Fundamentals and Innovations in Electricity Generation
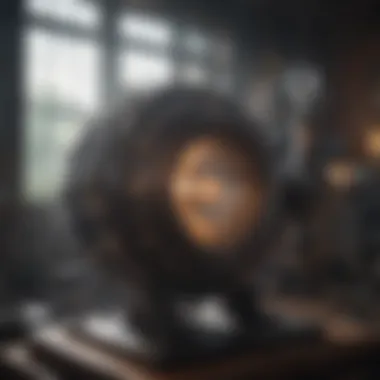
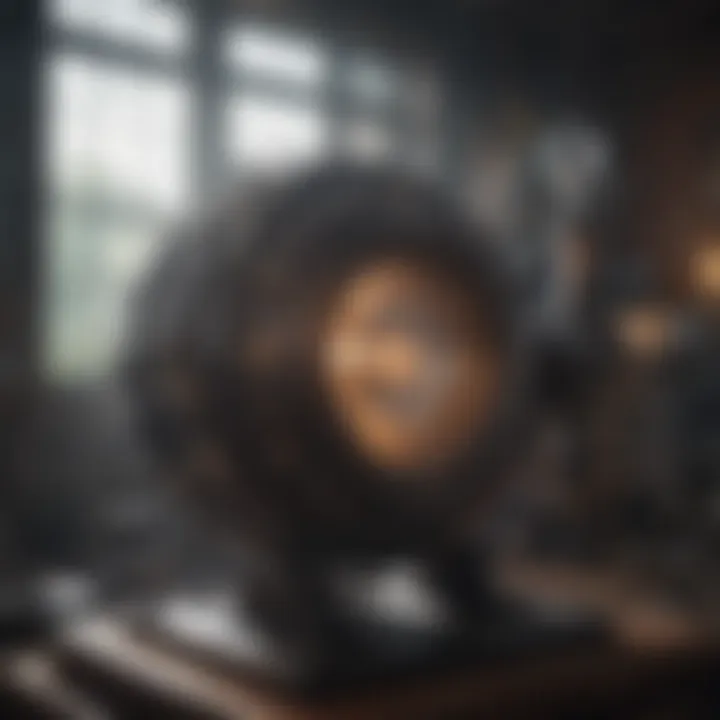
Intro
Understanding how electricity is generated is akin to peering through a window into the lifeblood of modern civilization. From powering our homes to driving industrial machinery, the nuances of electricity production weave a complex web of science, engineering, and innovation. In this exploration, we embark on a journey through the various methodologies used throughout history, the nuances of current technological advancements, and the promising future shaped by renewable energy sources.
Electricity isn't merely a commodity; it's a driving force of change. The interplay between fossil fuels, nuclear energy, and renewables like solar and wind shapes not just our grid but the entire fabric of our interconnected lives.
"Electricity generation is not just about providing power; it is about creating sustainable solutions for future generations."
For students, researchers, and professionals alike, grasping the fundamentals and innovations in this field can unveil opportunities for advancements in technology, efficiency, and sustainability. Let's dive into the core components of electricity generation, unraveling the historical and modern techniques that contribute to this essential sector.
Research Overview
Summary of Key Findings
This examination aims to shed light on several pivotal findings:
- Historical Context: Tracing the roots of electricity generation helps in understanding the evolution of various techniques.
- Current Methods: Conventional power plants and emerging technologies, including renewable energy sources, play substantial roles today.
- Environmental Concerns: Evaluating the impact of different generation methods on our ecosystem underlines the necessity of sustainable practices.
- Future Trends: Innovations in electrochemical systems hint at an evolving landscape filled with potential breakthroughs.
Background and Context
Electricity generation dates back over a century, where the steam engine laid the groundwork for future developments. Initially, coal-fired plants dominated the landscape, shaping industrial growth across continents. Over time, advancements in technology spotlighted nuclear power, which provided an alternative to fossil fuels but not without controversies about safety and waste.
In the contemporary arena, the conversation shifts towards harmonizing energy needs with sustainability. Solar panels dangling from rooftops and wind turbines sprouting in fields signify a revolution in thinking. These emerging methods strive not only for efficiency but to minimize the carbon footprint. The convergence of environmental concerns and energy needs is fostering a fresh wave of innovation, promising to redefine how we harness this crucial resource.
Understanding Electricity
Electricity is a fundamental pillar of modern life, shaping everything from our daily conveniences to groundbreaking technologies. Understanding electricity goes beyond just grasping its definition; it requires delving into its physics, generation methods, and its crucial role in our ever-evolving energy landscape. By exploring electricity's nature, characteristics, and instances in our lives, we can appreciate its significance and tackle the challenges that come with electrical consumption.
What is Electricity?
At its simplest, electricity can be defined as the flow of electric charge. This charge typically comes from subatomic particles called electrons. When these particles move through a conductive material, such as copper wire, they create an electric current that can be harnessed to power various devices. Although this definition sounds straightforward, the implications of electrical flow are profound—everything from lighting our homes to powering complex computational systems hinges on it.
The Physics of Electric Current
The physics surrounding electric current involves understanding two essential concepts: charge and flow.
Charge and Flow
Charge refers to the property of matter that causes it to experience a force when placed in an electric field. The movement of charged particles, specifically electrons, through a conductor is what we call flow. This flow of electricity is fundamental to many applications in our everyday lives today. It can efficiently transmit energy, which diverges into various forms, from light to heat, when needed.
The unique feature of this aspect lies in its capacity to create usable energy across significant distances. For example, power lines can transport electricity from a generating station hundreds of miles away to homes and industries without significant loss of energy. However, challenges like energy loss and infrastructure maintenance must be acknowledged in any comprehensive exploration of electric generation.
Voltage and Resistance
Voltage is another crucial element that measures the potential energy difference between two points in a circuit. Resistance refers to the opposition to the flow of current, and it can impact how effectively electricity is conducted through a material. Both voltage and resistance interact in ways that determine how much power flows in a circuit. Ohm's law, encapsulated by the equation V=IR (where V is voltage, I is current, and R is resistance), is a fundamental principle guiding the behavior of electrical systems.
Understanding voltage is beneficial as it helps in diagnosing electrical issues and optimizing the performance of devices. On the flip side, excessive resistance in a circuit can lead to energy loss and overheating. Balancing these factors is key in efficient electricity generation and consumption.
Types of Electricity
Electricity can mainly be classified into two types: Direct Current (DC) and Alternating Current (AC). Each type holds its unique characteristics and applications that relate directly to modern society's electricity consumption.
Direct Current
Direct Current refers to the unidirectional flow of electric charge. In this mode, power travels in one consistent path, which has its benefits in specific applications, such as batteries. This makes it a reliable choice for storing energy as it provides a stable voltage that can be easily regulated.
However, the uniqueness of DC also poses limitations. For instance, long-distance electricity transmission is less efficient compared to AC, mainly due to the inability to easily transform DC voltages to minimize losses. As such, while DC is valuable for certain technologies, like solar panels and electric vehicles, its practical use in the broader electrical grid remains limited.
Alternating Current
Alternating Current, on the other hand, involves a flow of electric charge that periodically reverses direction. AC power is favored because it can be easily transformed to higher voltages, which allows it to be transmitted over vast distances without significant energy loss. This characteristic forms the backbone of our modern electrical grid, where power plants supply homes and businesses with electricity.
The unique feature of AC enables a more versatile use across various applications, from household appliances to industrial machines. However, working with alternating current may introduce complex challenges, such as harmonics and the need for synchronization in multi-source systems. Thus, mastering AC requires deeper insight and careful consideration for ensuring overall system efficiency.
"Electricity is not a commodity; it’s a current, always constant yet ever changing."
Historical Context
Understanding the historical context of electricity generation provides valuable insights into how this crucial technology evolved and shaped our modern world. The journey from early discoveries to contemporary methods reveals not only technical advancements but also the societal transformations they catalyzed. Recognizing these developments allows us to appreciate the complexities involved in harnessing electricity and contemplating future innovations.
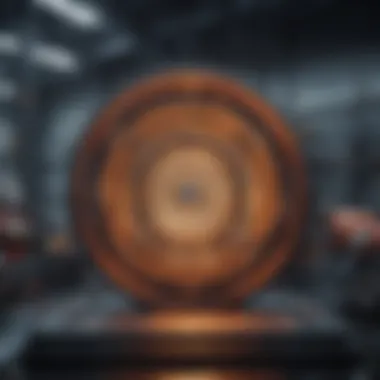
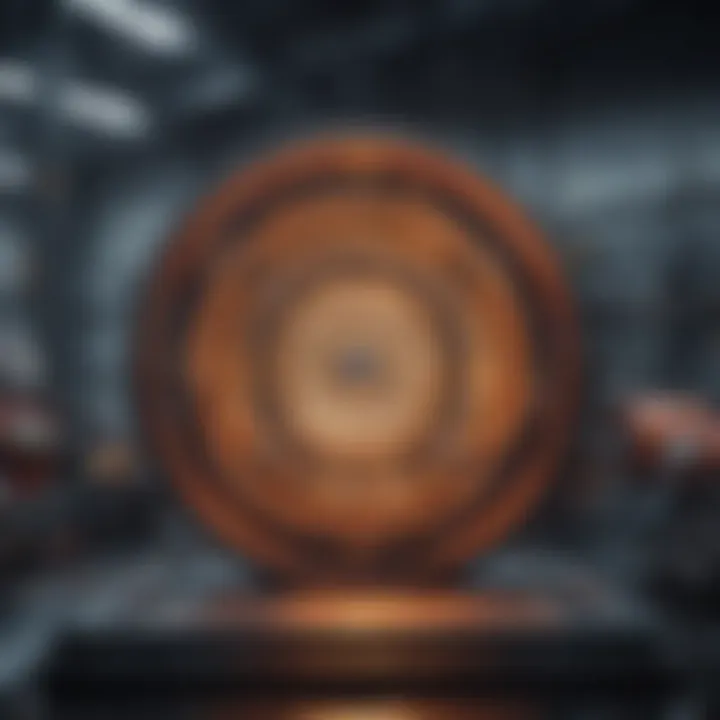
Early Discoveries
Static Electricity
Static electricity, often characterized by the buildup of electric charge on surfaces, marked a significant preliminary step in the field of electricity. One key characteristic of static electricity is that it can be easily observed; for instance, rubbing a balloon against your hair allows one to feel a distinct attraction between the two. This phenomenon is crucial because it highlights the fundamental principles of charge interaction, a concept that is foundational to understanding electricity in broader contexts.
A unique feature of static electricity is its visibility -- it’s something almost anyone can demonstrate. This easy demonstration contributes greatly to its initial appeal, making it a cornerstone in the educational foundations of electricity. In the context of this article, static electricity's relevance lies in its role as a springboard for further investigations and discoveries. However, static electricity has limitations. Being primarily cumulative and transient, it does not provide a consistent power source for any practical application.
Galvanism
Galvanism, the phenomenon of electricity produced by chemical reactions, was a game-changer for early electricity studies. Named after Luigi Galvani, who noted that frog legs twitched in response to electrical stimuli, galvanism opened doors to understanding bioelectricity and electrochemistry. A notable aspect of galvanism is its connection to living organisms, instilling intrigue among scientists and sparking further research into the relationship between electricity and life.
This idea of utilizing chemical reactions to produce electricity is a critical stepping stone towards today's battery technology. While galvanism's unique feature lies in its application for a wide range of chemical reactions, it presents disadvantages as well. The efficiency of energy conversion in early galvanic cells was relatively low, posing challenges for commercialization. Nevertheless, galvanism laid the groundwork for future innovations in the field of electrochemistry.
The Age of Invention
Faraday and Electromagnetic Induction
Michael Faraday's work on electromagnetic induction marked a pivotal moment in the history of electricity generation. His discovery that a change in magnetic fields can induce an electric current is a foundational principle that is still employed in generators today. Faraday’s key characteristic, his innovative experimental methods, distinguished him from his contemporaries, bringing hands-on science to the forefront.
Moreover, Faraday’s experiments demonstrated that electricity could be generated without the need for chemical reactions, revealing a broader spectrum of possibilities for energy generation. One significant advantage of Faraday's approach is its efficiency and applicability; it led directly to practical technologies, such as the dynamo. Conversely, a potential disadvantage is that Faraday's methods were largely empirical and often lacked theoretical grounding at the time, which slowed further progress until his principles were better understood.
Tesla's Contributions
Nikola Tesla’s contributions to electricity generation cannot be overstated. Tesla's pioneering work with alternating current (AC) systems revolutionized the way electricity could be transmitted over long distances. His key characteristics included a profound understanding of electromagnetic fields and innovative applications of those insights to create functional devices, such as AC motors.
Tesla’s unique feature lies in his vision for a more interconnected power grid, which could enable efficient delivery of electricity across vast areas. This forward-thinking perspective made him a remarkable figure in this era of innovation. However, the widespread adoption of Tesla’s AC system faced challenges, primarily due to the strong influence of other technology proponents, such as Thomas Edison, who was a staunch advocate of direct current (DC).
The Electrification Era
Power Grids
The establishment of power grids signaled a transformative phase in electricity generation, allowing for a centralized approach to energy distribution. Power grids consolidate various sources of electricity and deliver them to consumers efficiently. A key characteristic of these grids is their ability to balance supply and demand dynamically, which has become increasingly essential as energy sources diversify.
The unique feature of modern power grids is the integration of smart technology, enabling real-time monitoring and control. This modern setup presents significant advantages, such as improved reliability and efficiency in energy delivery. However, the complexity of maintaining these grids can also lead to vulnerabilities, such as susceptibility to cyber-attacks and infrastructure failures.
Industrial Applications
Industrial applications played a significant role in the evolution of electricity generation. The ability to harness electric power for manufacturing processes led to a greater scale of production and efficiency. A notable feature of industrial applications is their scale; industries such as steel production and chemical manufacturing rely heavily on electricity to power machinery and control processes.
The advantages of utilizing electricity in industrial settings include increased productivity and lower operational costs. However, the reliance on electricity also raises challenges, particularly concerning the sustainability of energy sources. Industries must balance their energy needs with environmental considerations to ensure sustainable operation. In sum, the historical context of electricity generation illustrates not just a timeline of discoveries and advancements, but also the interwoven complexities that have brought us to the present day.
Current Generation Methods
Electricity generation is a cornerstone of modern civilization. Current generation methods form the backbone of how we harness energy to power our homes, industries, and essential services. Understanding the various techniques used today is vital in addressing energy demands and environmental challenges. The intricacies of these methods reveal how society balances efficiency, sustainability, and innovation while tackling the ever-present quest for reliable power.
Thermal Power Generation
Thermal power generation, derived from the heat energy produced by burning fossil fuels or through nuclear reactions, remains a predominant method globally. The process involves creating steam that drives turbines connected to generators.
Fossil Fuels
When it comes to fossil fuels, oil, coal, and natural gas take the spotlight for their historical role in energy generation. The main draw of fossil fuels is their capacity to produce significant amounts of energy from relatively small quantities of fuel. For instance, a ton of coal can generate enough electricity to power a standard home for months. This efficacy makes fossil fuels a powerful choice, especially for developing regions looking to establish robust power infrastructures.
However, a critical issue lurks beneath the benefits. The combustion of fossil fuels releases greenhouse gases, significantly contributing to climate change. With a looming urgency to transition to cleaner energy sources, many argue that this dependence on fossil fuels must be reconsidered. As a result, while fossil fuels currently dominate the landscape, their future is fraught with uncertainty due to increasing regulatory and social pressures to reduce carbon footprints.
Nuclear Power
On the other hand, nuclear power offers an alternative that avoids the direct pollution associated with fossil fuels. It generates electricity through the process of nuclear fission. This method yields an enormous amount of energy from a relatively small amount of fuel, such as uranium. It’s not uncommon for just one uranium pellet to produce enough energy to power a home for days.
Yet, the allure of nuclear power comes bundled with its own set of challenges. The long-term effects of radioactive waste disposal raise significant concerns. Incidents like Chernobyl and Fukushima serve as stark reminders of the potential dangers involved. Despite this, nuclear energy remains a cornerstone of the energy mix in several countries, often touted as a necessary transition method toward more sustainable energy practices.
Hydroelectric Power
Hydroelectric power harnesses the kinetic energy of flowing water, converting it into electricity. This renewable source leverages the natural water cycle and is one of the oldest and most trusted methods for generating power.
Principles of Hydraulics
At the heart of hydroelectric power are the principles of hydraulics. Essentially, this entails the movement of water through canals or pipes, which can spin turbines that generate electricity. The efficiency of this method is impressive, as it often surpasses 90%, highlighting its potential for energy generation without the emissions associated with fossil fuels.
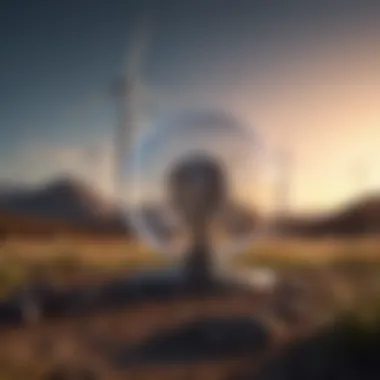
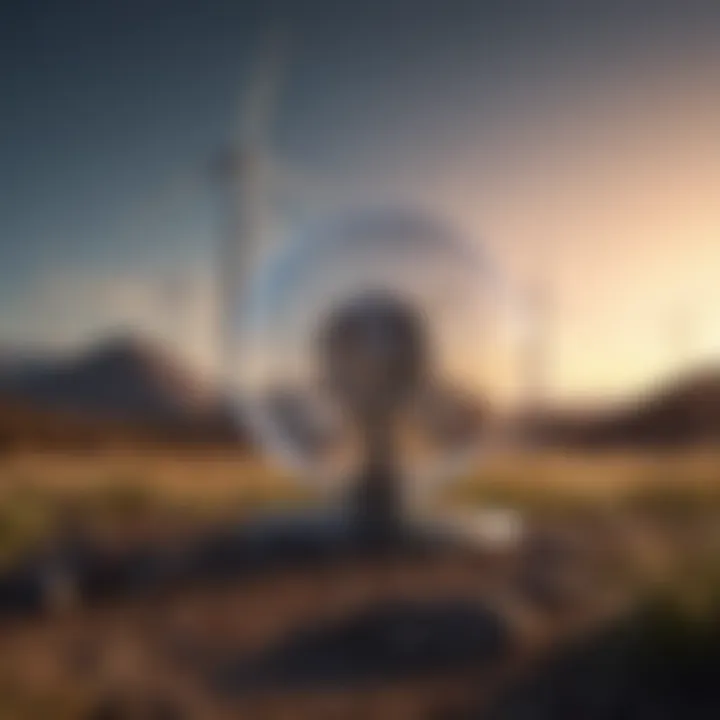
The primary characteristic of hydraulic systems is their reliance on gravity and water pressure, allowing them to work continuously as long as there is a supply of flowing water. However, significant environmental impacts arise, such as alterations to local ecosystems and the displacement of communities for dam construction, which cannot be overlooked.
Dam Design and Function
Dams play a pivotal role in harnessing hydroelectric power by impounding rivers and creating a reservoir. Key to this design is their ability to regulate water flow, ensuring a consistent power supply, especially during dry seasons.
Construction of large-scale dams can be a double-edged sword. While they generate large amounts of renewable energy and provide water management benefits, they often disrupt aquatic habitats and can lead to significant social ramifications for affected local populations. The ongoing debate regarding dam impacts versus benefits illustrates the complexities of relying exclusively on hydroelectric power.
Renewable Sources
In the quest for sustainable energy solutions, renewable sources have emerged as viable alternatives to both fossil and nuclear power. These methods focus on harnessing natural phenomena to generate energy.
Solar Power
Solar power is perhaps the most visible form of renewable energy available today. It employs photovoltaic cells to convert sunlight directly into electricity. This technology is incredibly appealing due to its scalability, ranging from small residential systems to massive solar farms that contribute extensively to national grids. The sun's inexhaustible nature gives solar power a significant advantage, as there are no fuel costs and minimal environmental emissions.
However, solar energy isn't without its challenges. The intermittent nature of sunlight can lead to supply inconsistencies, requiring battery systems or alternative generation methods to ensure energy availability during night or cloudy days. Recent advancements in energy storage technology are continually improving the viability of solar power as a primary energy source.
Wind Energy
Wind energy capitalizes on the natural power of moving air. Wind turbines convert kinetic energy into electricity, proving to be one of the fastest-growing energy sources globally. Wind farms can be constructed onshore or offshore, providing tremendous land-use flexibility.
The versatility of wind energy makes it a popular choice for many regions, particularly where consistent wind patterns prevail. Although wind energy production is clean and prevents harmful emissions, concerns about noise pollution and effects on local wildlife persist, particularly with bird and bat mortality due to turbine strikes.
Geothermal Resources
Geothermal energy taps into the Earth's internal heat, using steam or hot water from below the surface to generate power. This method is predominantly location-specific, necessitating drilling and geological assessments to identify suitable areas.
What sets geothermal resources apart is their capability to provide a continuous power supply, unaffected by atmospheric conditions like sun or wind. Despite this, the high upfront costs and geographic limitations impede broader implementation, leading to its status as a supplemental rather than a primary energy source in most regions.
Establishing clarity on current generation methods is essential in navigating the future landscape of electricity production. Each technology has its limitations and advantages, and understanding these nuances enables informed decisions in energy policy and personal energy use.
Alternative Energy Technologies
The incorporation of alternative energy technologies marks a significant transition in the landscape of electricity generation. These innovations not only enhance the sustainability of energy production but also tackle pressing environmental issues associated with traditional fossil fuel usage. From reducing greenhouse gas emissions to enhancing energy efficiency, alternative energy technologies are crucial in curbing climate change and fostering a greener world. They serve as a bridge from the antiquated systems of the past to a more sustainable and innovative future.
Emerging Technologies in Energy Capture
Photovoltaic Innovations
Photovoltaic innovations are transforming the way solar energy is harnessed. The key aspect here is the efficiency ratio - how effectively sunlight is converted to usable electricity. New materials, like perovskite solar cells, push efficiency levels of solar panels beyond the normal thresholds. This makes photovoltaics not only more efficient but also potentially cheaper to produce.
The unique feature of these innovations is their versatility in application. From residential rooftops to vast solar farms, photovoltaic systems are adaptable to various environments. Fleet-footed deployment of solar technology offers a swift pathway to mitigate energy crises in an eco-friendly manner. However, one must not gloss over the durability and longevity of these panels; they often require replacement after 25-30 years, which could incur extra costs.
Advanced Wind Turbines
Advanced wind turbines represent another leap in alternative energy technologies. These systems are designed to capture wind energy with greater efficiency and at lower wind speeds. The remarkable characteristic of modern turbines lies in their aerodynamics, which has radically improved turbine blades to optimize energy production.
Moreover, their larger capacity means they generate more power compared to older models. This translates into less land requirement for the same output, which is a key selling point—especially in densely populated or environmentally sensitive areas. Still, it is important to consider some drawbacks, such as potential impacts on wildlife and the intermittent nature of wind energy, which necessitate complementary systems for unwavering power supply.
Energy Storage Solutions
Energy storage solutions are indispensable for tackling the inherent instability of renewable sources. These technologies ensure that captured energy can be stored and utilized whenever demand is high, thus supporting grid stability and energy reliability.
Batteries and Capacitors
Batteries and capacitors play a central role in energy storage. Batteries tend to offer a higher energy density, which makes them suitable for longer-term storage applications. Lithium-ion batteries, for example, are commonly used in electric vehicles and grid storage due to their efficiency and reliability.
On the flip side, capacitors serve well for short bursts of energy. What stands out is their capacity to charge and discharge rapidly, making them ideal for applications like stabilizing voltage fluctuations. However, this comes with a trade-off; their total energy capacity is comparatively lower than batteries, thus limiting their role in long-term energy supply.
Supercapacitors
Supercapacitors, known for their rapid charge and discharge capabilities, have become essential in many applications parallel to batteries. They are particularly valued for their longevity, often lasting over a million charge cycles. This makes them a cost-effective solution in the long run.
Their unique feature is the ability to harvest energy from renewable sources quickly, which can be particularly beneficial during peak load times. Yet, the downside lies in their energy density - supercapacitors can't hold a substantial amount of energy compared to batteries, hence they are often employed in conjunction with battery systems to create a balanced and efficient energy storage solution.
"Embracing alternative energy technologies is not just about producing power, it's about shaping a sustainable future and safeguarding our planet for generations to come."
Environmental Considerations
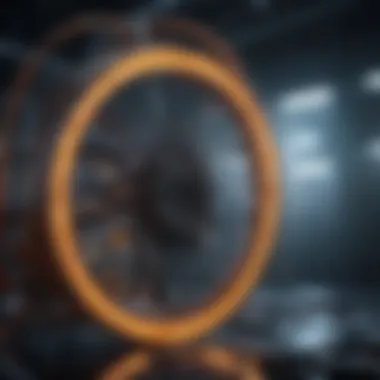
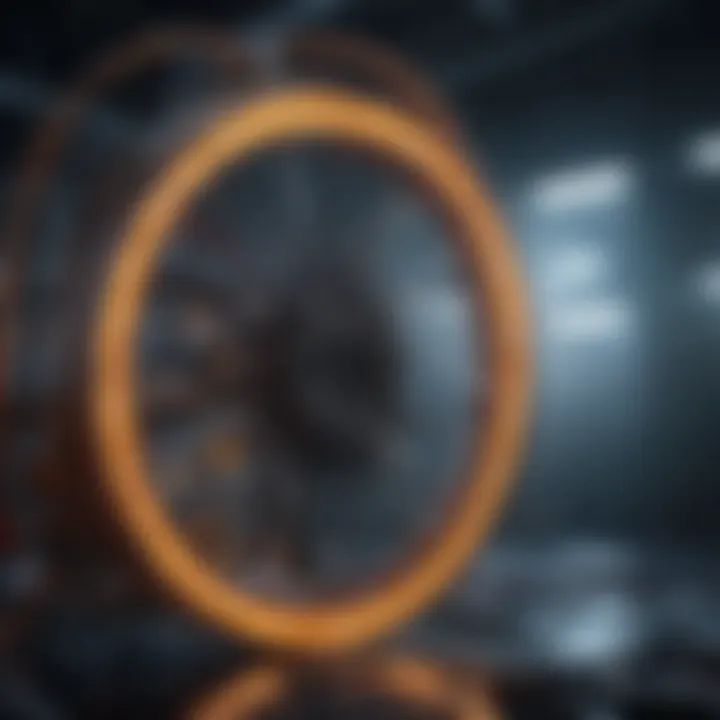
The conversation surrounding electricity generation today cannot sidestep the pressing topic of environmental considerations. These factors are not just an afterthought; they play a critical role in shaping how technologies are developed and implemented in real-world scenarios. As humanity grapples with climate change and the consequent policy shifts, understanding the environmental impacts becomes imperative for students, researchers, educators, and professionals. Here, we delve into the complexities of electricity generation and dissect what it means for the environment.
The Carbon Footprint of Electricity Generation
Impact of Fossil Fuels
Fossil fuels have served as the backbone for energy generation for decades. Their potency is derived from their high energy density; however, that's just scratching the surface. When we examine the impact of fossil fuels on the environment, the glaring characteristic that stands out is their significant carbon emissions, which are a leading contributor to global warming.
Why are fossil fuels a popular choice? The infrastructure for harnessing these energy sources is already in place, making them convenient and economically viable in many regions. Natural gas, coal, and oil power plants are capable of producing energy at scale, supporting industries and residential needs alike. Yet, the distinctive feature comes with a caveat – the very processes that generate power also spew out greenhouse gases.
The advantages of fossil fuels include not only their ability to generate massive electricity ratios but also their role in job creation and supporting economies reliant on these energy sources. However, the disadvantages are becoming increasingly hard to ignore, with studies revealing their long-term costs associated with health, environmental degradation, and climate change.
Lifecycle Analysis of Renewable Sources
In contrast, the lifecycle analysis of renewable sources presents a different narrative. This approach assesses the environmental impact of energy sources from cradle to grave. It looks at various stages, including material extraction, manufacturing, usage, and eventual disposal or recycling. It’s an essential criteria for understanding how each energy source contributes to or mitigates ecological harm.
One key characteristic of lifecycle analysis is its ability to quantify emissions over the entire life of a resource. This method highlights that while certain renewables, like solar panels, may have a higher initial environmental cost during production, they often yield significantly lower emissions when in operation. The compelling aspect here is that renewable energy technologies do not emit greenhouse gases during their operational phase, which makes them candidates for a more sustainable energy future.
The unique attribute of lifecycle analysis is that it spells out both pros and cons. While renewables promise sustainability and lower emissions, their production can also sometimes be resource-intensive, leading to concerns over ecological footprints in different contexts. This nuanced perspective often serves as a call to innovate rather than merely replace one energy source with another.
Sustainable Practices
Energy Efficiency
Energy efficiency stands at the forefront of sustainable practices in electricity generation. This concept aims to provide the same service while consuming less energy, optimizing resources in the process. The key to energy efficiency is that it is not merely about reducing consumption but also improving technological standards.
This practice remains a preferential choice in discussions about sustainable energy because it offers quick wins for reducing overall energy consumption. For instance, improving insulation in buildings can drastically lower heating costs and energy expenditures, all without compromising comfort. Unique to energy efficiency is that it can often be implemented in existing infrastructures, offering immediate benefits without the need for complete overhauls.
However, there's also a flip side. Transitioning toward a more efficient energy landscape can require significant upfront investment or policy shifts. If not managed correctly, it can lead to a misleading perception that less energy usage is equivalent to reduced impact, while the reality is that efficient systems still require careful monitoring and ongoing innovation.
Eco-friendly Innovations
When discussing sustainable practices, eco-friendly innovations take the cake. These are progressive solutions born from a desire to reduce environmental harm while enhancing energy production. Innovations like biogas generation, advanced geothermal systems, and ocean current energy capture are pioneering pathways toward greener energy landscapes.
The leading edge of eco-friendly innovations is that they often employ new technologies that are less harmful to the environment in varying capacities – from production to end-of-life disposal. This approach isn't just about generating energy; it's about how this energy moves through the entire ecosystem.
A notable aspect of eco-friendly innovations is their potential to revolutionize energy access, particularly in remote or underserved areas. While they promise significant advancements, there are also disadvantages. They can involve high initial costs, complexities in operation, and the need for skilled labor. Hence, balancing innovation with practicality is paramount for wider adoption.
"Innovation in energy is a dance of balancing carbon reduction with accessibility."
Ultimately, as we proceed into the future of electricity generation, paying heed to environmental considerations will be pivotal, guiding choices that respect ecological systems while still meeting societal demands.
Future of Electricity Generation
The future of electricity generation holds a crucial place within the broader context of our global energy landscape. Modernization in energy systems is no longer just an option; it's a necessity. As we thread through continuously evolving technologies and reliance on innovation, it’s important to not only consider the environmental impacts but also how society can adapt to new systems. The drive toward a sustainable energy future demands a nuanced understanding of consumption patterns and emerging technologies.
Trends in Energy Consumption
Smart Grids
Smart grids are turning what once was a one-way street of information into a highway filled with data, communication, and interaction. These grids represent a significant move away from the traditional electrical grid. Their key characteristic lies in their ability to communicate instantaneously between power providers and consumers, leading to improved efficiency.
One unique feature of smart grids is their capacity to integrate renewable energy sources seamlessly while managing their variability. The advantages of smart grids include enhanced reliability, lower costs over time through better resource allocation, and an encouraging environment for a range of renewable technologies. However, the introduction of smart grids does come with challenges; cybersecurity is a principal concern as the interconnectivity exposes power systems to potential threats.
Decentralized Energy Systems
Decentralized energy systems embody a shift in energy generation that empowers local communities. Instead of relying solely on large power plants, these systems generate energy distributedly, usually at the point of consumption. A key characteristic of these systems is their ability to adapt to local energy needs, often utilizing renewable sources such as solar panels or small wind turbines.
What makes decentralized systems particualry appealing is their potential to minimize transmission losses. Localized energy generation can also enhance energy security, making communities less reliant on centralized grids that may be vulnerable to outages or disruptions. Still, there are challenges to overcome. The complexities of integrating these systems into existing infrastructures can pose operational hurdles, and the initial setup costs can deter widespread adoption in some areas.
Anticipated Technological Advances
Artificial Intelligence in Energy Management
Artificial intelligence (AI) is rapidly deploying its potential into energy management, optimizing the use of resources like never before. The core aspect of AI's involvement revolves around data analytics, which allows for smarter predictions regarding energy consumption patterns. With its ability to analyze vast amounts of information, AI gives energy providers the tools needed to enhance efficiency across their operations.
A significant benefit of employing AI is its capability to predict energy demand with remarkable accuracy. This assists in optimizing supply chains and ensuring a balanced load, effectively reducing instances of blackout or energy waste. Nevertheless, challenges exist in terms of regulatory frameworks and the need for skilled personnel familiar with advanced technologies.
Fusion Energy Research
Fusion energy research stands at the frontier of our energy conversation, resembling an elusive goal as it mirrors the processes that power the sun. This energy source promises a low-carbon option with the potential to generate enormous amounts of electricity. A key characteristic of fusion energy is its sustainability, as its fuel—primarily isotopes of hydrogen—is vastly available and extremely dense when it comes to energy content.
The unique feature that sets fusion apart is its safety; in contrast to nuclear fission, fusion poses no risk of catastrophic failures. While the advantages are clear, the hurdles to practical fusion energy lie in the technological complexities and costs associated with achieving the necessary conditions for fusion reactions. Opting for fusion energy could provide an almost limitless energy source, but it will require continued investment and research to turn these theoretical experiments into practical reality.
"The future of electricity generation is about blending technology with sustainable practices to build a more resilient and efficient power grid that meets the demands of modern life."
In summarizing the dynamic landscape of electricity generation, understanding these trends and technologies can help guide sustainable practices for future advancements in the energy sector.