Exploring Ultrasonic Waves: Properties and Uses
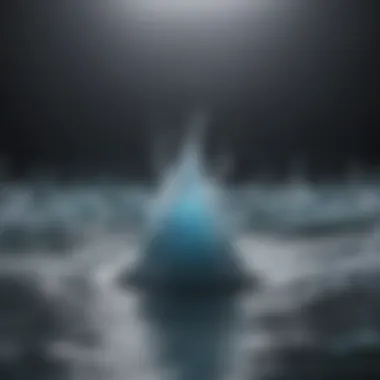
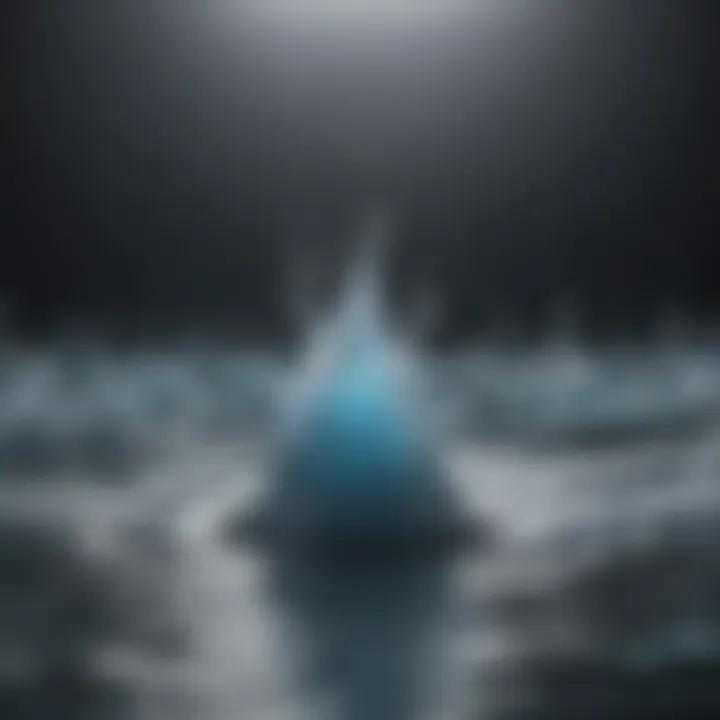
Intro
Ultrasonic waves play a crucial role in various fields, extending from medical applications to industrial testing. Ultrasonic waves are sound waves with frequencies above the audible range for humans, typically above 20 kHz. Understanding their properties—how they are generated, how they propagate through different mediums, and how they interact with other materials—provides valuable insights into their numerous applications.
The emergence of ultrasonic technology can be traced back to its basic scientific principles, which combine elements of physics and engineering. As we delve into the intricacies of ultrasonic waves, we uncover the practical implications of their use. In this article, we aim to present a detailed overview of ultrasonic waves, emphasizing not just their advantages but also addressing the safety concerns and limitations that accompany their deployment.
Research Overview
Summary of Key Findings
Researchers have identified several significant characteristics of ultrasonic waves that contribute to their versatility. Key findings indicate that:
- Ultrasonic waves exhibit high frequency, making them suitable for applications requiring precision.
- The ability to penetrate various materials allows for non-destructive testing in industrial contexts.
- Medical applications, particularly in imaging and therapeutic areas, showcase their importance in modern healthcare.
Background and Context
The investigation into ultrasonic waves began over a century ago, gaining momentum with technological advancements. Initially utilized in underwater exploration, ultrasonic waves now find extensive use in multiple disciplines. The advancements in transducer technology have improved the generation and reception of these waves, allowing for more practical and effective applications.
Ultrasonics are not just limited to medical imaging such as ultrasounds; they are found in cleaning processes, material characterization, and even in pest control. Such diverse applications underscore the relevance of this technology in contemporary society.
Intro to Ultrasonic Waves
Ultrasonic waves play a crucial role in various scientific and industrial applications. Understanding these waves is not just a matter of academic interest; it has practical implications in fields ranging from medicine to manufacturing. This section serves as an entry point to the fascinating world of ultrasonic technology, setting the stage for deeper exploration of its various aspects.
Definition of Ultrasonic Waves
Ultrasonic waves are sound waves with frequencies above the upper limit of human hearing, typically above 20 kHz. These waves propagate through different media such as liquids, gases, and solids. Due to their high frequency, ultrasonic waves possess unique properties that make them useful in applications where traditional sound waves are inadequate. For instance, their ability to penetrate materials and provide high-resolution images is essential in medical imaging.
Historical Background
The study of ultrasonic waves dates back to the early 20th century. The first applications were primarily in navigation, particularly using sonar technology. In World War I, submarines employed ultrasonic waves for detecting enemy vessels. Since then, advancements in technology led to the expansion of ultrasonic applications. By the mid-20th century, the medical field began utilizing ultrasonic waves for diagnostic purposes, significantly improving non-invasive imaging techniques.
Today, ultrasonic technology is an essential part of various industries. It plays a significant role in fields such as medicine, where it aids in diagnostics through ultrasound imaging. In industrial settings, ultrasonic waves are utilized for non-destructive testing of materials, ensuring product integrity without causing damage. Understanding the historical context of ultrasonic waves highlights their evolution and importance in modern applications.
Physical Properties of Ultrasonic Waves
Understanding the physical properties of ultrasonic waves is crucial for grasping their wide-ranging applications in both medical and industrial fields. These properties define how ultrasound behaves when it interacts with different materials, influencing its effectiveness for various uses. The frequency, wavelength, and speed of sound in different media are pivotal in determining how ultrasonic waves can be harnessed for specific applications. Each property affects how waves travel, how they are detected, and how materials respond to them.
Frequency Range
Ultrasonic waves are characterized by their frequency, which typically exceeds the upper limit of human hearing, set at about 20 kHz. Frequencies can range from 20 kHz to several gigahertz. The frequency chosen for a specific application is essential because it determines the resolution and penetration depth of the ultrasound. Higher frequencies provide better resolution, which is vital for detailed imaging in medical diagnostics. However, they also tend to have shallower penetration. Conversely, lower frequencies can penetrate deeper but sacrifice image resolution.
"The frequency of ultrasonic waves is a fundamental parameter that dictates their application efficiency and effectiveness."
In practice, frequencies like 1 MHz are very common in clinical ultrasound, while a range of 1-10 MHz is used frequently in industrial applications. Each industry carefully selects frequencies based on the characteristics of the materials involved and the nature of the inspection or diagnosis needed.
Wavelength Characteristics
The wavelength of ultrasonic waves is inversely related to their frequency. This means that as frequency increases, wavelength decreases. Wavelength plays a significant role in determining how ultrasonic waves interact with different materials. Shorter wavelengths are beneficial for detecting smaller defects in materials, making them preferable in non-destructive testing.
The formula for calculating wavelength is:
[ \lambda = \fracvf ]\
where ( \lambda ) is the wavelength, ( v ) is the speed of sound in the medium, and ( f ) is the frequency.
In applications involving thickness measurements and flaw detection, knowing the exact wavelength helps technicians interpret results accurately. It also facilitates the choice of transducers that can effectively generate or detect ultrasound with the required sensitivity.
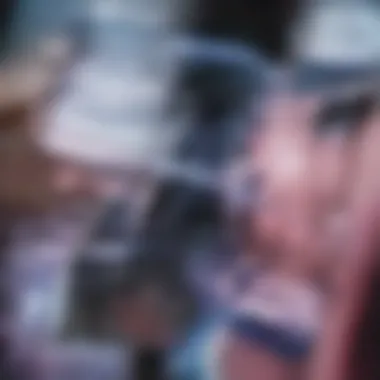
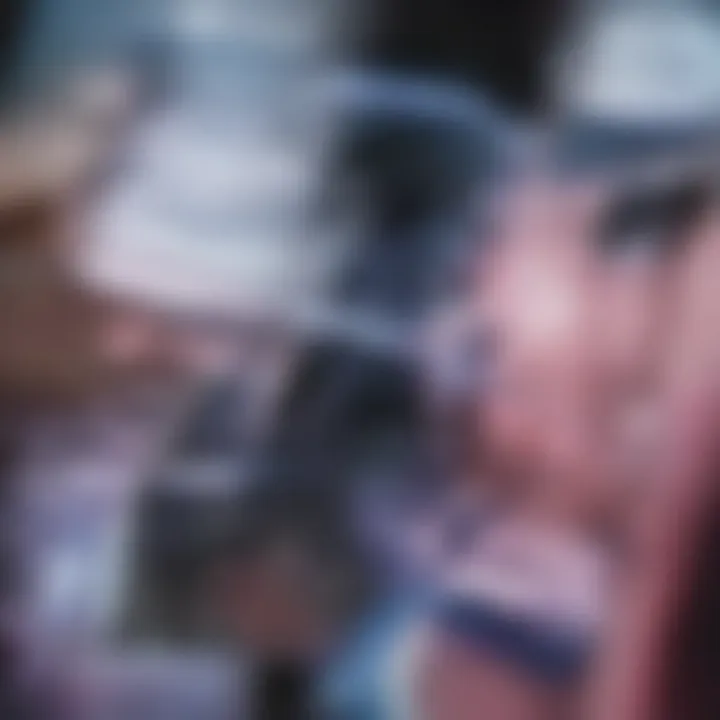
Speed of Sound in Different Media
The speed of sound is another critical property, varying significantly across different materials. Generally, sound travels fastest in solids, slower in liquids, and slowest in gases. For instance, the speed of sound in water is about 1,480 m/s, while in steel, it can reach 5,960 m/s. This difference profoundly affects the propagation of ultrasonic waves and is vital for calculating distances in applications like sonar and medical imaging.
Knowing the speed of sound in a specific material allows for accurate measurements. This property is especially important in medical imaging to accurately determine the distance to organs or structures within the body, facilitating a clearer view of what is being examined.
In summary, understanding the frequency range, wavelength characteristics, and speed of sound in different media is essential for applying ultrasonic technology effectively. Each physical property interlinks with the next, forming the foundation on which practical applications are built.
Generation of Ultrasonic Waves
The generation of ultrasonic waves serves as a fundamental aspect in understanding their numerous applications in various fields. These waves are essential for medical imaging, industrial testing, and material characterization. Their generation involves a set of technologies and principles that ensure their effective creation and deployment. Without efficient generation techniques, the myriad of applications that leverage ultrasonic technologies would not be feasible. Hence, exploring the processes involved in generating these waves is crucial.
Transducer Technologies
Transducers are devices that convert one form of energy into another. In the context of ultrasonic waves, they mainly convert electrical energy into ultrasonic mechanical waves. Two common types of transducers used are piezoelectric transducers and capacitive micromachined ultrasonic transducers (CMUTs).
- Piezoelectric transducers utilize materials like quartz or ceramics. When an electrical signal is applied, they change shape and produce sound waves.
- CMUTs operate on a different principle. They use microfabrication technology to produce tiny membranes that vibrate when voltage is applied, thus generating ultrasonic waves.
Both types of transducers have their unique benefits and considerations. Piezoelectric devices are known for their robustness and high sound pressure levels, while CMUTs can be integrated into more compact systems, making them particularly suitable for advanced applications, such as mobile ultrasound devices.
Piezoelectric Effect
The piezoelectric effect is a phenomenon where certain materials generate an electric charge in response to applied mechanical stress. This property is crucial for the operation of piezoelectric transducers. When these materials are squeezed or distorted, they create an electric field. This enables the conversion of electrical energy to mechanical energy and vice versa.
The piezoelectric effect is pivotal in applications such as:
- Medical imaging: Where it helps in crafting high-resolution ultrasound images.
- Industrial testing: Providing crucial insights into material integrity through non-destructive testing.
Understanding the piezoelectric effect is essential for enhancing the performance and efficiency of ultrasonic devices. Improved materials and designs are continually being studied to optimize this effect, which could lead to advancements in technology and applications.
Laser Ultrasonics
Laser ultrasonics is a sophisticated method of generating ultrasonic waves using laser technology. This technique employs short bursts of laser light, which create rapid heating in a material, causing the generation of sound waves.
Some key advantages of laser ultrasonics include:
- Non-contact: The process does not require physical contact with the material, minimizing the potential for damage.
- High spatial resolution: This method allows for the precise targeting of specific areas, making it superb for material evaluations.
- Broad range of applications: From medical diagnostics to evaluating the structural integrity of materials, this approach is versatile.
The growth of laser ultrasonics represents a significant leap forward in ultrasonic wave generation technology, demonstrating the potential for innovation and improvements in existing and emerging applications.
The generation of ultrasonic waves is a cornerstone of the technology's functionality, directly affecting its efficiency and utility across various industries.
Propagation of Ultrasonic Waves
Understanding how ultrasonic waves propagate is fundamental to their applications. This knowledge helps in optimizing the use of ultrasound in various fields including medical diagnostics and industrial testing. The behavior of these waves in different media can dictate their effectiveness in a given application. Analyzing the propagation characteristics ensures that professionals can predict how ultrasonic waves will interact with materials and environments.
Wave Behavior in Different Media
Ultrasonic waves travel differently depending on the medium they pass through. In gases, such as air, the speed of sound is significantly lower compared to liquids and solids. This is due to the density and elastic properties of the media. For instance, in water, sound travels approximately four times faster than in air.
- In solids, ultrasonic waves can travel very efficiently. The molecular structure allows for quicker transmission of sound.
- Changes in temperature, pressure, and density also affect speed.
This behavior is critical when selecting the appropriate medium for any ultrasonic application. In some cases, ultrasound might be more favorable in water for imaging compared to air.
Attenuation and Reflection
Attenuation refers to the decrease in amplitude and intensity of ultrasonic waves as they move through a medium. Various factors contribute to this, including.
- Viscosity: Higher viscosity leads to greater energy loss.
- Frequency: Higher frequencies experience more attenuation.
- Medium composition: Different materials absorb sound differently.
Reflection occurs when ultrasonic waves encounter a boundary between two different media. The degree of reflection is influenced by the impedance of both materials. When there is a large impedance mismatch, more energy is reflected. This principle is utilized in ultrasound imaging. For example, the detection of tissues in the body occurs through reflected waves, which create images based on the different acoustic properties of the tissues.
Understanding the balance between attenuation and reflection is essential for improving the clarity and quality of ultrasonic images.
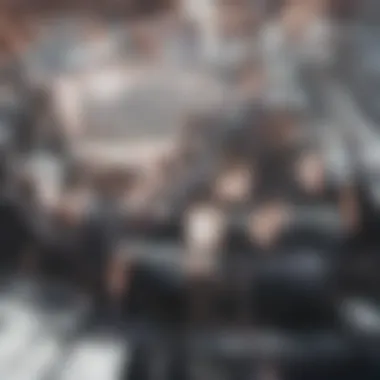
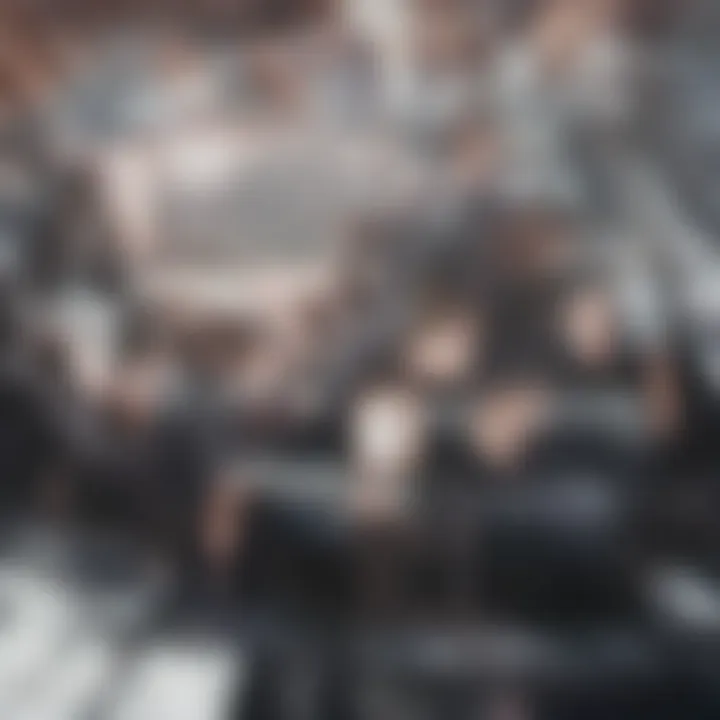
Refraction and Diffraction
Refraction happens when ultrasonic waves change direction as they pass through different media. The degree of bending depends on the angle of incidence as well as the properties of the media involved. The change in speed when passing from one medium to another leads to a redirection of the sound waves. This is important in applications such as focusing sound beams in medical imaging.
On the other hand, diffraction relates to the spreading of ultrasonic waves when they encounter an obstacle or a small aperture. The phenomenon of diffraction can affect resolution in imaging applications. Understanding diffraction helps in designing better systems that minimize resolution loss, thereby enhancing the quality of ultrasonic inspections and assessments.
Applications of Ultrasonic Waves
Ultrasonic waves have a diverse range of applications that leverage their unique properties. Their ability to penetrate materials and provide insights into internal structures is invaluable. Industries and fields such as medicine, manufacturing, and cleaning rely on these waves for various significant purposes. Understanding these applications is essential as it highlights both the economic benefits and technological advancements that ultrasound enables. The significance of ultrasonic applications extends beyond mere convenience, as they often ensure quality and safety in processes.
Medical Imaging and Diagnostics
In the realm of medical imaging, ultrasonic technology plays a crucial role. Ultrasound imaging, commonly known as sonography, uses ultrasonic waves to produce images of soft tissues, organs, and blood flow. This non-invasive technique allows for real-time imaging without the risks associated with ionizing radiation found in X-rays. Medical practitioners utilize ultrasound to monitor fetal development, diagnose conditions, and guide biopsies, making it a fundamental tool in prenatal care and many other medical applications.
One of the primary advantages of ultrasound is its portability, allowing for bedside imaging and reducing the need for elaborate imaging equipment. Additionally, ultrasound aids in detecting conditions such as gallstones, liver diseases, and cardiac issues. With continuous advancements in imaging techniques and software, the clarity and utility of ultrasound images have improved significantly, enhancing diagnostic accuracy.
Industrial Non-Destructive Testing
Ultrasonic waves are also pivotal in industrial applications, notably in non-destructive testing (NDT). NDT is a crucial process in ensuring the integrity and safety of materials and structures without causing any damage. Ultrasonic testing can detect flaws in a variety of materials, such as metals and composites, thereby preventing potential failures in critical components like pipelines, bridges, and aircraft.
The process involves sending ultrasonic waves into the material. When these waves encounter a defect, they reflect back to the transducer, revealing the presence and location of any irregularities. This method is both efficient and cost-effective, providing quick assessments that are essential for maintaining safety standards in industries.
Cleaning and Sterilization
The cleaning capabilities of ultrasonic waves are utilized in various sectors, particularly in medical and laboratory settings. Ultrasonic cleaning systems generate specific high-frequency sound waves that create cavitation bubbles in a cleaning solution. These bubbles collapse violently, generating high forces that dislodge contaminants from surfaces. This process is effective for cleaning intricate items that are difficult to clean by conventional means, such as surgical instruments or electronic components.
Moreover, the efficacy of ultrasonic cleaning extends to sterilization processes. By thoroughly cleaning items before sterilization, the effectiveness of sterilization methods, such as autoclaving, is enhanced. Therefore, ultrasonic cleaning not only ensures cleanliness but also plays a vital role in infection control practices.
Ultrasonic Welding
Ultrasonic welding is another noteworthy application of ultrasonic waves, particularly in the manufacturing sector. This technique joins materials, especially thermoplastics, by using high-frequency ultrasonic vibrations. The vibrations create localized heat at the junction of the materials being welded, achieving solid-phase bonding without the need for traditional heat sources or adhesives.
The advantages of ultrasonic welding include its speed and efficiency, resulting in lower energy consumption and reduced material waste. Additionally, this method is highly adaptable to automation, making it suitable for mass production. Industries such as automotive and electronics extensively use ultrasonic welding techniques to assemble components, ensuring robust and reliable joints in their products.
"Ultrasonic waves provide innovative solutions across various fields, demonstrating their fundamental role in modern technology."
These applications underline the versatility and utility of ultrasonic waves. They not only enhance efficiencies but also contribute to safety and quality in multiple industries. As technology continues to evolve, so too will the innovations surrounding ultrasonic applications, amplifying their impact on society.
Safety Considerations
Safety is paramount when working with ultrasonic technology. While it brings many benefits in various fields, understanding the associated risks is essential for effective and safe application. Failing to consider safety aspects can lead to health risks for operators and unintended damage to materials or equipment. This section discusses potential hazards related to ultrasonic waves and the regulatory frameworks that guide their safe use.
Potential Hazards of Ultrasonics
Ultrasonic waves, while largely safe, can pose hazards if not managed properly. Some of the key hazards include:
- Hearing Damage: Exposed individuals can experience hearing loss if they are around ultrasound equipment operating at high volumes. The intensity and frequency of sound can affect sensitive hearing mechanisms.
- Physical Effects: High-intensity ultrasonic fields can lead to thermal and mechanical effects in biological tissues. This can cause heating or localized damage, particularly in medical applications.
- Interference with Equipment: Ultrasound can cause interference with electronic devices, potentially impacting diagnostics or procedural equipment in sensitive environments like hospitals.
It is important to implement sufficient measures to mitigate these hazards. Proper training, equipment maintenance, and use of personal protective equipment should be standard practice to ensure the safety of personnel involved in ultrasonic operations.
"Awareness and rigour in applying safety practices are vital for safe ultrasonics operation."
Regulatory Standards and Guidelines
Various organizations have set guidelines for the safe use of ultrasonic waves. These standards aim to protect users and to provide a framework that manufacturers and operators can follow. Here are some of the prominent standards:
- Occupational Safety and Health Administration (OSHA): OSHA provides guidelines for permissible noise levels, which also applies to ultrasonic exposure in workplace environments. Businesses must adhere to these thresholds to safeguard employee health.
- International Electrotechnical Commission (IEC): The IEC lays out standards for ultrasonic equipment, ensuring devices are designed with safety mechanisms that limit exposure limits.
- American National Standards Institute (ANSI): ANSI guidelines cover training, operation, and maintenance of ultrasonic equipment to reduce risks related to sound exposure.
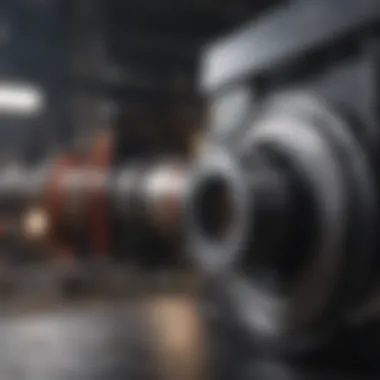
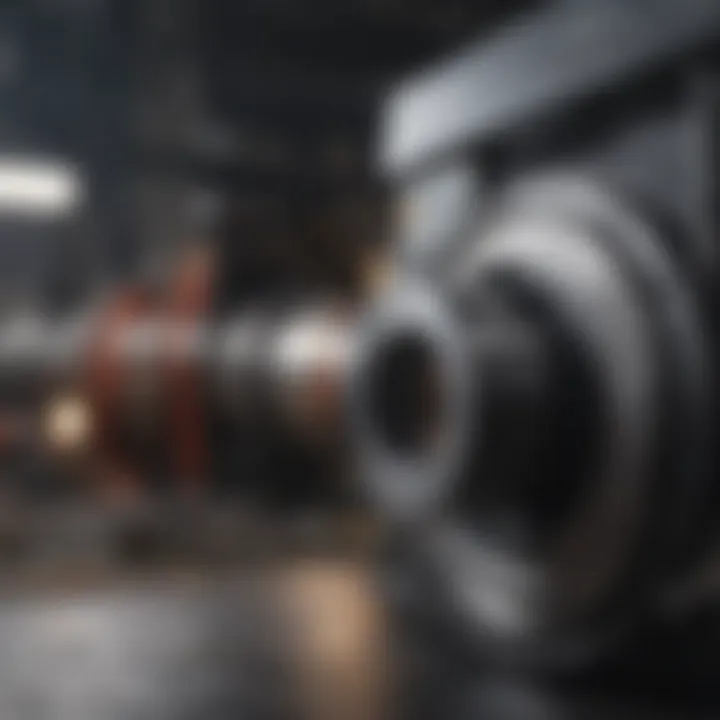
By adhering to these regulatory standards, industries can help ensure the safe use of ultrasonic technology. It minimizes health risks and fosters a culture of safety in associated fields, allowing the benefits of ultrasonics to be harnessed without compromising well-being.
Limitations of Ultrasonic Technology
Understanding the limitations of ultrasonic technology is crucial, especially when applying it in diverse fields such as medicine and industry. While ultrasonic waves hold great promise in various applications, they are not without challenges. Knowledge of these limitations aids in the development of better techniques and potential innovations.
Material Limitations
One primary limitation of ultrasonic technology is its dependence on the material properties of the medium through which the waves travel. Ultrasonic waves behave differently based on factors such as density, elasticity, and microstructure of the material. For example, certain materials can absorb ultrasonic energy, leading to ineffective signal transmission. This absorption can significantly limit the range and clarity of the signals, particularly in softer materials like plastics, which may hinder their use in certain testing scenarios.
In diagnostic imaging, if the tissue being examined is too dense or heterogeneous, the ultrasonic waves may not penetrate adequately. Consequently, this can result in insufficient data for accurate diagnoses in clinical settings. Moreover, interfaces between different materials can introduce further complications due to varying acoustic impedance. When waves cross these interfaces, they can reflect or refract in unexpected ways, challenging the reliability of readings.
Resolution Challenges
Resolution is another significant concern when it comes to ultrasonic technology. The ability to discern small features relies on the wavelength of the ultrasonic waves used. High-frequency waves offer better resolution but are more susceptible to attenuation, especially in denser materials. Therefore, achieving the right balance between frequency and penetration depth proves to be difficult.
For instance, in medical imaging, higher frequencies can provide better detail for soft tissue evaluation. However, they may not effectively penetrate denser tissues or bone, compromising the overall diagnostic capability. This presents a dilemma for healthcare professionals seeking to utilize ultrasonic techniques effectively while managing limitations.
In industrial applications, higher resolution can also lead to better defect detection. Yet, this is complicated by the necessity for a suitable frequency that can penetrate various materials without excessive loss of energy. As a result, industries often need tailored approaches that consider specific material contexts and the requirement for clarity in image or signal data.
The complexities surrounding material limitations and resolution challenges emphasize the need for ongoing research and development in ultrasonic technology.
Ultimately, acknowledging these limitations helps professionals understand the boundaries of ultrasonic applications and encourages innovation to overcome them.
Future Directions in Ultrasonics Research
The field of ultrasonics is advancing rapidly, spurred by the need for innovative solutions across multiple industries. Understanding the future directions in ultrasonics research is crucial for leveraging the full potential of ultrasonic technology. This entails exploring emerging technologies that could redefine applications and addressing challenges that currently limit its effectiveness.
Emerging Technologies
In recent years, several emerging technologies have begun to shape the landscape of ultrasonics. Researchers are focusing on developing more sophisticated transdusers that enhance the sensitivity and efficiency of ultrasonic devices. For example, advancements in nanomaterials are being integrated into transducers to improve their performance at a micro-scale level. This could revolutionize medical imaging and allow for more precise diagnostics.
Additionally, the integration of machine learning and artificial intelligence with ultrasonic systems is gaining traction. These technologies can optimize signal processing and data interpretation, making it possible to extract more meaningful information from ultrasonic data. For instance, AI can analyze complex patterns in imaging data, leading to improved diagnostic accuracy in healthcare applications.
Another noteworthy technology is 3D ultrasound, which provides a spatial understanding of structures. As this technology matures, it has the potential to enhance fields like obstetrics and cardiology by offering detailed visualizations that can improve treatment strategies.
Innovative Applications
Innovative applications of ultrasonic technology continue to emerge as industries identify new challenges to address. In the medical field, ultrasonic waves are being employed not just for imaging but also for therapeutic uses. High-Intensity Focused Ultrasound (HIFU) is a technique that uses ultrasound energy to destroy tissue in specific locations without harming the surrounding area. Applications are increasingly seen in treating tumors and fibroids.
Moreover, in material science, researchers are utilizing ultrasonic waves for nanofabrication processes, creating materials with superior properties. This has implications for electronics and aerospace industries, where lightweight and strong materials are critical.
The food industry also stands to benefit from ultrasonic technology. Ultrasonic waves are being explored for food preservation and extraction of nutrients, which promise to enhance food quality while extending shelf life. This shows the versatile nature of ultrasonic applications, moving beyond conventional sectors into everyday consumer products.
"Ultrasonic technology possesses unique capabilities that are still being discovered, reinforcing its relevance in future technological advancements."
By critically examining and investing in these future directions, stakeholders can propel the field of ultrasonics into a new era of possibilities.
End
Understanding ultrasonic waves is crucial for multiple sectors, especially in medicine and industry. The exploration of their properties reveals a great deal about how these waves can be utilized for various applications, from imaging to testing materials. As outlined, ultrasonic technology generates significant advantages, such as non-destructive testing which safeguards material integrity without compromising their functionality.
Summary of Key Points
In this article, several key points have been discussed:
- Definition and Nature: Ultrasonic waves are sound waves with frequencies above the human hearing range, typically above 20 kHz.
- Generation and Propagation: Various methods of producing ultrasonic waves were explored, including the piezoelectric effect and laser ultrasonics. The propagation behaviors of these waves through different media were also reviewed.
- Wide Applications: The applications are diverse and impactful, spanning medical imaging, industrial non-destructive testing, cleaning solutions, and welding technologies.
- Limitations and Safety: While beneficial, certain limitations exist, such as material restrictions and less than ideal resolution in some scenarios. Safety considerations regarding the use of ultrasonic devices are also essential to address.
- Future Directions: Emerging technologies and innovative applications signal a promising future for ultrasonics research.
Implications for Future Applications
The implications of ultrasonic technology extend into numerous domains. As the field of ultrasonics continues to advance, several developments can be anticipated:
- Medical Advancements: New techniques in medical imaging could evolve, improving diagnostic capabilities and patient outcomes.
- Industrial Improvements: Enhanced non-destructive testing methods can lead to safer structures and products by identifying flaws before they yield critical failures.
- Research Innovations: Ongoing research will likely explore novel applications, such as ultrasonic therapies for health treatment or advancements in materials science.
In summary, as technology progresses and understanding deepens, ultrasonic waves will continue to have a transformative impact across various disciplines. Their properties and applications resonate not just within technical fields but also in broader societal contexts.