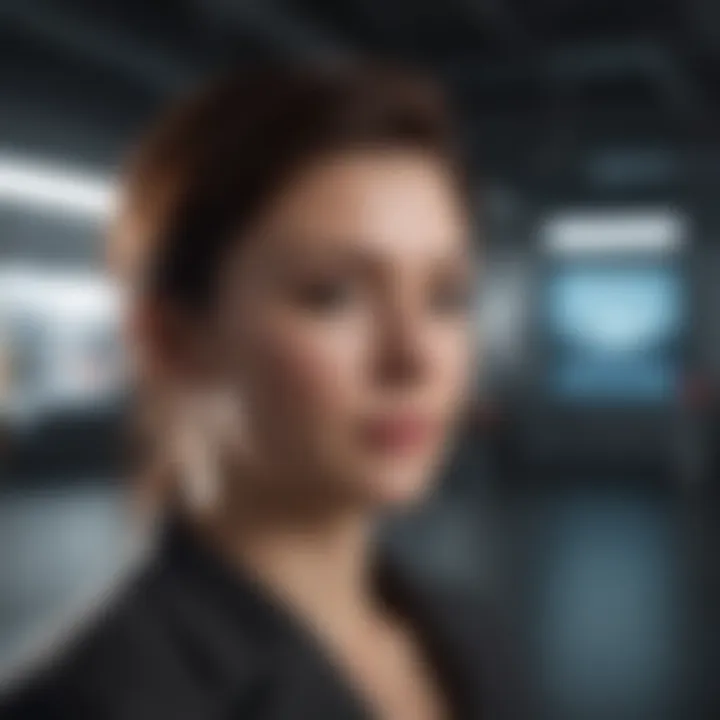
"The success of the HDR protocol highly depends on the combination of all experimental steps taken prior. Rigorous screening methods provide insights into the editing efficiency, thus guiding further optimization."
Through these stages, from cell line choce to effective screening, the experimental workflow ensures that researchers can achieve reliable and reproducible results in their HDR CRISPR applications.
Applications of HDR CRISPR Protocol
The Homology-Directed Repair (HDR) CRISPR protocol stands as a cornerstone in the realm of genetic engineering. Its applicability spans various domains, including biomedical research and agriculture. This section delves into the significant applications of HDR CRISPR, further illuminating its essential role.
Biomedical Research and Therapeutics
In biomedical research, HDR CRISPR technologies enable precise genome modifications, which facilitate the understanding of genetic disorders and the development of novel therapies. By utilizing HDR, researchers can correct mutations at specific loci within the genome. This is especially useful in conditions where a single nucleotide variation leads to a disease. For instance, scientists are exploring HDR for conditions such as cystic fibrosis and sickle cell anemia, where targeted corrections can potentially reverse the pathological effects.
Moreover, HDR CRISPR can be leveraged to create disease models in cell lines or organisms, thus advancing our understanding of disease mechanisms. The precise alterations made possible by HDR enable researchers to investigate gene functions accurately and can provide insights into treatment responses.
Key benefits of using HDR in biomedical applications include:
- Precision: Specific gene editing allows targeted therapy, minimizing off-target effects that can arise from other methods.
- Versatility: The ability to introduce larger DNA sequences expands the scope of therapeutic possibilities.
- Model Systems: Enhanced gene editing techniques facilitate the development of accurate model systems for studying diseases.
"The accuracy of HDR CRISPR allows for corrections that were unimaginable with older gene editing methods."
Agricultural Enhancements and Crop Improvement
In agriculture, the HDR CRISPR protocol is revolutionizing crop improvement strategies. This method permits the validation and customization of plant genomes, ensuring that crop traits remain resilient in the face of climate change and pests. The application of HDR makes it possible to insert beneficial traits, such as drought resistance or improved nutritional content, into crops like corn, rice, and wheat.
HDR techniques also contribute to sustainable practices. By enhancing traits that require fewer chemical inputs, such as pesticides or fertilizers, HDR CRISPR can foster environmentally friendly agricultural solutions. This is crucial in meeting the growing food demands while maintaining ecosystem balance.
Some of the primary benefits found in agricultural applications are:
- Enhanced Yield: Targeted traits can increase harvests with reduced vulnerability to diseases.
- Nutritional Improvement: Crops can be modified for increased vitamins or minerals, enhancing food security and health.
- Sustainability: Minimizing the environmental impact of agriculture through increased resistance to biotic and abiotic stresses.
In summary, the HDR CRISPR protocol shows tremendous potential in both biomedical and agricultural domains. Its implications extend beyond mere genetic modifications, shaping future therapeutic avenues and sustainable agricultural practices. Researchers and practitioners must remain aware of these advancements to leverage HDR CRISPR fully.
Challenges in HDR CRISPR Protocols
The HDR CRISPR protocol has transformed genetic engineering by allowing targeted modifications to the genome with remarkable precision. However, the application of this technology is not without its challenges. Understanding these challenges is essential for researchers and practitioners in the field, as they can influence the outcomes of experiments and the broader implications of gene editing.
Limitations in Efficiency
One of the primary challenges in HDR CRISPR protocols is the efficiency of the homology-directed repair process itself. HDR can occur in less than 20% of cases in various cell types. Several factors contribute to this low efficiency:
- Cell Type: Different cell types exhibit varying capacities for HDR. For instance, stem cells may show higher rates of HDR compared to differentiated somatic cells.
- Donor Template Design: The way the donor template is designed significantly affects HDR efficiency. Templates need to be long enough to provide sufficient homology but also optimized to avoid undesirable insertions.
- Cell Cycle Phase: HDR is most active during the S and G2 phases of the cell cycle. Targeting cell populations in these phases is crucial for improving repair rates.
Despite these limitations, ongoing research is focusing on improving these aspects. Methods such as enhancing the accessibility of the DNA template via chromatin remodeling or synchronizing cells in specific phases of the cell cycle are areas of active investigation.
Off-Target Effects and Specificity Issues
Another significant challenge in using HDR CRISPR protocols is the potential for off-target effects. These unintended modifications can complicate the interpretation of experimental results, posing risks in therapeutic applications. Multiple factors can contribute to these specificity issues:
- Guide RNA Design: The selection of guide RNAs is critical. Low specificity guides can lead to unwanted editing at genomic sites similar to the target sequence. Researchers must carefully design and validate guides to minimize this risk.
- Cas9 Variants: Different Cas9 variants have been shown to have distinct off-target profiles. Identifying the right variant, such as SpCas9 or SaCas9, may help balance efficiency and specificity.
- Detection Methods: There is a need for robust methods to assess off-target effects. Techniques such as whole-genome sequencing or targeted deep sequencing are essential to identify potential off-target mutations.
"To address off-target effects, it is vital to combine advanced guide RNA design with precise screening techniques to ensure the fidelity of CRISPR applications."
By actively addressing these challenges, the genomic editing community can enhance the safety and efficiency of HDR CRISPR protocols, paving the way for more reliable applications in both research and medicine.
Recent Advancements in HDR Techniques
Recent advancements in HDR techniques have significantly transformed gene editing practices. As the field of genetic engineering grows, researchers focus on refining these methods to increase precision and efficiency. This section highlights pivotal aspects of current advancements in HDR, addressing benefits, considerations, and the impact on future research.
CRISPR Enhancements in HDR
One of the most notable enhancements in HDR techniques involves improving the CRISPR-Cas9 system itself. Various modifications to the Cas9 protein have been developed to enhance specificity and efficiency. For instance, engineered versions of Cas9, such as eSpCas9 and SpCas9-HF1, exhibit reduced off-target activity compared to the wild-type Cas9. These variants yield better precision in genome edits, thus minimizing unintended mutations.
In addition to protein modifications, the advancement of guide RNA (gRNA) design has also proven essential. The use of longer gRNAs or multi-targeting arrays allows researchers to effectively target multiple loci within the genome simultaneously. This maximizes the utility of individual HDR events, thus increasing the potential for comprehensive genomic modifications.
Furthermore, innovative techniques like prime editing and base editing have emerged as enhancements to traditional HDR methods. These approaches enable accurate modifications with fewer errors. Prime editing, for example, allows for precise insertions and deletions without causing double-strand breaks, which are inherent to previous HDR techniques. This ability to edit DNA with a high degree of accuracy marks a significant step forward in harnessing HDR for therapeutic and research applications.
Novel Donor Templates for Increased Precision
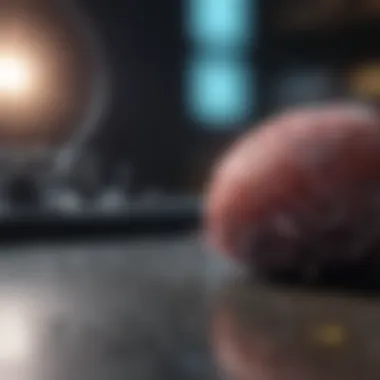
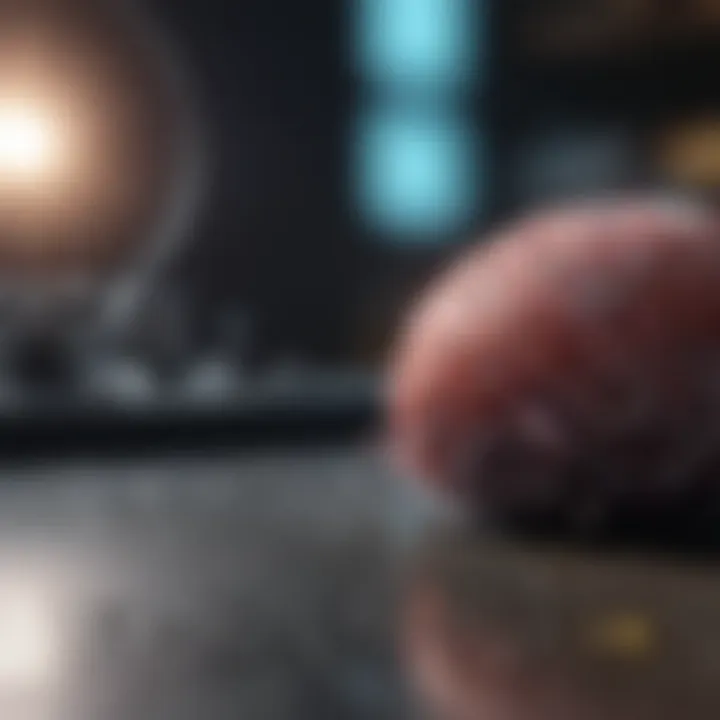
The choice of donor templates is critical in HDR protocols. Recent advancements have focused on developing novel systems for donor templates, which significantly influence HDR success rates. Single-stranded oligonucleotides (ssODNs) have gained prominence due to their simplicity and effectiveness. These ssODNs provide a clean way to introduce single-nucleotide changes or small insertions without the complexities associated with larger donor constructs.
Another emerging trend is the use of circular donor templates. These templates can enhance the stability of donor DNA within a cell, increasing the likelihood of successful integration during the HDR process. Researchers have noted that circular templates sometimes yield more efficient and reliable integration compared to linear forms.
Moreover, advancements in synthetic biology allow for the creation of more complex donor templates that can include features like multiple editing sites or regulatory elements. This provides the ability to not only edit sequences but also control expression levels and achieve more refined genetic alterations.
"The integration of novel donor strategies with robust CRISPR tools signifies a promising horizon for precise genome editing."
Ethical Considerations in Using HDR CRISPR
The implementation of HDR CRISPR technologies brings forth several ethical considerations that merit careful examination. Understanding these aspects is essential for researchers, practitioners, and society as a whole. The implications of using gene editing tools extend beyond scientific advancement; they touch upon moral, social, and regulatory domains.
Among the pressing questions are those related to societal impacts of gene editing. As HDR CRISPR allows for precise modifications to the genome, it presents opportunities for improving health outcomes, eradicating genetic disorders, and enhancing agricultural productivity. However, the potential to modify human DNA raises concerns about unintended consequences. For example, genetic editing in embryos could lead to germline alterations that might affect future generations. There exists a tension between the pursuit of progress and the ethical need to maintain a commitment to human rights and dignity.
"Ethics must play a role in guiding the application of sophisticated tools like HDR CRISPR to weigh benefits against potential risks to future generations."
In addition to individual health concerns, there lies the broader issue of equity in access to these technologies. If HDR CRISPR becomes exclusively accessible to certain populations, it may exacerbate existing health disparities. The risk of creating a divide between those who can afford genetic enhancements and those who cannot is a valid concern that needs addressing.
Societal Impacts of Gene Editing
The societal impacts of gene editing can be far-reaching. HDR CRISPR technology has the potential to revolutionize medicine by allowing for the correction of genetic mutations responsible for inherited diseases. Examples include conditions like sickle cell anemia and cystic fibrosis. Yet, the possibility of 'designer babies' raises ethical debates about eugenics, parental choice, and societal pressure to conform to perceived norms of desirability.
Moreover, there is the environmental aspect to consider. In agriculture, gene editing can reduce the need for pesticides and herbicides, promoting sustainable practices. However, risks associated with genetically modified organisms must be weighed, including long-term effects on biodiversity and ecosystem stability.
Regulatory Framework for CRISPR Research
A robust regulatory framework is critical to ensure ethical research and application of HDR CRISPR technologies. Various countries have adopted different approaches to governing genetic research, which creates an uneven landscape. In the United States, the Food and Drug Administration (FDA) plays a vital role in overseeing genetic therapies, while organizations like the National Institutes of Health (NIH) set guidelines for research funding.
Globally, organizations such as the World Health Organization (WHO) are working towards establishing ethical codes applicable across borders. These regulations aim to prevent misuse and promote responsible conduct of research. Continuous dialogue among scientists, ethicists, and policymakers is necessary to navigate the complexities inherent in gene editing.
Future Perspectives on HDR CRISPR Protocol
The exploration of HDR CRISPR Protocol is a critical area within the field of genetic engineering. Looking forward, the future perspectives on this protocol reveal a landscape rich with possibilities and considerations. Current advancements in technology are pushing the boundaries of what is achievable in genome editing. The prospects of HDR techniques not only promise to refine existing processes but also to introduce novel approaches that elevate precision and efficacy in genetic modifications.
The integration of next-generation sequencing techniques and bioinformatics is crucial for enhancing HDR. These tools can facilitate a more refined selection of target sites within the genome, allowing researchers to navigate intricate genetic landscapes with improved accuracy. Furthermore, as understanding of the HDR mechanisms deepens, new methodologies to overcome challenges in efficiency and specificity will likely emerge.
Focusing on efficiencies that leverage HDR in therapeutic applications could pave the way for revolutionary treatments. This is especially relevant in gene therapies aimed at diseases with genomic roots, such as certain cancers and heritable disorders. The adaptability of HDR protocols might enable targeted treatments that minimize off-target effects, providing safer options for patients.
"The future of HDR CRISPR technology holds great promise, enabling precision medicine and evolutionary applications in genetic therapies."
Next Generation Techniques in Genome Editing
Next-generation techniques in genome editing are making headlines for their revolutionary potential in the scientific community. These methods, in conjunction with traditional HDR strategies, present a more comprehensive toolset for genetic manipulation.
Among these are advancements in base editing and prime editing. Base editing allows for the direct conversion of one DNA base pair to another without causing double-strand breaks, reducing potential errors compared to traditional methods. This is crucial for high-fidelity edits that HDR frequently aims to achieve. Prime editing offers even greater precision, enabling targeted edits with minimal unintended consequences. Both techniques may integrate seamlessly with HDR protocols, enhancing overall outcomes for genome editing projects.
Research institutions are also focusing on the minimization of disruption during editing processes. Reducing the cellular stress caused by editing events is essential for improving cell viability and achieving higher rates of HDR. Techniques that employ altered ribonucleoproteins or small molecules to modulate the DNA repair landscape are promising strategies that could yield significant benefits.
Integrating HDR with Other Genomic Technologies
Integrating HDR with other genomic technologies can present a multifaceted approach to tackling complex genetic challenges. One notable aspect is how HDR can be combined with CRISPR's cutting-edge gene drives. Gene drives can bias inheritance patterns, ensuring that desired traits propagate through populations. When integrated with HDR, researchers can ensure that these traits are introduced with high precision.
Another significant integration is with synthetic biology. The rational design of novel proteins and pathways can enhance the efficacy of HDR by creating optimum conditions for DNA repair. Coupled with computational modeling, these efforts can streamline the genetic editing process by predicting the most favorable editing outcomes.
Moreover, advancements in AI and machine learning are poised to revolutionize how HDR protocols are designed and implemented. AI can analyze vast amounts of genetic data to predict outcomes and optimize donor template designs, leading to better-targeted interventions and reducing trial and error in experiments. This approach can accelerate progress within the HDR landscape, making it an essential aspect of future research endeavors.
End
The conclusion of an article serves as the essential wrap-up. In the context of this comprehensive guide on HDR CRISPR protocol, it synthesizes the key insights while also prompting reflection on future pathways in genetic research. This final section addresses the main concepts discussed earlier, tying them together cohesively.
Summary of Key Insights
Several critical insights emerge from the exploration of the HDR CRISPR protocol. First, HDR presents a powerful means for precise genome editing. It relies on natural repair mechanisms to correct or introduce changes in DNA with high accuracy. Unlike other methods, HDR is particularly effective when dealing with specific genetic alterations, making it invaluable in fields like therapeutic development and crop improvement. Additionally, the importance of designing optimal donor templates is underscored in maximizing HDR efficiency. Research indicates that customized templates can significantly increase the likelihood of successful edits.
Moreover, researchers should remain vigilant concerning the challenges presented by efficiency limitations and off-target effects. These factors can greatly influence the outcomes of HDR applications. Awareness of these issues allows scientists to make informed decisions as they refine their techniques and methodologies.
Lastly, ethical considerations surrounding genetic modifications cannot be overlooked. As scientists navigate the complexities of HDR CRISPR, they must also contemplate the broader societal impacts of their work and engage in discussions about regulations guiding such research.
Ongoing Research and Future Directions
The landscape of HDR CRISPR protocols is evolving. Ongoing research focuses on enhancing the efficiency and precision of HDR techniques. Innovations in vector design and improved methods for delivering CRISPR components into cells are areas of active investigation. Such advancements could lead to more reliable outcomes in various applications, from medicine to agriculture.
Additionally, integrating HDR protocols with other genomic technologies promises to produce synergistic effects. Combining strategies like base editing or prime editing could lead to unprecedented accuracy in genome editing. As researchers pursue these avenues, the field of genetic engineering stands on the brink of remarkable developments.