Exploring the Fundamental Elements of Chemistry
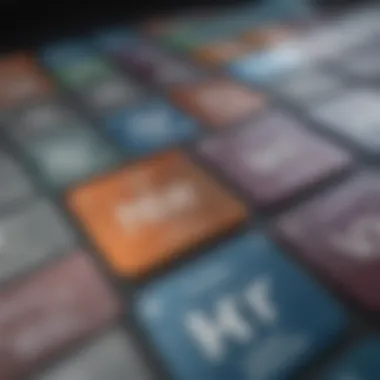
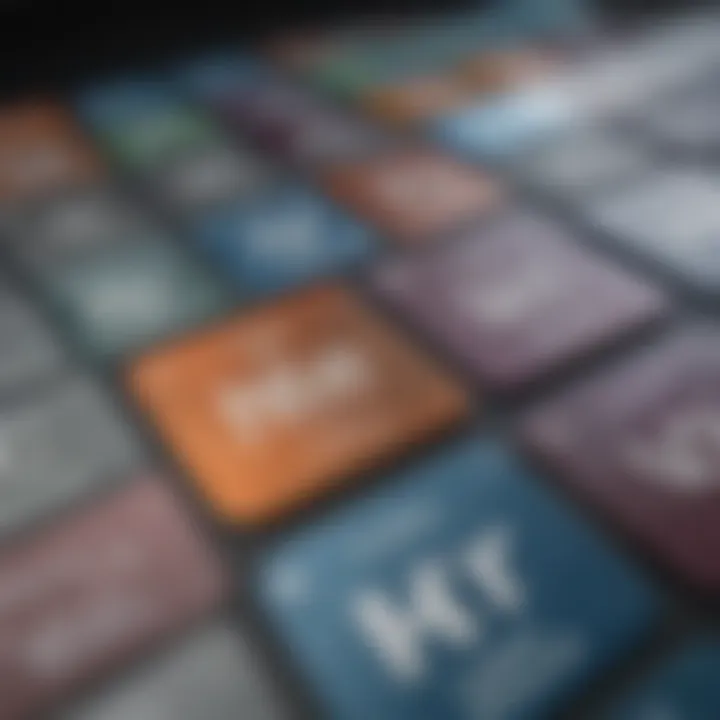
Intro
Chemistry is a core science that explores the properties, composition, and transformations of matter. Central to this discipline are the elements, which act as the building blocks for all substances. Understanding these elements is crucial for students, researchers, educators, and professionals involved in various scientific fields. This article aims to provide a comprehensive overview of the fundamental elements of chemistry, examining their classifications, properties, and significance in both theoretical and practical applications.
The periodic table serves as a vital framework in chemistry. It systematically organizes elements based on their atomic number, electron configurations, and recurring chemical properties. Each element has unique characteristics that define its behavior and role in reactions. By grasping these foundational aspects, one can appreciate the broader implications in materials science, pharmacology, and environmental chemistry.
In this exploration, the elemental characteristics will be dissected, illustrating their interactions in compounds and highlighting their multifaceted applications. This structured analysis will not only clarify how elements operate individually but also how they contribute to advanced technologies and environmental solutions.
Intro to Chemical Elements
Understanding chemical elements serves as the cornerstone of chemistry. They are pure substances that consist of one type of atom and define the phase of matter. Chemical elements have unique properties that dictate how they interact with one another. This article aims to provide a thorough exploration of these elements and their critical roles in various scientific domains. By dissecting the nature of elements, their historical backdrop, and their significance, this text will elucidate the foundational principles of chemistry.
The Nature of Elements
Chemical elements are categorized in the periodic table, each with its own distinct atomic number, symbol, and mass. The properties of these elements arise from their atomic structure. For example, hydrogen, the lightest element, has one proton in its nucleus, leading to its gaseous state at room temperature. On the contrary, heavier elements like lead exhibit metallic characteristics at room temperature due to their complex atomic structures.
Elements can be broadly classified into metals, nonmetals, and metalloids, each group showcasing a unique set of characteristics.
- Metals are typically conductive, malleable, and lustrous.
- Nonmetals can exist in various states and often display poor conductivity.
- Metalloids possess properties that are intermediate, making them ideal for various applications, such as semiconductors in electronics.
The interplay between these elements leads to the formation of compounds, further emphasizing their importance in the vast realm of chemistry.
Historical Context and Discoveries
The journey of understanding chemical elements traces back to ancient civilizations. Early thinkers proposed elemental theories, laying groundwork for more systematic study. The Greeks, for instance, categorized matter into four fundamental elements: earth, water, air, and fire.
It wasn’t until the late 17th and early 18th centuries that the concept of a chemical element began to take shape. Notable scientists like Antoine Lavoisier played pivotal roles in defining elements in a more precise manner. Lavoisier's efforts culminated in the recognition of oxygen and hydrogen as elements rather than compounds.
The development of the periodic table by Dmitri Mendeleev in 1869 marked a watershed moment. Mendeleev organized elements in a way that showcased periodicity in chemical properties, fundamentally changing the course of chemistry. His predictions led to the identification of new elements, cementing the importance of periodicity in understanding element behavior.
Furthermore, advancements in technology and research have continued to enhance our understanding of chemical elements, including the discovery of synthetic elements in laboratories, broadening the periodic table. Each discovery enriches the narrative of chemistry, reminding us of the dynamic nature of science and the ongoing quest for knowledge.
The Periodic Table of Elements
The periodic table serves as a cornerstone in the study of chemistry. It is not simply a chart of elements; it provides essential insight into the properties and relationships among various substances. Understanding the periodic table allows scientists, educators, and students to predict the behavior of elements in different chemical reactions and applications. Furthermore, it serves as a vital communication tool in the scientific community, facilitating a common language for discussing elemental properties.
Structure and Layout
The periodic table is organized into rows called periods and columns known as groups. Each row corresponds to the number of electron shells surrounding an atom's nucleus. For instance, the first period contains elements with one electron shell, whereas the second period has elements with two. The general layout facilitates a systematic approach to understanding trends in element properties.
The table itself is divided into several sections. On the left side, we commonly find metals, which tend to lose electrons easily, resulting in positive ions. Nonmetals occupy the right side. These elements tend to gain electrons during interactions. Metalloids, possessing characteristics of both metals and nonmetals, are located along the dividing line. This structure helps to navigate through the vast array of chemical elements efficiently.
Periods and Groups
Elements are arranged in groups, which are vertical columns on the table. Each group contains elements with similar chemical properties. For example, the alkali metals in Group 1 are highly reactive and have one valence electron, while the noble gases in Group 18 are largely inert and have full electron shells.
Apart from groups, periods play an equally significant role. Moving down a group, elements display a gradation in properties. This allows for predictive capabilities regarding reactivity, atomic size, and electronegativity.
"The periodic table organizes elements in a way that reflects their atomic structure; this organization reveals trends in element behaviors, which can be applied in various scientific fields."
Types of Elements
The topic of types of elements is crucial to understanding the broader scope of chemistry. Elements are classified mainly into three categories: metals, nonmetals, and metalloids. Each category possesses distinct properties and plays vital roles in various applications. Understanding these differences helps in grasping how elements interact and combine to form compounds, which is fundamental in both science and industry. The classifications of elements guide chemists in predicting behaviors, reactivities, and the possible uses of different substances.
Metals
Properties of Metals
Metals are generally characterized by high electrical and thermal conductivity, malleability, ductility, and lustrous appearance. These properties make metals a focal point in many applications, from construction to electronics. For instance, copper, known for its excellent conductivity, is extensively utilized in electrical wiring.
A significant property of metals is strength. They are often capable of withstanding considerable force without deformation. This characteristic makes them beneficial for creating structural materials in buildings and infrastructure. However, metals can also be prone to corrosion when exposed to moisture, which necessitates protective coatings or alloys.
Common Uses of Metals
The common uses of metals span several industries. In manufacturing, iron and aluminum are integral for producing various tools, machinery, and vehicles. Gold and silver are used in jewelry due to their aesthetic appeal and resistance to tarnish. Additionally, metals like titanium find applications in aerospace due to their high strength-to-weight ratio.
These applications demonstrate the versatility of metals, but one unique feature is their recyclability. Many metals can be melted down and reused without degrading their properties, making them an environmentally friendly choice. The durability of metals contributes greatly to their widespread use across many sectors.
Nonmetals
Properties of Nonmetals
Nonmetals tend to have lower densities and are generally poor conductors of heat and electricity. This distinct property results in their use as insulators in various applications. For example, sulfur is an essential nonmetal that is crucial in the manufacturing of fertilizers and pesticides. The nonmetal category includes gases like oxygen and nitrogen, both essential for life.
A significant characteristic of nonmetals is their ability to form covalent bonds. They often share electrons with other nonmetals to create stable molecules. This feature makes them essential in organic chemistry, where nonmetals like carbon, hydrogen, and oxygen combine to form organic compounds.
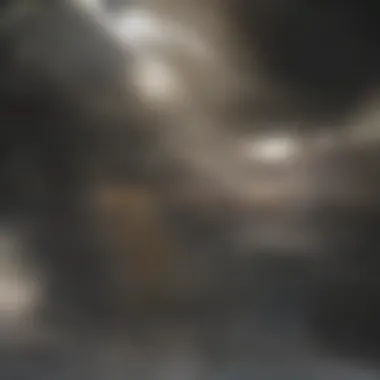
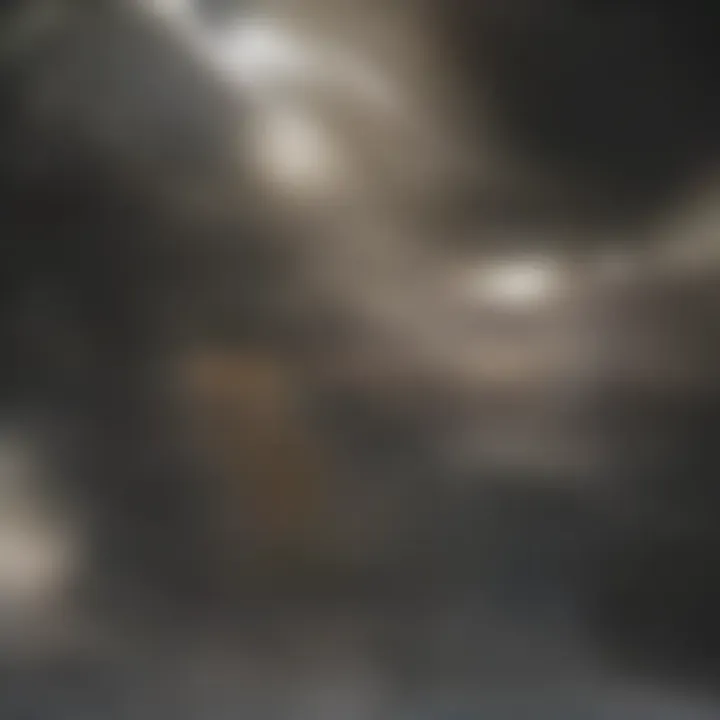
Common Uses of Nonmetals
Nonmetals have diverse applications, notably in the fields of chemistry and medicine. For instance, chlorine serves as a disinfectant in water treatment plants. Carbon, in its various forms, is used in steel production and as a foundational element in organic life.
The versatility of nonmetals is notable. However, a unique feature is their variable reactivity; nonmetals can react in different ways under various conditions. This variability poses certain challenges but also results in a wide range of useful compounds.
Metalloids
Properties of Metalloids
Metalloids possess properties intermediate between metals and nonmetals. They are semiconductors, meaning they can conduct electricity better than nonmetals but not as well as metals. Elements like silicon are critical in electronics due to their semi-conductive properties. Their ability to conduct electricity based on temperature changes makes them versatile and valuable in various applications.
A key characteristic of metalloids is their ability to form alloys with metals. This property allows for the enhancement of other materials, giving a range of improved characteristics. While they provide benefits in terms of electronic applications, metalloids like arsenic also pose hazards and must be handled with care.
Applications of Metalloids
Common applications of metalloids primarily focus on their semiconductor properties. Silicon is extensively used in the production of computer chips and solar cells. Other metalloids like germanium also find uses in fiber optics. The high demand for these technologies underscores the importance of metalloids in modern developments.
Moreover, metalloids' unique properties allow them to serve in niche areas like glasses and alloys, making them valuable in such applications. However, one disadvantage can be their potential toxicity, necessitating careful management when utilized in industry.
Understanding the types of elements and their roles in chemistry is essential. This knowledge is pivotal not only in laboratory settings but also in various practical applications that define our modern world.
Essential Elements for Life
Understanding the elements that are essential for life is crucial in the study of chemistry and biology. These elements are classified into two groups: macronutrients and micronutrients. Macronutrients are required in larger quantities and include elements such as carbon, hydrogen, nitrogen, and oxygen. Micronutrients are needed in smaller amounts but are equally vital. This section will detail these elements, their specific roles, and why they are indispensable for life.
Macronutrients
Carbon
Carbon is often termed the backbone of life. It possesses a unique ability to form four covalent bonds, which allows it to create a vast diversity of organic compounds. This versatility facilitates the formation of complex molecules such as proteins, nucleic acids, lipids, and carbohydrates, all of which are fundamental to biological functions.
The key characteristic of carbon is its tetravalency, which enables it to link with many other elements, including hydrogen, nitrogen, and oxygen. A benefit of carbon is its central role in the chemical structure of living organisms. Additionally, its ability to participate in stable bonds leads to a wide variation of organic molecules. However, carbon can create compounds that are not always stable under certain conditions, leading to considerations in environmental chemistry and organic synthesis.
Hydrogen
Hydrogen is the simplest and most abundant element in the universe, crucial for the formation of water and organic compounds. Its role in life is primarily as a part of water molecules and organic compounds, where it exhibits a +1 oxidation state. Hydrogen's presence in compounds enables various chemical reactions that sustain life.
The characteristic of hydrogen is its single electron, which allows it to bond easily with other elements. This property is beneficial for biological processes, such as energy transfer during cellular respiration. The unique feature of hydrogen is its involvement in forming acids and bases, impacting pH balance in living organisms. However, hydrogen can also react with various elements in potentially hazardous ways.
Nitrogen
Nitrogen is an essential element in amino acids, the building blocks of proteins, and nucleotides, which form DNA and RNA molecules. The nitrogen cycle is critical for recycling nitrogen through ecosystems, making it available for biological use. This element plays a vital role in the synthesis of biomolecules essential for life.
Nitrogen’s main attribute is its ability to form stable bonds, allowing it to create various functional groups within molecules. This characteristic is beneficial for maintaining the integrity of genetic information. However, while nitrogen is abundant in the atmosphere, most organisms cannot use it directly. Therefore, nitrogen fixation processes are necessary to convert it into usable forms.
Oxygen
Oxygen is essential for cellular respiration, a process crucial for producing energy in living cells. It is also a part of water, making it vital to life on Earth. Oxygen's electronegativity leads to the formation of polar molecules, which have significant biological implications.
One key characteristic of oxygen is its role in oxidation reactions, where it gains electrons. This is beneficial in energy production processes. The unique feature of oxygen in biological systems is its ability to form reactive species, which can be harmful in higher concentrations. Thus, while necessary for life, it requires careful management within biological systems to prevent oxidative stress.
Micronutrients
Iron
Iron is a vital trace element that serves crucial roles in various biological functions, including oxygen transport in the blood. Hemoglobin, found in red blood cells, relies on iron to bind oxygen. Iron is also critical in electron transport chains in cellular respiration, making it essential for energy production.
The characteristic of iron is its ability to exist in multiple oxidation states, which is advantageous for its role in redox reactions within cells. However, excess iron can lead to toxicity, thus necessitating regulated levels in organisms.
Copper
Copper is essential for several enzymatic reactions within living organisms. It plays a significant role in iron metabolism and the formation of connective tissue. Copper is crucial in producing energy via aerobic respiration.
One key characteristic of copper is its involvement in redox processes, essential for maintaining cellular functions. However, while it is beneficial, its deficiency can lead to anemia and cardiovascular issues. Conversely, excess copper can cause toxicity.
Zinc
Zinc plays a crucial role in the function of numerous enzymes and the immune system. It is pivotal for maintaining cellular structure and function, as it stabilizes proteins and assists in DNA synthesis and repair.
The characteristic of zinc is its ability to act as a structural and catalytic component in various enzymes. This makes it indispensable for numerous biochemical processes within the body. Its unique feature is that it cannot be stored in the body effectively, necessitating regular dietary intake to maintain optimal levels.
In summary, the essential elements for life—macronutrients and micronutrients—are foundational to the chemistry of living organisms. Their specific roles and attributes highlight how life is not just a chemical phenomenon but a complex interaction of elemental forces.
To further explore these elements, readers can refer to educational resources like Wikipedia, Britannica, and discussions on Reddit.
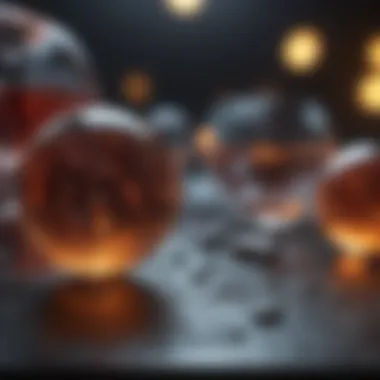
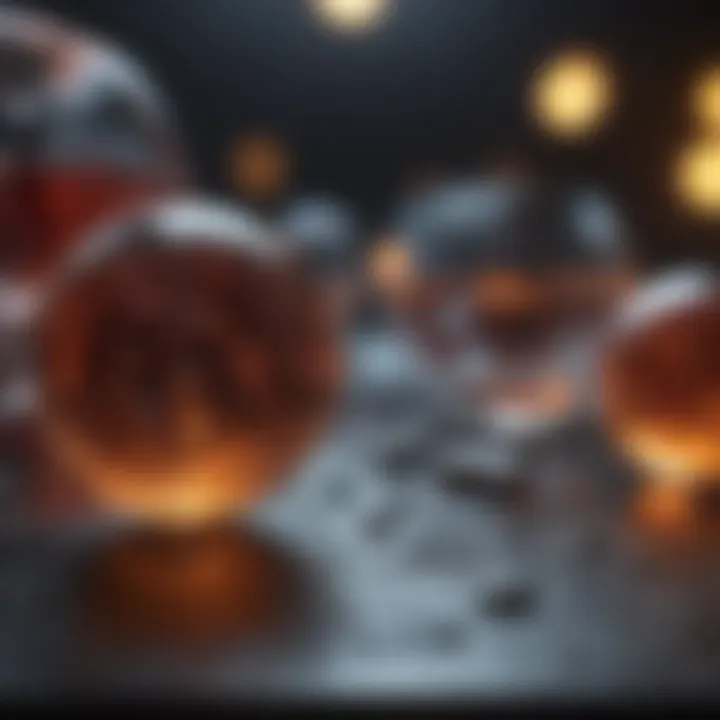
Understanding these essentials can deepen our grasp of biological processes and highlight the delicate balance they maintain in life.
Chemical Bonding and Element Interaction
Understanding chemical bonding is crucial in the study of chemistry. It lays the foundation for how elements interact, form compounds, and contribute to the material world around us. Chemical bonding describes the attractive forces between atoms, leading to the formation of stable arrangements, which are essential in all chemical processes. The types of bonds formed between elements determine the properties of the resulting compounds and, therefore, play a significant role in various applications across fields such as materials science, medicine, and environmental chemistry.
Ionic Bonds
Ionic bonds occur when electrons are transferred from one atom to another. This transfer typically happens between metals and nonmetals, resulting in the formation of positively charged cations and negatively charged anions. The electrostatic attraction between these oppositely charged ions holds them together in a lattice structure, which gives ionic compounds their characteristic properties, such as high melting and boiling points, and electrical conductivity when dissolved in water.
Common table salt, sodium chloride, is a prime example of an ionic compound. Sodium donates an electron to chlorine, resulting in the formation of Na⁺ and Cl⁻ ions. This interaction illustrates the fundamental nature of ionic bonds in establishing stable compounds that are common in everyday life and essential in various chemical reactions.
Covalent Bonds
Covalent bonds, on the other hand, are formed when two atoms share one or more pairs of electrons. This type of bonding typically occurs between nonmetals. The shared electrons allow each atom to attain a more stable electronic configuration. Diatomic molecules like hydrogen (H₂) and oxygen (O₂) demonstrate covalent bonding through sharing pairs of electrons, resulting in strong bonds that are vital for the structure of many biological molecules.
The strength of covalent bonds can vary. For instance, single bonds involve one shared pair of electrons, while double or triple bonds involve two or three pairs, respectively. This variability in bonding leads to different molecular structures and properties, influencing everything from the boiling point of a substance to its reactivity.
Metallic Bonds
Metallic bonds are a unique form of chemical bonding that occurs between metal atoms. In this case, electrons are not shared or transferred but are instead delocalized among a lattice of metal cations. This 'sea of electrons' allows metals to conduct electricity and heat efficiently, providing durability and malleability, which are essential properties for metallic materials.
The characteristics of metallic bonds explain why metals can be easily shaped and stretched. For example, copper, widely used in electrical wiring, maintains conductivity due to its metallic bonding, which facilitates the flow of electrons. Additionally, metals tend to possess luster and high melting points, which are directly linked to the strength of their metallic bonds.
Understanding these bonding types provides insights into the behavior of elements and their compounds, shaping our knowledge of chemistry.
The Role of Elements in Compounds
The role of elements in compounds is crucial to understanding chemistry. Elements combine to form compounds, which are the building blocks of both biological and inorganic systems. This section highlights several key aspects regarding how elements interact and the resulting impact on various scientific disciplines.
Chemical compounds embody the distinctive properties of the constituent elements while displaying new characteristics through their interactions. Knowledge of these interactions aids in numerous real-world applications, ranging from pharmaceuticals to material science. Given the relevance of this topic, insights into how elements form compounds will illuminate the complexities and significance of chemistry in our daily lives.
Defining Chemical Compounds
Chemical compounds are substances formed when two or more elements chemically bond. This occurs through different types of bonds, such as ionic or covalent bonds, which dictate the properties of the resultant compound. The arrangement of elements within a compound defines not just its chemical behavior but also its physical properties. For example, water (H₂O) is a compound that displays unique properties not evident in its constituent elements, hydrogen and oxygen. Understanding how compounds are formed gives fundamental insight into chemical reactions, stoichiometry, and various applications in chemistry.
Types of Chemical Compounds
The classification of chemical compounds can be broadly categorized into organic and inorganic compounds. Each type exhibits unique characteristics, contributions, and roles in scientific research and industry.
Organic Compounds
Organic compounds primarily consist of carbon atoms bonded with hydrogen, oxygen, nitrogen, and other elements. The key characteristic of organic compounds is their distinct carbon backbone, which allows for extensive variability in structure and function. These compounds are essential in biochemistry and form the basis of all known life. Molecules such as carbohydrates, proteins, and lipids are all organic compounds that play vital roles in biological processes.
One significant aspect of organic compounds is their versatility. The various functional groups attached to carbon skeletons allow for an array of chemical reactions, making them crucial in pharmaceuticals, agricultural chemicals, and plastics. However, organic compounds may often present challenges, such as stability issues or environmental consequences, that require careful consideration in research and industry.
Inorganic Compounds
In contrast to organic compounds, inorganic compounds generally do not contain carbon-to-carbon bonds. Many inorganic compounds consist of metals, minerals, and a range of elements such as oxygen and nitrogen. A defining characteristic of inorganic compounds is their ability to exhibit diverse properties depending on the elements involved. For example, table salt (sodium chloride) is ionic, while water is covalent. These unique attributes contribute to their importance in fields like materials science, catalysis, and medicine.
Inorganic compounds tend to be more stable than their organic counterparts and often serve as catalysts in chemical reactions. They are broadly utilized in industrial processes, including the production of fertilizers and glass, as well as in the synthesis of chemicals. Nonetheless, some inorganic compounds can be harmful or toxic, necessitating strict handling protocols.
Understanding both organic and inorganic compounds is essential for grasping the broader implications of elemental chemistry. Each category serves fundamental roles in various scientific disciplines and industries.
Advanced Concepts in Elemental Chemistry
The realm of chemistry extends beyond the basic understanding of elements and their properties. Advanced concepts in elemental chemistry provide a deeper insight into the behavior and application of specific elements. These concepts are crucial not only for academic discourse but also for practical implications in various fields such as medicine, technology, and environmental sciences. Understanding isotopes and radioactive elements grants researchers and professionals the tools needed to push boundaries in their respective fields.
Isotopes and Their Applications
Isotopes are variations of a chemical element that differ in the number of neutrons within their atomic nuclei. This distinction results in different atomic masses. The most well-known isotopes are Carbon-12 and Carbon-14. Carbon-14, for instance, is a radioactive isotope used extensively in radiocarbon dating, a technique vital for archaeologists to determine the age of ancient organic materials.
The applications of isotopes circle various domains:
- Medical Uses: In medicine, isotopes play a significant role. Isotopes such as Technetium-99m are employed in medical imaging, providing critical information about the functioning of organs. This has transformed diagnostic procedures, allowing for less invasive investigations.
- Agricultural Implements: Isotopes aid in understanding soil dynamics and plant nutrition, driving advancements in sustainable agriculture.
- Industrial Applications: In industries, stable isotopes enhance the precision of various processes, from quality control to tracing material flows.
Isotopes also enable scientists to track biological processes in real-time. They act as tags, offering insight into metabolic pathways and uncovering mechanisms of disease. Because of their ability to reveal hidden relationships, isotopes remain a focal point of research in fields like biochemistry and pharmacology.
"Understanding isotopes transforms our grasp of elements from merely theoretical concepts to powerful tools for innovation."
Radioactive Elements
Radioactive elements possess unstable nuclei that decay over time, emitting radiation in the process. This radiation can be characterized as alpha particles, beta particles, or gamma rays. The study of radioactive elements is vital for several reasons. They contribute to both the natural environment and significant technological advancements.
Some well-known radioactive elements include Uranium, Thorium, and Radon. Their applications vary widely:
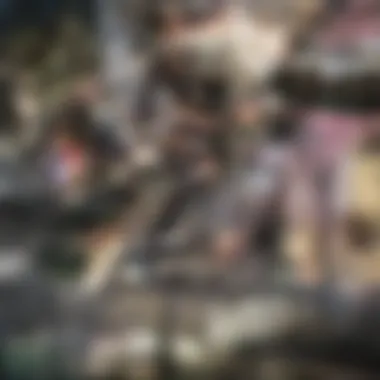
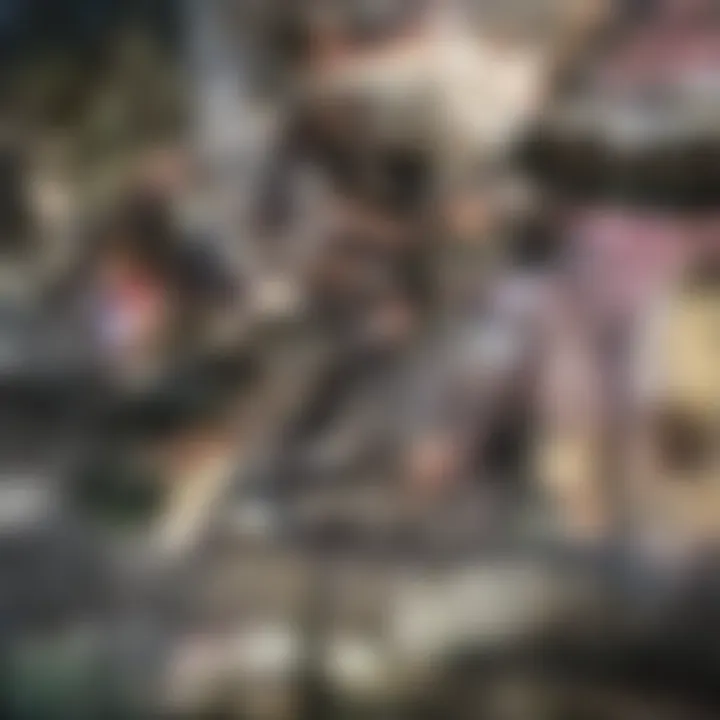
- Energy Production: Uranium fuels nuclear reactors, providing a substantial amount of energy while minimizing greenhouse gas emissions. This characteristic is especially pertinent as the world shifts toward sustainable energy solutions.
- Medical Applications: Radioactive isotopes, such as Cobalt-60, are used in cancer treatments through radiation therapy. This highlights the humanitarian aspect of studying such elements, as they can directly affect life quality and longevity.
- Scientific Research: Radioactive tracing aids in diverse research projects, including studies of chemical reaction rates and geological formations. They help reveal Earth's history, contributing to our understanding of natural processes.
The management of radioactive elements requires strict safety protocols due to their potential risks. Understanding these elements' behavior forms the basis for risk assessments in both medical and energy sectors. As research progresses, the applications of radioactive elements continue to expand, paving the way for new innovations and discoveries.
Applications of Chemical Elements in Technology
The field of technology relies heavily on various chemical elements. These elements serve as the backbone for numerous advancements. Their unique properties allow for a diverse range of applications, spanning from electronics to medical uses to environmental solutions. Understanding how these elements apply to technology is crucial for both practical implementations and future innovations.
Elements in Electronics
In electronics, elements such as silicon, germanium, and gallium are fundamental to the manufacturing of semiconductors. Silicon, for instance, is highly regarded for its efficiency in conducting electricity while being a poor conductor of heat. This quality makes it an ideal choice for creating integrated circuits, crucial components in computers and smartphones.
Germanium and gallium arsenide are other important elements. They often provide superior performance in various electronic devices. For example, gallium arsenide is utilized in laser diodes and solar cells, benefiting from its ability to convert solar energy to electricity more effectively than silicon. With the continuous growth in technology, the optimization of these elements remains a focal point.
Elements in Medicine
The medical field also benefits significantly from chemical elements. Notably, elements such as iron, iodine, and platinum exhibit unique properties with therapeutic applications. Iron is an essential component of hemoglobin in blood. Its relevance is central to the treatment of anemia and other blood deficiencies. Likewise, iodine is crucial in various medical imaging techniques. It is often used as a contrasting agent in X-rays and CT scans.
Platinum plays a critical role in the development of chemotherapeutic agents. By incorporating platinum into compounds, doctors can target cancer cells more effectively. The versatility of these elements reveals the profound impact that chemistry has had on medical interventions, improving health outcomes for many.
Elements in Environmental Science
Lastly, elements play an instrumental role in environmental science. For instance, carbon, in the form of carbon dioxide, is central to discussions around climate change. Understanding its behavior in the atmosphere contributes to developing strategies for emissions reduction.
Moreover, elements like lead and mercury have been studied to understand their toxic effects on ecosystems. Proper handling and awareness of these elements are crucial in preventing environmental degradation.
In terms of sustainability, elements such as lithium are vital for the production of batteries used in electric vehicles. These batteries are essential for promoting a cleaner alternative to fossil fuels. By focusing on sustainable practices involving these elements, technological advancements can contribute to protecting the environment.
"The impact of chemical elements on technology is profound, with applications that reshape our future and environment."
Safety Considerations in Handling Chemical Elements
Understanding how to safely handle chemical elements is vital for anyone working in laboratories, industrial environments, or even at home. Chemical elements can range from benign to highly toxic, and recognizing the potential hazards can prevent accidents and health risks. These considerations extend beyond merely using a pair of gloves; they encompass proper procedures for storage, disposal, and emergency responses.
Toxic Elements
Certain elements are particularly hazardous. Some examples are lead, mercury, and arsenic. These toxic elements can result in serious health issues, including poisoning and long-term neurological damage.
- Lead: This element can accumulate in the body over time, leading to cognitive impairments and developmental issues in children.
- Mercury: Exposure, even in small amounts, can cause severe health complications, including damage to the nervous system and kidneys.
- Arsenic: This element is known for its carcinogenic properties and can be present in various compounds, making it especially dangerous.
Precautions when working with toxic elements include:
- Wearing appropriate personal protective equipment (PPE), such as gloves and respirators.
- Using fume hoods to prevent inhalation of vapors.
- Ensuring proper labeling and handling to avoid accidental exposure.
Safe Storage Practices
Proper storage of chemical elements is critical to ensure ongoing safety. Factors like temperature, humidity, and containment must be considered.
Best practices include:
- Segregation: Store toxic elements separately from benign substances to avoid contamination.
- Temperature Control: Some chemicals require refrigeration or specific temperature ranges to remain stable. Make sure to read the material safety data sheet (MSDS) for guidance.
- Use of Containers: Chemicals should be stored in appropriate containers, preferably labeled with contents and hazard warnings.
In the event of an emergency, having a clearly defined protocol for dealing with spills and exposure is essential. Training and regular reviews of safety procedures can significantly reduce risks and potential accidents.
"Safety is not merely a checklist; it is a continuous commitment to ensuring that both people and chemicals are handled with care."
Future Directions in Elemental Research
The study of elemental research is poised at a fascinating frontier. As technology advances, the ability to discover and utilize elements in novel ways continues to expand. This section addresses the significance of innovations in element discovery and the sustainable utilization of these elements.
Innovations in Element Discovery
Innovations in element discovery have significant implications for various scientific fields. This includes materials science, energy production, and environmental chemistry. With the help of advanced techniques like mass spectrometry and nuclear magnetic resonance, researchers are finding new isotopes and previously unknown elements.
Recent developments have allowed for the synthesis of superheavy elements, providing insights into the forces that hold atomic nuclei together. Understanding these forces is not only fascinating but may contribute to nuclear energy applications in the future. Additionally, researchers are leveraging computational chemistry to predict properties and behaviors of these elusive elements, speeding up their discovery process.
These innovations are essential for numerous applications:
- Renewable energy technologies
- Development of advanced materials
- Enhancements in electronic devices
Furthermore, collaborations across disciplines have led to groundbreaking discoveries. By merging chemistry with fields like physics and engineering, science is unlocking potential that was once thought impossible.
Sustainable Utilization of Elements
As we uncover new elements, sustainability becomes a critical consideration. The focus on sustainable utilization of elements emphasizes a balanced approach to harnessing these materials while minimizing environmental impact. For instance, rare earth elements are crucial in creating efficient batteries and electronics. The challenge is to extract and use these elements responsibly.
A few strategies are critical for sustainable use:
- Recycling existing materials: This reduces the need for raw extraction and conserves resources.
- Developing alternatives: Research into materials that can replace rare elements is ongoing. This is vital for reducing dependency on limited resources.
- Enhancing extraction methods: Innovations in extraction technology aim to lower environmental impact during mining processes.
"In the quest for new materials and technologies, the balance between discovery and sustainability will define our chemical landscape."
Through these explorations, both students and professionals will find inspiration to engage in elemental chemistry in meaningful ways.