Guide RNA and CRISPR: A Comprehensive Exploration
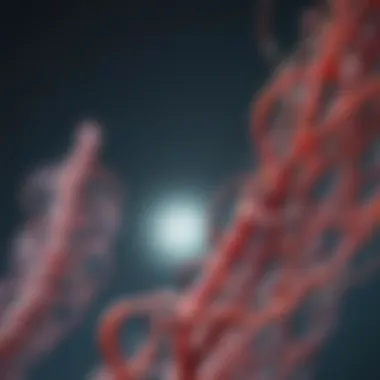
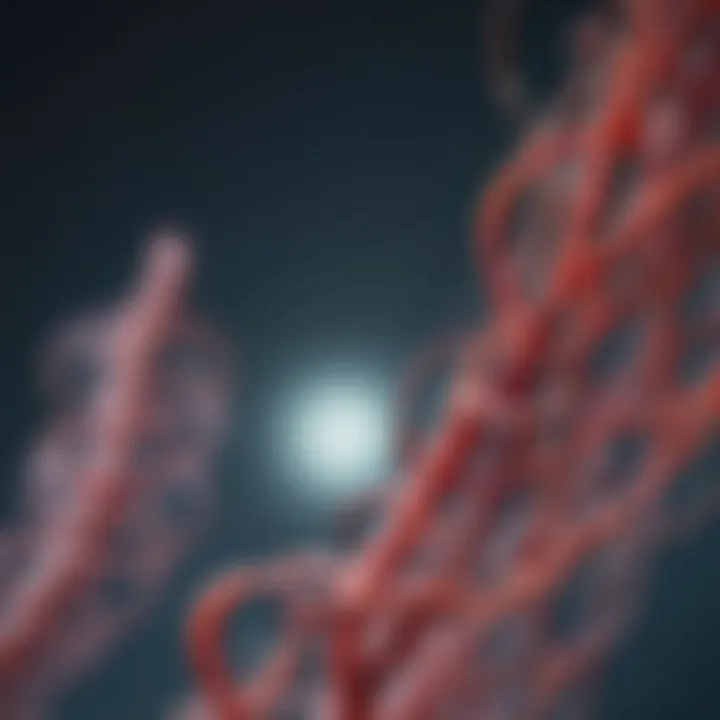
Intro
The emergence of CRISPR technology has drastically shifted the landscape of genetic research and modification. Within this realm, guide RNA serves a pivotal purpose. Its role in the CRISPR-Cas9 system is not just fundamental but transformative. The ability of guide RNA to direct a molecular ‘scissors’ to precise locations on the genome has revolutionized fields such as medicine and agriculture.
In this detailed exploration, we delve into several critical aspects of guide RNA's functionality. We will look at how it is designed, its biochemical properties, and its applications. Moreover, we will touch on ethical implications associated with its use, the current limitations of CRISPR, and prospective future developments. This multifaceted approach aims to enhance understanding among students, researchers, educators, and professionals who seek a deeper insight into this cutting-edge technology.
Research Overview
Summary of Key Findings
Guide RNA is integral to the efficacy of the CRISPR-Cas9 genome editing process. It is the component that ensures accuracy and specificity in targeting specific DNA sequences. Key findings indicate that:
- The structure and design of guide RNA directly influence editing efficiency.
- Variations in guide RNA can lead to different outcomes in genetic modifications.
- Understanding the interactions between guide RNA and the target DNA is essential for improving CRISPR techniques.
Background and Context
The scientific community recognized the CRISPR system's potential in the early 2010s. Initially an adaptive immune response mechanism in bacteria, CRISPR has become a powerful tool for genome editing.
Guide RNA is composed of two main parts: the scaffold RNA and the targeting RNA. The scaffold stabilizes the Cas9 enzyme, while the targeting RNA specifies the DNA sequence for modification. This system allows researchers to create targeted genetic changes with remarkable precision.
Such advances prompt significant implications within various sectors, including therapeutic development and crop engineering. Nonetheless, the quick development of this technology brings about various ethical considerations, positioning researchers to navigate these complexities carefully.
“Guide RNA enables a level of precision in genetic editing that was previously unattainable.”
The practical applications of CRISPR technology underscore its revolutionary nature, but they also necessitate a deeper understanding of its components, particularly guide RNA.
Prologue to CRISPR Technology
CRISPR technology has transformed the field of genetics and molecular biology. This revolutionary tool allows for precise editing of DNA, enabling researchers to modify genes with unprecedented accuracy. The importance of this technology cannot be overstated; it opens new avenues in medicine, agriculture, and biotechnology. Understanding CRISPR involves delving into its history and mechanisms, which lay the groundwork for its applications today.
History of CRISPR Discovery
The discovery of CRISPR sequences began in the late 1980s. Researchers first identified repetitive DNA sequences in the genomes of bacteria. These sequences were initially of little interest; however, over time, their significance became clear.
In 2005, a breakthrough occurred when scientists discovered that these CRISPR sequences acted as a form of immune defense in bacteria. They provided a way for bacteria to recognize and cut foreign genetic material, such as that from viruses. This foundational knowledge paved the way for the development of CRISPR as a gene-editing tool. In 2012, Jennifer Doudna and Emmanuelle Charpentier created the CRISPR-Cas9 system. This advancement provided a straightforward means to edit genes across various organisms, from bacteria to humans.
Mechanism of CRISPR-Cas9
The CRISPR-Cas9 mechanism is striking in its simplicity and efficiency. At its core, this system involves two main components: the guide RNA and the Cas9 protein. The guide RNA functions as a navigation tool, directing the Cas9 protein to the target DNA sequence.
- Guide RNA Design: The guide RNA is designed to match the specific sequence of DNA intended for editing. This specificity is essential to ensure that only the desired target is affected.
- Targeting Process: Once the guide RNA aligns with its complementary DNA sequence, the Cas9 protein binds to the RNA-DNA complex. The Cas9 then makes a precise cut in the DNA at the specified location.
- DNA Repair Mechanisms: After the cut, the cell’s natural repair mechanisms are activated and can be harnessed to introduce desired changes, such as inserting or deleting genetic material.
This dual-functionality not only contributes to the effectiveness of CRISPR-Cas9 but also marks a significant advancement over earlier gene-editing techniques. The ability to edit genomes with this precision has profound implications for research and application in diverse fields.
Understanding Guide RNA
The topic of guide RNA serves as a cornerstone in the CRISPR-Cas9 genome editing system. Understanding its role provides clarity on how CRISPR technology functions effectively. Guide RNA is critical as it determines the accuracy of the CRISPR-Cas9 mechanism, directing the Cas9 protein to specific locations in the genome. This specificity is vital for successful gene editing, minimizing unintended alterations.
Additionally, exploring guide RNA contributes to knowledge about optimizing CRISPR applications across various disciplines, from agriculture to medical research.
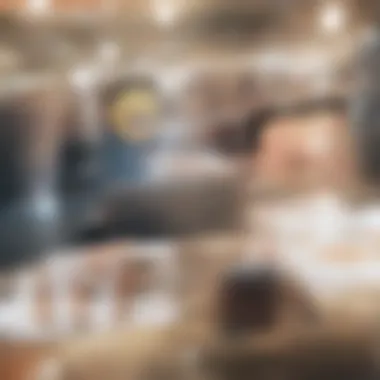
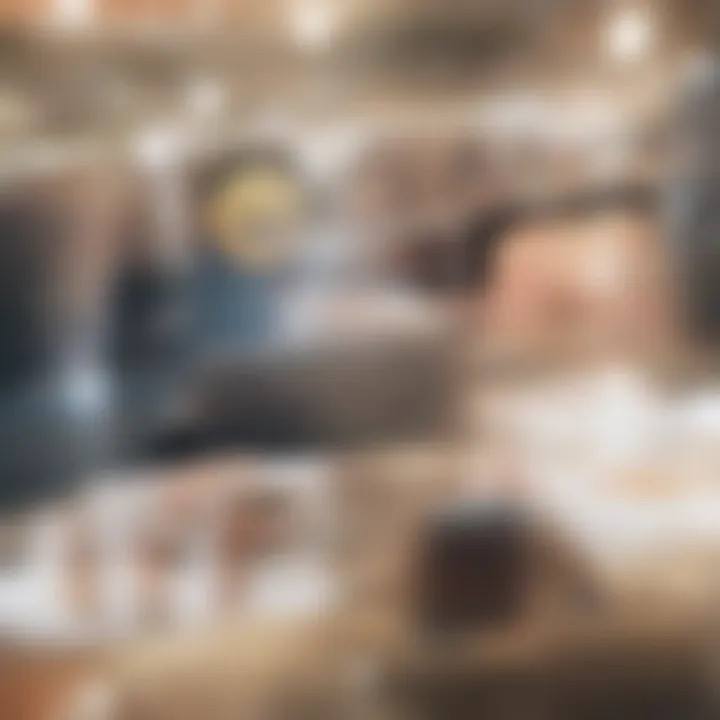
Composition of Guide RNA
Guide RNA (gRNA) is made up of two critical components: the scaffold sequence and the targeting sequence. The scaffold sequence is essential for the binding of the Cas9 protein. It ensures the proper structure and stability of the gRNA-Cas9 complex. The targeting sequence is uniquely designed to complement a specific target DNA sequence. The length of the targeting sequence usually ranges from 20 to 22 nucleotides, which provides sufficient specificity.
A well-designed gRNA can significantly enhance the efficacy of gene editing. This bodes well for applications in genetic engineering, where precision is paramount.
Types of Guide RNA
Different types of guide RNA have emerged to meet various needs in CRISPR applications. Key classifications include single-guide RNA and dual-guide RNA.
Single-guide RNA
Single-guide RNA is the most widely used form in CRISPR applications. It combines both the targeting and scaffold sequences into a single strand. This simplicity allows for easy design and synthesis. Notably, single-guide RNA provides a streamlined approach, reducing the complexity involved in creating multiple RNA strands. The advantage is clear: designing a single-guide RNA is often more efficient and cost-effective. However, one consideration is that while it performs well for most applications, there may be cases where it lacks versatility when targeting complex genomic regions.
Dual-guide RNA
Dual-guide RNA takes a different approach by using two separate RNA molecules. One molecule serves as the scaffold, while the other encompasses the targeting sequence. This arrangement allows for enhanced binding to the Cas9 protein, improving precision in certain contexts. A unique feature of dual-guide RNA is its potential to target two sites simultaneously. This can be beneficial in applications requiring multiple edits at once. Nevertheless, the complexity of designing dual-guide RNA might pose a challenge compared to single-guide RNA.
As CRISPR technology evolves, understanding these types of guide RNA is essential for selecting the appropriate method for specific applications.
Guide RNA Design Considerations
The design of guide RNA plays a significant role in the success of CRISPR-Cas9 genome editing. Guide RNA, short for gRNA, acts as a crucial component in the CRISPR mechanism, ensuring that the Cas9 enzyme reaches and cleaves the correct DNA sequence. Proper design considerations can enhance the efficiency and specificity of the editing process while minimizing unintended consequences. Understanding these considerations is vital for researchers and professionals involved in genetic modifications.
Target Sequence Selection
Choosing the correct target sequence is foundational to guide RNA design. This sequence should be unique to the region of interest in the genome. It is essential that it matches the target DNA precisely because even minor mismatches can compromise the gRNA’s ability to guide Cas9 accurately. For effectiveness, the selected sequence often extends around 20 nucleotides prior to a protospacer adjacent motif (PAM) site, which allows the Cas9 to recognize where to bind and cut.
When selecting sequences, one should utilize bioinformatics tools to analyze the genome. These tools can identify potential off-target sites, which are unintended sequences that may be affected by the gRNA. Database resources such as the UCSC Genome Browser and Ensembl can facilitate these analyses.
Efficiency and Specificity
The efficiency of guide RNA is determined by how effectively it can recruit Cas9 to the target DNA. Factors influencing efficiency include the secondary structure of the gRNA and its binding affinity for target DNA. A well-designed gRNA should have a stable structure and minimal potential for off-target binding.
Specificity, on the other hand, is critical for ensuring that CRISPR technology is precise. Researchers aim for a gRNA that binds only to the intended target without affecting similar sequences elsewhere in the genome. Enhancing specificity can be achieved by selecting unique sequences and optimizing gRNA design parameters. For example, using a truncated gRNA can increase binding efficiency while reducing off-target effects.
Potential Off-Target Effects
Off-target effects represent one of the significant challenges in CRISPR technology. These occur when the gRNA binds to unintended genomic locations, potentially resulting in unanticipated mutations. Addressing target specificity in gRNA design is imperative to mitigate these risks.
Researchers often perform a thorough analysis of potential off-target sites prior to experiments. Techniques such as whole-genome sequencing can detect these effects post-editing, allowing for assessment of unintended variations. Optimizing gRNA sequences to minimize homology with non-target sites can greatly reduce the chance of off-target cleaving.
It is vital to balance efficiency and specificity for successful genome editing outcomes.
Applications of Guide RNA in CRISPR
The role of guide RNA in controlling the CRISPR-Cas9 system cannot be overstated. Its design and application have far-reaching implications in various domains, such as genetics, agriculture, and medicine. This section will delve into three key areas of application: gene editing in animal models, application in plants, and therapeutic potential in humans. Understanding these applications sheds light on the transformative capacity of guide RNA within CRISPR frameworks.
Gene Editing in Animal Models
Gene editing in animal models serves as a crucial step in validating therapeutic strategies, studying genetic diseases, and understanding biological processes. The precision offered by guide RNA allows scientists to target specific genes in organisms such as mice or rats, mimicking human genetic conditions. This approach enables a deeper exploration into gene function and interactions.
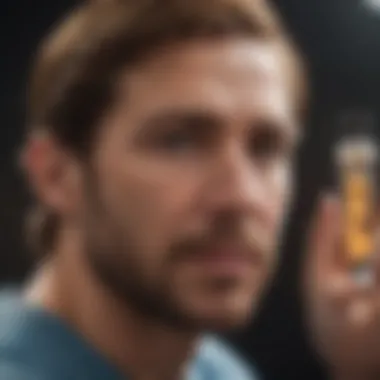
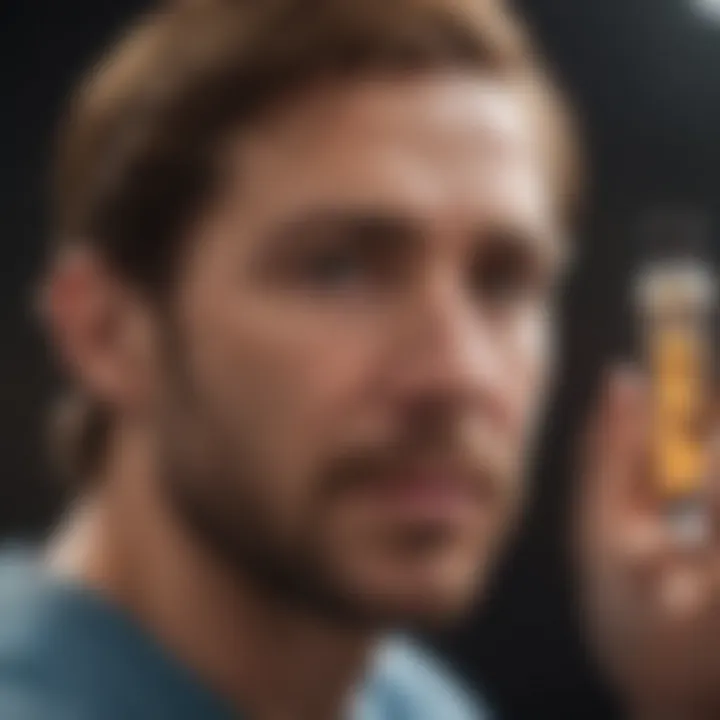
Before using CRISPR technology, researchers often screen potential target sites within the genome to select appropriate guide RNA sequences. Efficiency of gene modifications can vary, but recent advancements have significantly improved success rates. Additionally, animal models developed through guide RNA-mediated edits can be used for drug testing, leading to more effective treatments for diseases.
Application in Plants
The application of guide RNA in plants has revolutionized agricultural practices. By introducing specific genetic changes, researchers can enhance crop resistance to diseases, pests, and environmental stresses. This is particularly important given the increasing global demand for food coupled with the challenges posed by climate change.
The CRISPR-Cas9 system enables the development of genetically modified crops that retain favorable traits while minimizing undesirable characteristics. This can lead to plants with improved yield and nutritional quality. One effective use of guide RNA has been to engineer plant varieties that can withstand drought or higher salinity. This method is advantageous, as it can provide solutions to food security in challenging conditions.
Therapeutic Potential in Humans
In the realm of human health, the therapeutic potential of CRISPR technology, guided by precise RNA sequences, is immense. Guide RNA's capability to target specific genes paves the way for treatments of genetic disorders. For instance, conditions such as sickle cell disease and certain forms of muscular dystrophy may be addressed through gene editing, correcting mutations at their source.
However, this potential is coupled with ethical considerations, particularly concerning germline editing. Ethical frameworks are being developed to address concerns about unintended consequences and the long-term impacts of gene editing on future generations. Nevertheless, ongoing research continues to provide hope that conditions once deemed incurable could potentially be treated through CRISPR-based therapies.
"Guide RNA, as a crucial element in the CRISPR-Cas9 toolkit, enables precise editing, marking a significant shift in genetic engineering approaches."
Ethical Considerations in CRISPR Research
As research in CRISPR technology advances, ethical considerations have become ever more critical. The potential applications of CRISPR-Cas9, especially concerning gene editing, raise questions about the long-term implications for society and the environment. Addressing these ethical issues is crucial for responsible research and application. This section will focus on germline editing concerns and the regulatory frameworks that can help navigate these challenges.
Germline Editing Concerns
Germline editing refers to changes made to the DNA of germ cells, which can be passed onto future generations. This aspect of CRISPR technology ignites significant debate among scientists, ethicists, and the public alike. One of the primary concerns regarding germline editing is the risk of unintended consequences. These can range from off-target mutations to unforeseen health effects in descendants.
The potential for germline modifications also raises profound moral questions. For instance, should scientists possess the power to reshape human genetics? The concept of "designer babies" leads to fears of exacerbating social inequality. If only the wealthy can afford genetic enhancements, this could deepen societal divides. Moreover, there is uncertainty surrounding the long-term ecological impacts of altering genes in a consistent and inheritable manner. These considerations emphasize the requirement for stringent oversight and ethical reflection in the field of gene editing.
Regulatory Frameworks
Regulatory frameworks are essential to ensure that CRISPR applications are developed and employed responsibly. Various countries have begun to establish guidelines and policies to govern gene-editing research. These frameworks aim to balance innovation with ethical practices, placing safety and societal considerations at the forefront.
In the United States, the National Institutes of Health (NIH) plays a pivotal role in overseeing research funded by federal grants. Meanwhile, the Food and Drug Administration (FDA) regulates products developed from genetic engineering, including those involving CRISPR technologies. Internationally, agreements like the Cartagena Protocol on Biosafety offer guidelines regarding genetically modified organisms (GMOs) and their movement across borders.
Ensuring that ethical considerations are integrated into the regulatory process is vital for the future of CRISPR technology.
However, the rapid evolution of the technology often outpaces current legislation. This gap necessitates ongoing discussions and the establishment of dynamic regulatory systems capable of adapting to new scientific discoveries and societal views. As a result, collaboration between scientists, policymakers, and ethicists will be critical in shaping the future landscape of CRISPR research while safeguarding against potential abuse and unintended consequences.
Limitations of Current CRISPR Technology
CRISPR technology, while revolutionary, is not without its limitations. Understanding these restrictions is crucial for accurate application and future advancements. Limitations in CRISPR can impact efficacy, safety, and the breadth of potential applications. Addressing these shortcomings is essential for researchers and practitioners in the field, as they shape the next steps in genetic research. This section explores two main areas of concern: the technical limitations of guide RNA and challenges associated with delivery systems.
Technical Limitations of Guide RNA
Guide RNA (gRNA) is a critical element in the CRISPR-Cas9 system. However, it presents several technical limitations that can hinder efficacies, such as:
- Design Complexity: Creating an effective guide RNA is not straightforward. The gRNA must have high specificity to target sequences within the genome. This specificity ensures that off-target edits are avoided. Identifying a suitable target sequence requires extensive analysis and trial-and-error approaches.
- Stability Issues: Guide RNA can suffer from stability problems. RNA molecules, including gRNA, are often prone to degradation. This limits their longevity in cellular environments, affecting the outcomes of experiments and treatments.
- Variability in Efficiency: The efficiency at which gRNAs direct Cas9 to make precise cuts can vary significantly. Some gRNAs perform well, while others may achieve only a low percentage of desired edits. This inconsistency can compromise experimental results and applicability in therapeutic contexts.
These technical limitations necessitate further investigation into optimizing gRNA design and enhancing its stability within cells.
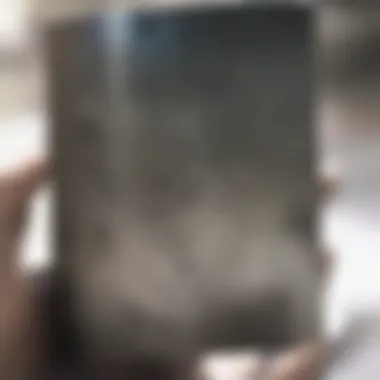
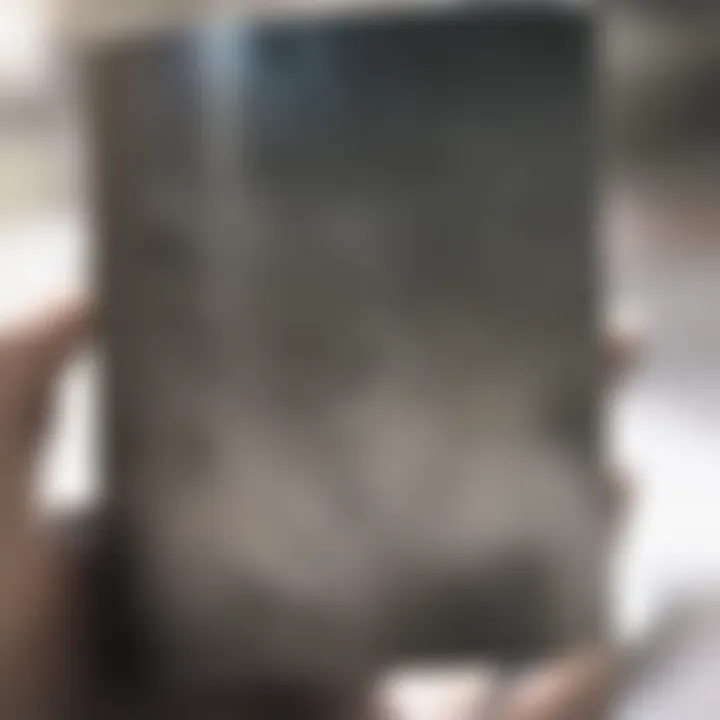
Challenges in Delivery Systems
The delivery of CRISPR components to target cells is another significant challenge. Effective delivery is imperative for maximizing the potential of CRISPR technology. Major challenges include:
- Delivery Methodology: Currently, there is no universal solution for delivering CRISPR systems. Different types of cells may require tailored delivery methods, making it difficult to find an efficient means for all cell types. Common methods include viral vectors and nanoparticles, each with its own set of limitations.
- Immune Response: The human body may recognize CRISPR components as foreign. This immune response can reduce the effectiveness of treatments. If immune reactions occur, they can lead to inflammation or neutralization of the therapeutic vector, inhibiting the intended gene editing effects.
- Efficiency of Cellular Uptake: Not all cells will effectively take up the CRISPR system. The efficiency of uptake can significantly influence the success of gene editing. Strategies to enhance cell uptake are an area of ongoing research.
Future Directions in CRISPR Research
The field of CRISPR technology is advancing at a rapid pace, with future directions promising to enhance its application and efficacy. This section discusses various innovations and potential paths for CRISPR research, particularly focusing on the evolution of guide RNA design and the possibility of multi-gene editing. Both areas present exciting opportunities that can significantly impact genetic research and therapeutic interventions.
Innovations in Guide RNA Design
The design of guide RNA is crucial in determining the success of CRISPR applications. Recent innovations have introduced various strategies aimed at improving guide RNA functionality and stability. One notable trend is the development of optimized guide RNA sequences that enhance targeting accuracy while minimizing off-target effects.
Researchers are also exploring alternative structures for guide RNA, such as circular RNA and chemically modified RNA, to improve stability and enhance the binding affinity with Cas9 proteins. The incorporation of specific motifs in guide RNA may also aid in increasing its efficiency, leading to higher rates of successful gene editing.
Several experimental approaches demonstrate promise:
- Modifications to Backbone: Altering the RNA backbone may contribute to improved performance during CRISPR applications.
- Inclusion of RNA Aptamers: Using aptamers to increase guide RNA interaction could enhance specificity and targeting.
- Dual-Guide Systems: Developing simultaneous usage of two guides, targeting different sites, may improve efficiency.
Ultimately, these innovations lay the groundwork for more precise applications of the CRISPR-Cas9 system, ensuring better outcomes in gene editing practices.
Potential for Multi-gene Editing
The ability to target multiple genes simultaneously represents a major leap forward in gene editing capabilities. Current CRISPR methodologies predominantly focus on single-gene modifications. However, multi-gene editing expands this scope and allows for comprehensive genetic interventions.
One promising avenue involves the coordination of multiple guide RNAs in a single delivery system. By leveraging advanced delivery mechanisms, researchers are capable of introducing various guide RNAs that can target distinct genes concurrently. This approach can be valuable in addressing complex genetic disorders requiring simultaneous modifications in multiple pathways.
Some benefits of multi-gene editing include:
- Enhanced Treatment Efficacy: Multiple pathways can be altered to produce a more robust therapeutic effect.
- Functional Studies: Facilitating the understanding of gene interactions can illuminate relationships between different genetic elements.
- Agricultural Advancements: Multi-gene editing can lead to improved crop traits, promoting higher yields and disease resistance.
As technologies continue to evolve, the potential for efficient multi-gene editing will likely drive significant advancements in both medical and agricultural fields, changing the landscape of genetic research and its applications for future generations.
"The future of CRISPR represents not only a tool for gene editing but also a paradigm shift in our understanding of genetics and therapeutic possibilities."
In closing, the future directions in CRISPR research stand to redefine the boundaries of genetic manipulation, combining theoretical advancements with practical applications, leading to a more nuanced understanding of biology and potential treatments.
Closure
The conclusion serves as the final articulative element of this exploration into guide RNA and its essential role in CRISPR technology. It synthesizes the foundational knowledge and practical applications of guide RNA. As we delve into the implications of this innovative genomic tool, it is clear how significant understanding guide RNA is for various fields. This knowledge informs ongoing research and the development of therapeutics, agricultural modifications, and more.
Summary of Key Points
Throughout this article, we have dissected critical components of guide RNA and its interaction with the CRISPR-Cas9 system. The following key points have emerged:
- Definition and Composition: Guide RNA is a complex molecule crucial for directing the Cas9 nuclease to specific genomic locations, facilitating precise gene edits.
- Types of Guide RNA: Distinctions between single-guide RNA and dual-guide RNA reveal diverse applications and efficiencies in genetic engineering tasks.
- Design Considerations: Successful implementation of guide RNA in CRISPR requires careful selection of target sequences and consideration of potential off-target effects.
- Applications: Guide RNA has shown promise across various domains, including gene editing in animal models, applications in agriculture, and potential therapeutic uses in humans.
- Ethical and Regulatory Aspects: Ongoing debates regarding the ethical implications of CRISPR technology highlight the importance of a claimed regulatory framework to guide research direction.
- Future Prospects: Innovations in guide RNA design may unlock greater precision in multi-gene editing and expand the boundaries of genetic research.
Implications for Future Studies
The implications of guide RNA and CRISPR technology for future studies are profound. As our understanding of gene expression and genetic modifications deepens, researchers will be better equipped to address complex biological challenges. Potential areas for further exploration include:
- Enhancing Guide RNA Design: Continuous improvement in guide RNA design tools may lead to increased specificity and decreased off-target effects, essential in sensitive applications such as therapeutic gene editing.
- Broader Applications: Expanding the scope of CRISPR applications beyond basic research to encompass therapeutic interventions for genetic disorders underscores the potential for significant societal impact.
- Ethical Frameworks: As technology advances, developing comprehensive ethical frameworks will be crucial to guide responsible research in the context of CRISPR, especially related to germline modifications.
- Interdisciplinary Collaboration: Future progress will likely benefit from collaboration between geneticists, bioethicists, and legislators, ensuring that technological innovations align with public values and ethical standards.
Understanding guide RNA within the CRISPR-Cas9 context not only marks a milestone in genetic engineering but also challenges pre-existing paradigms in medicine and agriculture. This complex interplay continues to hold enormous potential for future research, emphasizing the necessity for informed and careful exploration of its capacities.