In-Depth Analysis of Ha Tag DNA Sequences
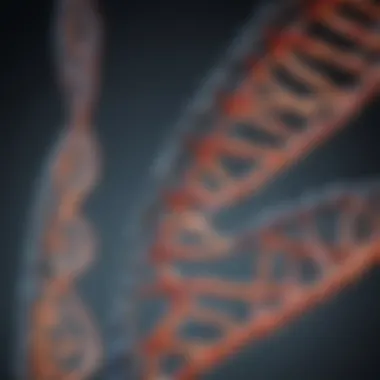
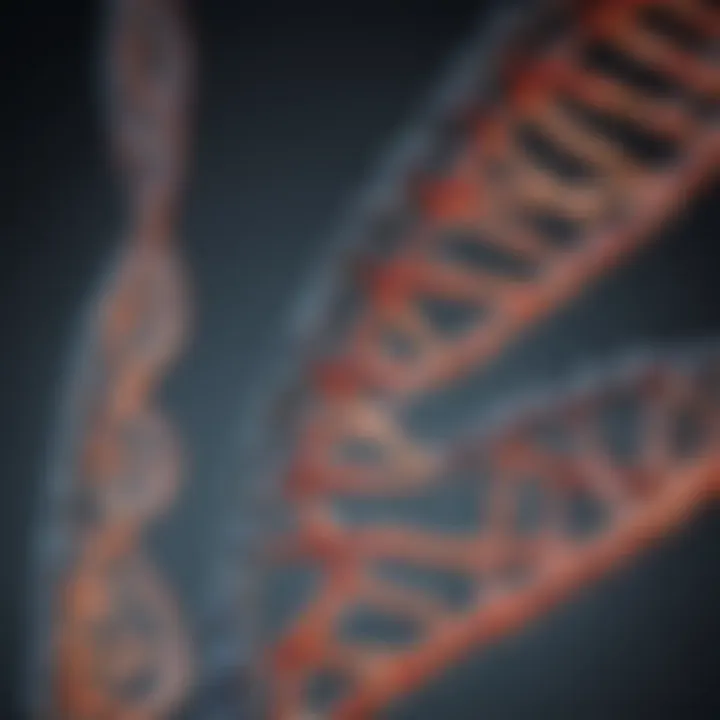
Intro
In the world of molecular biology, specificity and accuracy reign supreme when it comes to studying proteins and their functions. One of the tools that researchers have harnessed effectively over the years is the Ha tag—a short peptide sequence that allows for the precise detection and purification of proteins. This article dives into the pivotal role of Ha tag DNA sequences, highlighting their historical significance and the innovative methodologies that have evolved around them. This expertise not only illuminates their application in research but also paves the way for future advancements in biotechnology and therapeutic practices.
Research Overview
Summary of Key Findings
The intricate nature of Ha tags reveals numerous findings:
- Discovery: Ha tags originated as a methodological breakthrough, offering researchers an efficient means of tagging proteins without interfering with their normal biological functions.
- Applications: They have established their utility across various scientific disciplines, from fundamental research in gene expression to cutting-edge developments in gene therapy.
- Techniques: Emerging approaches for incorporating and detecting Ha tags continue to evolve, ensuring their relevance in modern scientific inquiry.
Background and Context
The historical journey of Ha tags began several decades ago when the need for reliable protein markers became evident. Researchers sought a solution that would allow for the tracking of proteins in live cells and experimental settings without disrupting their native behaviors.
The original synthesis of the Ha tag peptide sequence, inspired by similar existing markers, marked a turning point in molecular technology. Biochemists initially faced challenges such as ensuring specificity and avoiding cross-reactivity with other proteins. However, through persistent experimentation, they overcame these hurdles.
Ha tags gained acceptance for their compatibility with various protein structures, which contributed further to their adoption. Importantly, as emphasis on precision medicine and targeted therapies grew, so too did the relevance of these tags in tracking the expression of therapeutic proteins in clinical settings.
Methodology
Experimental Design
Within laboratory settings, the design of experiments employing Ha tags typically involves cloning the Ha tag sequence into the DNA construct of interest. Researchers may utilize various vectors to facilitate the incorporation of these tags into specific genes, ensuring eventual expression in host cells.
This tagging process often utilizes techniques such as PCR (Polymerase Chain Reaction) and restriction enzyme digestion, empowering scientists to accurately insert and express Ha tags within desired molecular frameworks. Consequently, tagged proteins can undergo purification through affinity chromatography or various detection methods, facilitating subsequent analysis.
Data Collection Techniques
To assess the efficacy of Ha tags, researchers employ several techniques:
- Western Blotting: Utilized to determine the presence of tagged proteins in various samples, allowing for molecular weight checks.
- Immunoprecipitation: Involves isolating the target protein through specific antibody binding, which is essential for analyzing interactions between proteins.
- Fluorescence Microscopy: Enables visualization at a cellular level, complementing data acquisition on protein localization.
A detailed account of these methodologies offers insight into how advancements in tagging technologies continue to explore uncharted territories in molecular biology. In considering the implications of Ha tags, researchers can ensure their techniques remain robust and productive, enhancing our understanding significantly.
"In molecular biology, precision in measurement and observation is the key to breakthroughs. Ha tags have bolstered this precision immensely."
As we dive deeper into their applications, the foundational understanding provided here serves to frame the larger scope of the discussion surrounding Ha tag DNA sequences.
Prelims to Ha Tag DNA Sequences
The realm of molecular biology hinges on a variety of techniques and tools that enable researchers to decipher the complexities of biological molecules. Among these tools, Ha tag DNA sequences have carved a niche for themselves. Understanding Ha tags is not just about recognizing a sequence; it represents an essential anchor in the dynamic world of protein research. These sequences have significantly revolutionized the methodologies used in protein expression and purification, facilitating a deeper dive into cellular processes.
Definition and Basic Concept
Ha tags, or hemagglutinin tags, are short peptide sequences derived from the influenza virus. Typically consisting of nine amino acids, the most common Ha tag sequence is YPYDVPDYA. This tag plays a critical role in biotechnology as it provides a straightforward method for protein identification and purification. Researchers attach the Ha tag to recombinant proteins. This allows scientists to employ various methods, like immunoprecipitation and chromatography, to selectively capture tagged proteins from complex mixtures. Therefore, the tag adds a layer of simplicity, enabling detailed study of protein structure and function without the noise of unwarranted contaminants.
Moreover, the Ha tag is particularly favored because it is small enough to minimize interference with protein functionality. Unlike larger tags, which may alter protein folding or activity, the proper positioning of an Ha tag typically preserves the biological nature of the protein under investigation.
Historical Background
The journey of Ha tags began with the advent of biotechnology in the late 20th century. As molecular cloning techniques advanced, the necessity for reliable tagging systems became evident. This need drove scientists to explore various methods for protein characterization and validation. The discovery of the hemagglutinin protein from the influenza virus in the 1980s marked a turning point in this quest.
The initial applications of Ha tags were modest, focusing primarily on simple detection assays. Over the decades, the increasing sophistication of analytical techniques led to a broader range of applications. Notably, the introduction of monoclonal antibodies significantly enhanced the ability to isolate and study tagged proteins. As the understanding of protein interactions and functions deepened, the role of Ha tags evolved from mere identifiers to crucial components in complex protein studies.
Through trials, successes, and a few setbacks, Ha tags carved their mark in molecular biology and now stand as an indispensable tool in various research fields. This historical context illustrates not only the technical evolution of Ha tags but also their significance in pushing the boundaries of scientific inquiry.
"The integration of Ha tags in molecular studies has profoundly changed the way researchers approach protein analysis. They provide a link between basic research and practical applications in biotechnology and therapeutic development."
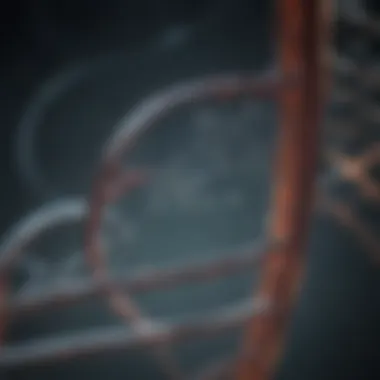
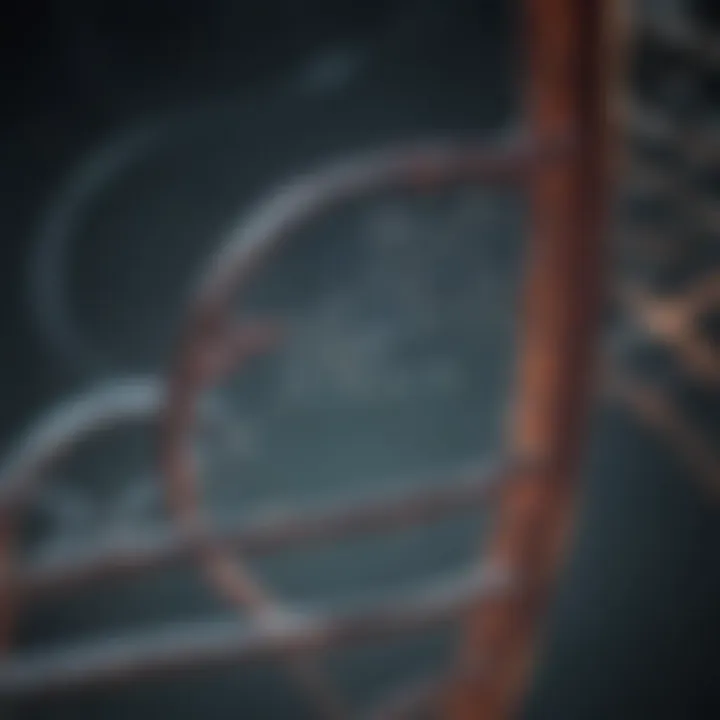
Understanding the past helps illuminate the path forward, as we explore the biochemical properties and innovative applications of Ha tags in the subsequent sections.
Biochemical Properties of Ha Tags
Understanding the biochemical properties of Ha tags is crucial for anyone involved in molecular biology or related fields. The characteristics of these tags directly influence their efficacy and reliability in various applications, such as protein tagging, purification, and detection. Knowing the amino acid composition and structural attributes of Ha tags can guide researchers to make informed decisions in experimental design and technique selection, ultimately leading to clearer, more reproducible results.
Amino Acid Composition
The amino acid composition of Ha tags plays a significant role in how these tags interact with proteins and other molecules. Typically, Ha tags are short peptide sequences that consist of hydrophobic and hydrophilic residues, impacting solubility, stability, and overall functionality. The most notable Ha tag, the HA (hemagglutinin) tag, features a sequence derived from the influenza virus, which makes it particularly effective at binding to antibodies that recognize this specific epitope.
Key considerations regarding amino acid composition include:
- Hydrophilicity vs. Hydrophobicity: Tags often have a balanced combination of both hydrophilic and hydrophobic side chains which is essential for their proper folding and solubility in different buffers.
- Charge: The presence of positively or negatively charged amino acids can affect how the tag interacts with its environment, including other proteins, nucleic acids, and membrane structures.
- Length and Flexibility: The length of the tag can influence the sterics of protein interactions. Too long a sequence may hinder accessibility while a too short tag may not be functional enough.
Moreover, when considering variations of the HA tag, mutations in specific amino acids could enhance affinity for antibodies or alter resistance to proteolytic cleavage, thus tailoring the tag’s properties for specific experimental setups.
Structural Characteristics
The structural characteristics of Ha tags also warrant careful examination, as they ultimately determine how effectively these tags can bind to target proteins and facilitate downstream applications such as purification and detection. Typically, the HA tag and similar sequences display a unique blend of secondary structures such as alpha helices and beta sheets that help maintain their stability.
- Conformation: The conformation of the Ha tag is crucial for accurate recognition by anti-HA antibodies. A misfolded peptide can lead to poor binding efficiency, which could skew experimental outcomes.
- Surface Accessibility: For the entire tagging system to function efficiently, the HA tag must be conveniently positioned on the protein surface. This ensures that any binding partners can easily interact without steric hindrance.
- Interactions with Host Proteins: Understanding how Ha tags might interact with native cellular proteins is important. Sometimes tags can inadvertently become part of interactions that affect protein function or localization.
"The structural design of protein tags is not just about recognition; it’s about context, functionality, and predictability in biological systems."
By appreciating these biochemical properties, researchers can manipulate Ha tags to enhance their effectiveness, streamline protocols, and ultimately drive forward breakthroughs in fundamental and applied sciences. Researchers may also explore novel tag modifications to customize their features, making tags versatile tools in protein manipulation.
Development of Ha Tag Technologies
The evolution of Ha tag technologies plays a critical role in harnessing the potential of molecular biology. This advancement has opened several doors, enabling researchers to employ practical techniques in various biological applications. Ha tags serve as vital tools in protein analysis, allowing for precise tracking and manipulation of proteins in different environments. It’s not just about having a tag; it’s about how these technologies are integrated into experimental protocols to maximize effectiveness and accuracy.
Synthetic Approaches
When we talk about synthetic approaches in Ha tag technologies, we refer to the deliberate design and construction of these tag sequences. Synthetic methods have revolutionized how we produce protein tags, providing researchers with the means to create bespoke solutions tailored to their specific needs. Here are some of the key points:
- Customizability: Synthetic approaches allow for fine-tuning of the amino acid sequence. This customizability enhances binding efficiency and specificity in various assays.
- Scalability: With the ability to synthesize DNA sequences on a large scale, scientists can produce a high volume of Ha tags, thus enabling widespread use in different research settings.
- Quick Turnaround: Instead of relying on plasmid constructs, synthetic approaches often provide faster results, allowing for rapid experimentation and analysis.
For instance, in research where specific protein interactions need to be evaluated, synthetic Ha tags can be engineered to include specific motifs that increase binding affinity to target proteins. With such flexibility, researchers are not only limited by natural occurrences but can also explore novel interactions and mechanisms.
Natural Occurrence
Natural occurrences of Ha tags also deserve consideration, as they reveal the biological trafficking systems utilized by organisms. Many proteins in nature have evolved mechanisms to facilitate their localization and function, which can inspire the design of synthetic tags. Some essential aspects to note include:
- Evolutionary Insights: By studying how proteins are tagged and modified in their natural environments, researchers can glean insights into evolutionarily beneficial tagging mechanisms. This understanding opens pathways to develop more efficient synthetic tags.
- Biocompatibility: Utilizing naturally occurring tags ensures better acceptance within biological systems, reducing the risk of immune responses or dysfunctions which synthetic tags might provoke. Biocompatibility is critical in therapeutic applications, which may eventually utilize Ha tags.
- Comparative Effectiveness: Understanding naturally occurring tags allows researchers to compare their functionality with synthetic counterparts, leading to improved design and engineering of these constructs.
In sum, the development of Ha tag technologies through both synthetic approaches and the study of natural occurrences offers a broad spectrum of opportunities for innovation in molecular biology.
"Understanding the balance between synthetic ingenuity and natural mechanisms is key in advancing Ha tag technologies."
These emerging methods and insights directly impact our capacity to manipulate proteins, guiding future research toward more effective and tailored applications. As we continue to explore this field, we may very well find new frontiers that stretch the boundaries of our understanding and capabilities.
Applications in Molecular Biology
Molecular biology thrives on the intricate interplay between DNA sequences, proteins, and cellular mechanisms, where Ha tags serve as pivotal tools. These short peptide sequences are strategic assets in various research applications, streamlining processes like protein expression, purification, and gene regulation—all fundamental components of molecular biology.
Among the foremost benefits of utilizing Ha tags is their versatility. Researchers can attach Ha tags to proteins of interest, enabling easy identification and isolation. This not only saves time but also enhances accuracy, which is essential in experimental reproducibility. Moreover, given the quick pace of technological advancements, integrating Ha tags into modern genetic manipulation techniques continues to gain importance.
Protein Expression Studies
In the realm of protein expression, Ha tags stand out due to their instrumental role in monitoring and enhancing protein production. By inserting Ha tag sequences into the plasmids that express target proteins, researchers can track the expression levels via various detection methods, such as western blotting or ELISA. This simplifies the quantification of proteins and allows scientists to adjust expression conditions and improve yields.
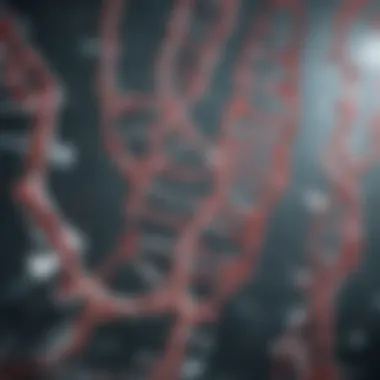
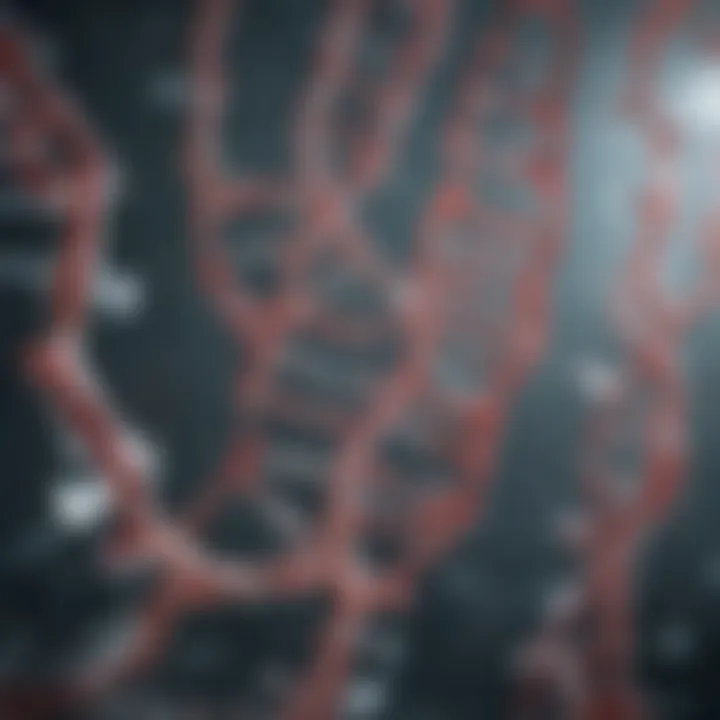
Just imagine a scenario where researchers are developing a new vaccine; having a reliable way to validate the protein expression levels rapidly can mean the difference between timely progress and unnecessary delays. Knowledge of how much protein is being produced, along with its functional integrity, becomes critical here.
"Ha tags are like traffic lights in the busy world of protein expression—helping researchers to spot progress and avoid collisions with unforeseen issues."
Protein Purification Techniques
The purification of proteins is another domain where Ha tags shine brightly. By using affinity chromatography, researchers can leverage the binding properties of Ha tags to simplify the purification process. A column containing antibodies specific to Ha tags allows for the selective isolation of tagged proteins from complex mixtures.
This technique is particularly beneficial when dealing with crude extracts that may contain a cocktail of undesired proteins. The attributes of Ha tags enhance not only the selectivity but also the efficiency of the purification process.
A typical workflow may involve:
- Cell lysis to extract proteins from cultured cells.
- Column equilibration using buffer to prepare for sample application.
- Binding phase, where tagged proteins adhere to the column.
- Elution phase, collecting purified proteins that can now be analyzed for further applications such as functional assays or crystallography.
Gene Regulation and Expression
Exploring the combined abilities of Ha tags in gene regulation opens another avenue of investigation. By employing techniques like chromatin immunoprecipitation (ChIP), researchers can determine the interaction between tagged proteins and specific DNA regions. Such studies elucidate the role of various proteins in the regulation of gene expression, thus impacting our understanding of cellular responses under different conditions.
For instance, in studying the expression of a gene that responds to stress, scientists can engineer a plasmid with both the gene of interest and an Ha tag. This allows further analysis on how different environmental factors might upregulate or downregulate the gene expression.
Ha tags, therefore, are not merely markers; they are essential tools that assist in unraveling the complex mechanisms underlying gene expression. Their applications in molecular biology contribute significantly to both fundamental research and applied sciences.
In short, Ha tags enhance the efficiency and accuracy of processes in molecular biology, offering numerous advantages that continue to underscoredive the field's expansion. As techniques advance, the potential for these tags to uncover further biological insights remains immense.
Innovative Techniques in Ha Tag Research
In the ever-evolving world of molecular biology, the introduction of innovative techniques in Ha tag research forms a crucial part of developing our understanding and applications of these sequences. These methods enable researchers to manipulate and study proteins in ways that were once deemed impossible. With a focus on PCR and cloning methods and mass spectrometry applications, this section explores the significance, advantages, and challenges associated with these leading-edge strategies.
PCR and Cloning Methods
Polymerase Chain Reaction (PCR) has transformed molecular biology, making the amplification of specific DNA sequences not just a possibility but a routine part of laboratory practice. When it comes to Ha tags, PCR allows scientists to quickly generate large quantities of tagged genes, facilitating their study in various contexts.
The process begins simply; a mixture of DNA template, primers, nucleotides, and polymerase is cycled through a series of temperature changes. It’s like a well-rehearsed dance where every participant has a role to play, ultimately leading to the creation of numerous copies of the desired segment. In the case of Ha tags, direct integration into plasmids can be achieved, allowing for seamless gene expression studies.
Some benefits of utilizing PCR in Ha tag research include:
- Speed and efficiency: Amplifying desired sequences can be accomplished in a few hours, significantly reducing the time spent in the lab.
- Precision: Tailored primers can contain the Ha tag sequence, ensuring that the end product is compatible with further experiments.
- Versatility: PCR can be adapted for various applications, including site-directed mutagenesis, which can modify proteins for specific research goals.
However, PCR is not without its pitfalls. The potential for non-specific amplification can lead researchers down the wrong path if caution isn’t exercised. Careful primer design and reaction conditions must be optimized to ensure the reliability of results.
Mass Spectrometry Applications
Mass spectrometry (MS) is another cornerstone technique that has made noticeable waves in the field of Ha tag research. Known for its sensitivity and accuracy, mass spectrometry can analyze the molecular weight and sequence of proteins, allowing for a better understanding of both structure and function.
In practice, mass spectrometry can identify the presence of Ha tags on proteins and quantify their abundance within complex mixtures. This characterisation is vital for studies in protein interactions and post-translational modifications, enabling scientists to pinpoint exact biochemical processes.
Some key advantages of employing mass spectrometry in Ha tag research include:
- High resolution: MS can differentiate between very closely related protein forms, invaluable for distinguishing between tagged and untagged versions.
- Broad applicability: Mass spectrometry is well-suited for various sample types, from bacterial lysates to mammalian cells.
- Quantitative capabilities: Researchers can easily assess the amount of tagged protein present, which is crucial for understanding its functional roles.
However, mastering mass spectrometry requires a steep learning curve. Moreover, there’s the risk of cross-reactivity with other proteins. It’s essential to lift various complexities, ensuring that the data obtained is both accurate and interpretable.
"Innovative methods are the lifeblood of science; without them, we remain stuck in the past, unable to explore the full potential of our discoveries."
By embracing these innovative techniques, researchers not only push the boundaries of knowledge surrounding Ha tags but also enhance their overall understanding of molecular mechanisms. The integration of PCR and mass spectrometry has elevated research possibilities, transforming ideas from mere conjecture into tangible findings, ultimately paving the way for breakthroughs in biotechnology and therapeutic applications.
Challenges in Ha Tag Utilization
The incorporation of Ha tags in molecular research is not without its hurdles. Although these sequences boast numerous benefits, their actual implementation faces a variety of challenges that can complicate the execution of experiments and affect the overall results. When diving into the intricacies of Ha tag utilization, one must acknowledge elements such as cross-reactivity issues and stability concerns.
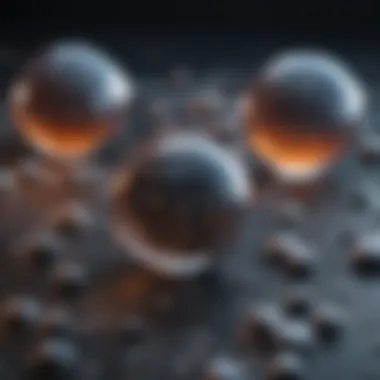
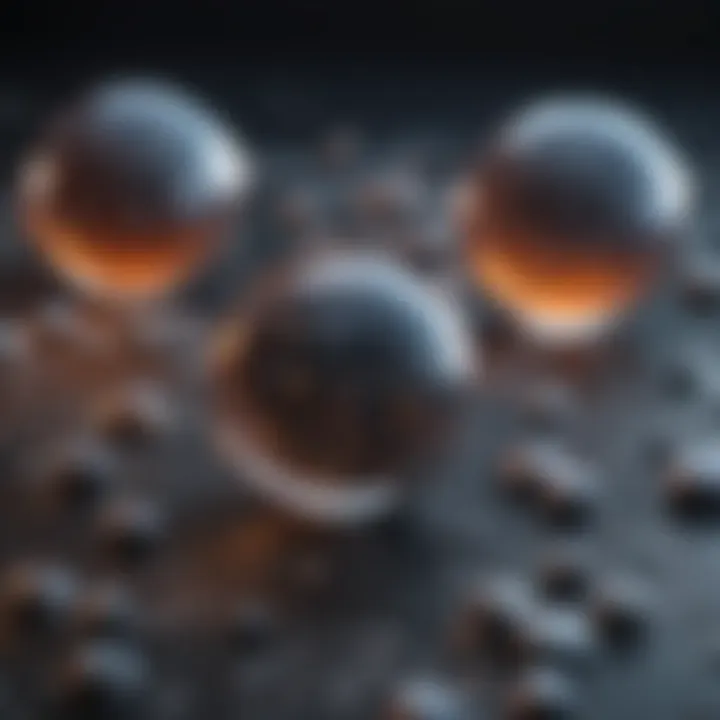
These challenges are critical not only because they can hinder the effectiveness of the Ha tags themselves but also because they can have cascading effects on experimental outcomes, data interpretations, and even subsequent applications in biotechnology. Understanding these obstacles is essential for researchers aiming to deploy Ha tags successfully while ensuring robust and reliable results.
Cross-Reactivity Issues
One significant hurdle tied to Ha tags is cross-reactivity. This phenomenon occurs when antibodies or other reagents bind to unintended targets, leading to potential false positives in protein assays. Imagine conducting an experiment where you're searching for a specific protein, only to end up with a clouded interpretation due to interference from similar, yet irrelevant, proteins. The implications can be serious, particularly in contexts like drug development and diagnostic assays where precision is paramount.
Several factors contribute to cross-reactivity:
- Structural similarities between proteins can mislead detection methods.
- Binding affinities of antibodies may vary significantly, increasing the risk of observational errors.
- Environmental variables, such as pH and ionic strength, can alter interaction dynamics, complicating results further.
While researchers can mitigate some of these issues through careful selection of antibodies or reagents, achieving complete specificity remains a challenge. Reference to resources such as Wikipedia can provide a broader understanding of how cross-reactivity can affect not just Ha tags, but protein assays at large.
Stability Concerns
Stability can make or break the effectiveness of Ha tags in practical applications. If the constructs containing the Ha tag are not stable, they might degrade quickly under normal laboratory conditions, which can lead to compromised experimental data. This issue often manifests in two ways:
- Chemical instability—where the tag itself might undergo modifications under varying conditions, potentially altering its function.
- Physical instability—where the tagged proteins might aggregate or precipitate, leading to low yields or inactive protein preparations.
Researchers can face a real dilemma when it comes to this challenge. On one hand, it’s crucial to maintain conditions that promote stability, such as optimizing buffer compositions and temperature. On the other hand, over-engineering these conditions can lead to increased costs and resource use. Consideration of storage solutions becomes critical as well. For example, utilizing proper storage conditions such as -80°C freezers or specialized stabilizing agents can help preserve the integrity of Ha-tagged proteins.
As we look toward the future of Ha tag research, these foundational hurdles must be tackled head-on. Only then can we unlock the full potential of these remarkable sequences.
Regulatory Considerations
In the world of molecular biology, the adoption and application of Ha tag DNA sequences come with a heap of regulatory considerations that scientists must navigate. These guidelines not only govern how we utilize these sequences for research but also ensure safety in experimental environments. The significance of regulatory aspects extends to fostering public trust and ensuring that findings benefit society without compromising ethical standards. Thus, it becomes essential to grasp the various elements that inform these regulations.
Safety and Compliance
Safety considerations take center stage when engaging with Ha tags, especially in contexts involving human or animal subjects. Laboratories handling Ha tag-related experiments must adhere to local and international safety protocols to minimize risks.
- Biohazard Protocols: Working with genetically modified organisms (GMOs) demands stringent biosafety measures. Institutions often implement tiers of containment based on potential risks. For instance, if a Ha tag is fused to a pathogen, it will fall under higher containment levels.
- Quality Control: Compliance testing ensures that products developed using Ha tags are free from contaminants and function as intended. Rigorous analysis is conducted to validate the sequences, which can prevent erroneous results and implications in clinical settings.
- Responsible Disposal: It all boils down to the disposal of biological waste; following proper protocols prevents contamination of waste sites. Adhering to thoughtless disposal practices can lead to serious ecological and health implications.
Ethical Implications
The ethical considerations surrounding the use of Ha tags delve into profound philosophical and societal questions. Many researchers today are acutely aware of the ethical framework needed when engaging in genetic manipulation or biological research. There are a couple of key areas where ethical reflections are tremendously relevant:
- Human Gene Editing: With the ability to tag proteins potentially influencing gene expression, the moral implications of altering human DNA cannot be overlooked. Society grapples with the risks associated to gene editing. Where do we draw the line?
- Transparency: Engaging in open communication about the methodologies employed in tag research is paramount. Failing to share critical information with the public might stir mistrust within communities. Sharing findings openly can lead to constructive dialogue while addressing concerns over safety and efficacy.
- Sustainability: Research practices must reflect environmental consciousness. As we make strides in biotechnology using Ha tags, we must be conscious of our ecosystem and endeavor to limit our footprint.
"An ounce of prevention is worth a pound of cure."
This age-old adage rings true in the context of regulatory scrutiny. Being proactive about safety, compliance, and ethical standards can pave the road for fruitful research ventures.
Future Directions in Ha Tag Research
The landscape of molecular biology is evolving at a pace that demands we keep our finger on the pulse of emerging trends, especially when we consider the implications of Ha tag DNA sequences. Understanding the future directions in Ha tag research is crucial, as these paths will likely influence not only basic science but also the applications that can revolutionize clinical practices and therapeutic strategies.
Emerging Technologies
The exploration of new technologies holds a promising future for Ha tag applications. Advances in biotechnology are rapidly progressing, leading to innovative methods that enhance the precision and efficiency of Ha tag implementation. Some key technologies poised to influence Ha tag research include:
- CRISPR-Cas9: This gene-editing tool can be optimized to integrate Ha tags directly into target genes, improving precision in protein tagging and tracking.
- Next-Generation Sequencing (NGS): With NGS, researchers can analyze vast amounts of sequencing data, providing insights into Ha tag effectiveness across different organisms and conditions.
- Quantitative Mass Spectrometry: Advances in mass spectrometry enable more accurate quantification of tagged proteins, thus offering a clearer picture of protein dynamics.
Furthermore, the coupling of Ha tags with bioinformatics tools allows for sophisticated data analysis related to protein interactions and functionality. By harnessing these technologies, researchers can delve deeper into the complexities of protein networks and molecular pathways, ensuring a more nuanced understanding of biological systems.
Potential Therapeutic Applications
As we gaze into the future, potential therapeutic applications of Ha tags start to emerge, glimmering with promise. The ability to tag proteins has vast implications in various fields, particularly in therapy development. Some noteworthy applications include:
- Personalized Medicine: The incorporation of Ha tags can enable the development of personalized therapies by tracking patient-specific proteins. This customization can lead to more effective treatments with minimized side effects.
- Cancer Therapy: Ha tags can be used to evaluate the efficacy of new cancer drugs by tagging specific proteins involved in tumorigenesis, making it easier to measure responses in real-time.
- Vaccine Development: Innovations in tagged proteins can assist in creating more effective vaccines by ensuring robust immune responses. Tracking these proteins can lead to better understanding of how immunity can be cultivated and sustained.
Utilizing Ha tags in these avenues not only enhances understanding but also opens doors for novel treatments that leverage molecular biology's frontiers.
"Understanding the potential of Ha tags in therapeutic applications is not just about technology; it’s about reimagining our approach to health and disease management."
As we tread forward, a collaborative approach linking academia and industry will be fundamental in bridging the gap between laboratory findings and practical applications. The ability to effectively utilize Ha tags could bring significant advancements in medicine, offering a glimpse into a future enriched by scientific breakthroughs.