Understanding Inertial Motion Tracking: Principles & Uses
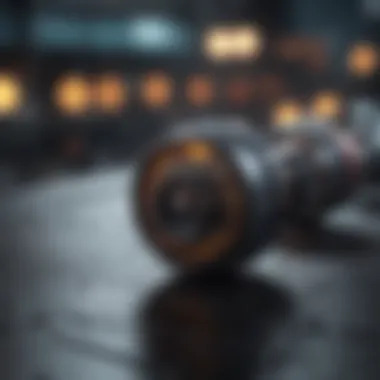
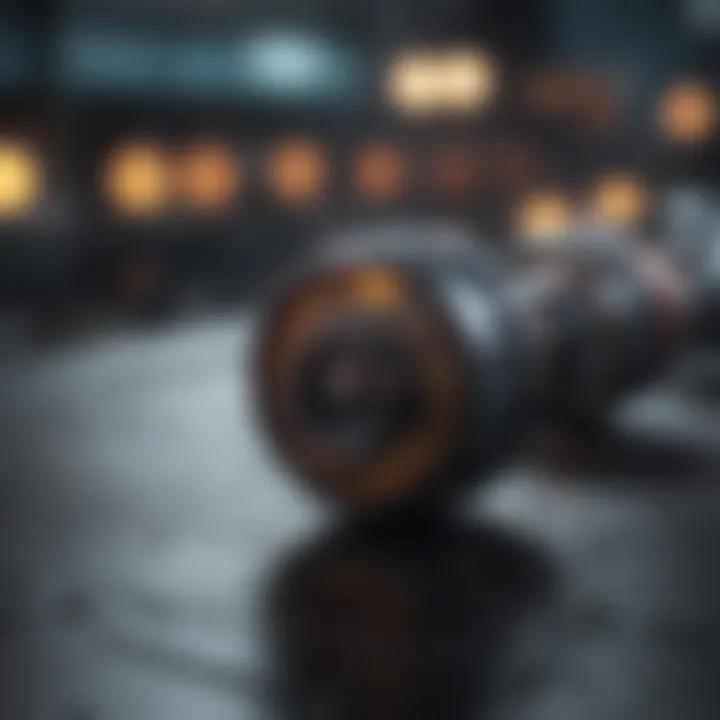
Intro
In the rapidly evolving world of technology, the capacity to track motion accurately and efficiently is becoming increasingly crucial. Inertial motion tracking stands out as a compelling solution, interconnected with a range of applications across various sectors. Whether in healthcare, robotics, or virtual reality, understanding how motion is tracked and the technologies employed is fundamental to leveraging its full potential.
This article aims to explore the core principles and transformative applications of inertial motion tracking, providing insights into the underlying technologies and the challenges faced in this dynamic field. With so many layers to dissect, delving into this topic opens up new discussions about its relevance in modern society.
Research Overview
Summary of Key Findings
After thorough investigation, several key findings emerge about inertial motion tracking:
- Inertial sensors, chiefly accelerometers and gyroscopes, play a pivotal role in capturing motion.
- The applications are diverse, impacting sectors like biomechanics in healthcare, precision agriculture, and even enhanced gaming experiences.
- Despite its advantages, challenges such as sensor drift and environmental factors present obstacles to accuracy.
Background and Context
The roots of inertial motion tracking can be traced back several decades. Initially, it found its footing in aerospace and defense. However, as technology advanced, its relevance expanded to consumer electronics and beyond. Over the years, researchers have fine-tuned the principles that govern the performance of various sensors, leading to today’s sophisticated systems that can analyze movements in real time.
The integration of inertial tracking technologies with algorithms has been a game-changer, enabling compact devices to understand complex motion patterns. As modern applications become increasingly reliant on real-time data, the capabilities of inertial motion tracking systems continue to grow, hinting at promising advancements on the horizon.
Understanding Inertial Motion Tracking
Inertial motion tracking is an increasingly vital component in various technological landscapes. Understanding this area not just involves grasping the mechanics behind it but also recognizing its vast applications across diverse fields. From healthcare providers using these systems to enhance patient outcomes to autonomous systems relying on accurate tracking for effective navigation, the significance of inertial motion tracking is evident everywhere.
As the title implies, comprehending inertial motion tracking requires a dive into its fundamental principles and its evolution over time. This intricacy is not merely academic; it shapes how we interact with technology in our daily lives. For instance, think about how wearable fitness trackers help monitor our health or how drones fly seamlessly through complex environments. These advancements are intertwined with the principles of inertial motion tracking.
Defining Inertial Motion Tracking
Inertial motion tracking refers to the method of determining the position, velocity, and acceleration of an object based on its movement and changes in its orientation. It utilizes inertial sensors—like accelerometers and gyroscopes—to measure the forces acting on an object as it moves. This understanding forms the bedrock upon which further applications and technologies are built.
The operation of inertial tracking relies heavily on the fundamental principle of inertia, which states that an object will remain at rest or in uniform motion unless acted upon by an external force. This mechanic illustrates that understanding the motion of the object involves not only tracking current positions but also predicting future movements based on its current velocity and acceleration. This can be particularly useful for various applications in sectors like healthcare, where tracking patient movements can inform rehabilitation processes.
Historical Context
The roots of inertial motion tracking can be traced back to the early work of Sir Isaac Newton, who laid the groundwork for modern mechanics in the late 17th century. The advent of motion sensors began to take shape during World War II, mainly to improve aircraft navigation systems. Over the decades, advancements in technology have led to notable developments, including the miniaturization of sensors and the refinement of data processing algorithms.
In the late 20th century, we saw a significant evolution in inertial measurement systems, primarily with the introduction of microelectromechanical systems (MEMS). These compact sensors made it possible for everyday devices—like smartphones and smartwatches—to incorporate motion tracking capabilities that weren't feasible before. This transformation is a testament to how the principles of inertial motion tracking adapted alongside technological advancements, helping create a foundational system that underscores many applications today.
Additionally, various fields have begun to recognize the potency of inertial motion tracking. From military applications, such as missile guidance systems, to fitness and virtual reality devices, nearly every sector stands to gain from understanding how motion tracking works. The history of this technology illustrates its pivotal role in shaping both current practices and future innovations.
Fundamental Principles
Understanding the fundamental principles behind inertial motion tracking is crucial for grasping the technological advancements and the wide-ranging applications it supports. These principles allow users to process movement data effectively, whether in healthcare, robotics, or virtual environments. The significance of these principles lies in their capacity to enable systems to predict movement trajectories accurately, all while possessing minimal human intervention.
Inertial motion tracking operates primarily through Newtonian mechanics and the principle of inertia, each playing a pivotal role in how motion is quantified. As this technology matures, being aware of these underlying principles is necessary, particularly when considering their influences on performance metrics in various fields. The implications stretch from precise motion capture systems to innovations in biomechanics, making it a fertile ground for exploration.
Newtonian Mechanics Overview
Newtonian mechanics forms the bedrock of our understanding of physical motion. At its core lies Newton's three laws of motion, fundamental in explaining how objects behave under various forces. These laws relate directly to inertial motion tracking, where sensors measure accelerations and angular velocities to provide insights into movement.
- First Law (Law of Inertia): An object at rest stays at rest unless acted upon by a net force. This principle is the essence of why unexpected movements can trigger errors in data collection in motion tracking systems.
- Second Law (F=ma): The relationship between an object's mass, its acceleration, and the applied force illuminates the dynamics of motion. Hence, the design of algorithms for interpreting sensor data often makes use of this foundational equation.
- Third Law (Action-Reaction): For every action, there is an equal and opposite reaction. In motion tracking, this law is vital in understanding how physical forces interact, particularly in applications like virtual reality, where user interactions count heavily.
To summarize, a sound grasp on Newtonian mechanics not only helps in effectively utilizing inertial measurement units but also allows for a deeper evaluation of data interpretations derived from sensor outputs.
The Role of Inertia
Inertia serves as a guiding concept in both physics and inertial motion tracking. Essentially, inertia is the tendency of an object to resist changes in its state of motion. In practical terms, it explains why heavy objects are more challenging to budge than lighter ones. Within motion tracking, sensors measure motion by calculating rates of change in velocity; inertia impacts how these sensors react to external forces.
In applications ranging from sports analytics to rehabilitation, understanding how inertia affects an object's trajectory and movement dynamics is paramount. Practitioners can leverage this insight to optimize performance or rehabilitate injuries more effectively. Some aspects of inertia that warrant attention include:
- Static vs. Dynamic Inertia: Knowing how an object behaves when at rest versus in motion can affect data accuracy.
- Inertial Damping: This is crucial for eliminating unexpected noise in sensor data, which can derive from rapid movements or environmental conditions.
"Inertia is not just an abstract principle; it is a guiding force behind how we experience and interpret motion every day."
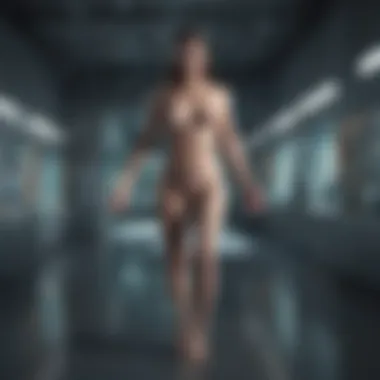
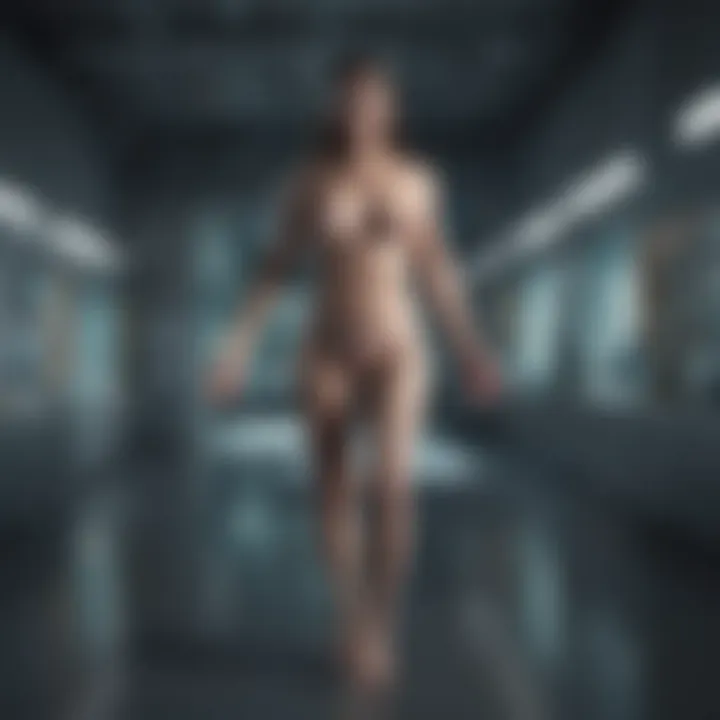
Key Technologies in Inertial Motion Tracking
Inertial motion tracking has dramatically changed the way we understand and utilize motion data in various fields. The advancements in technology, specifically regarding the tools and methodologies employed, have made it feasible for professionals to harness this information for practical applications. This section will detail the essential technologies that underpin inertial motion tracking and explain their significance. By recognizing how these technologies work together, students, researchers, and professionals can gain insights useful for their explorations and implementations.
Inertial Measurement Units (IMUs)
Inertial Measurement Units, or IMUs, are the backbone of inertial motion tracking systems. These devices combine multiple sensors to capture motion data in three dimensions. IMUs typically comprise accelerometers, gyroscopes, and sometimes magnetometers, each contributing a unique aspect of measurement.
- Accelerometers measure linear acceleration along one or more axes. They indicate how fast an object is speeding up or slowing down.
- Gyroscopes assess angular velocity, providing insights into how fast an object is rotating. If one imagines a spinning top, the gyroscope could indicate the speed of its spin.
- Magnetometers can offer orientation in relation to the Earth's magnetic field, which can complement the data collected by the other sensors.
The combination of these sensors allows IMUs to provide accurate and detailed motion tracking information. This is essential in applications ranging from navigation systems in vehicles to motion capture in virtual reality experiences. Without IMUs, achieving the level of precision required in modern applications would be exceptionally challenging.
Sensor Types: Gyroscopes and Accelerometers
Diving deeper, the two main types of sensors—gyroscopes and accelerometers—merit further exploration. Both sensors serve distinct purposes yet work closely to deliver comprehensive results.
Gyroscopes, originally used in various engineering applications, have evolved significantly. Modern MEMS (Micro-Electro-Mechanical Systems) gyroscopes are compact and highly accurate, which allows them to be integrated into consumer electronics. Their ability to maintain accurate orientation even with rapid movements is crucial for gaming devices and mobile phones.
Accelerometers, on the other hand, are equally critical. They are often found in smartphones and fitness trackers. Users might consider how their phone counts steps. It relies on accelerometer data, processing changes in position to gauge activity levels.
- Advantages of Gyroscopes:
- Advantages of Accelerometers:
- High precision in rotational tracking.
- Immediate response to changes in motion.
- Simplicity and widespread use in consumer devices.
- Effectiveness in measuring static and dynamic acceleration.
When combined, these sensors enable applications that require nuanced movement detection, including robotics and automotive technologies.
Fusion Algorithms for Data Interpretation
The raw data generated from IMUs needs thorough processing to extract useful insights. This is where fusion algorithms come into play. These algorithms combine data from different sensors—accelerometers, gyroscopes, and sometimes magnetometers—into a unified motion profile.
Consider a scenario where an IMU measures abrupt movements, such as a quick turn in a vehicle. Raw data alone may yield inconsistencies due to sensor noise or lag. Fusion algorithms enhance the accuracy by applying principles like Kalman filtering or complementary filtering.
"Fusion algorithms are essential for refining motion data, ensuring that interpretation aligns closely with real-world conditions, mitigating noise from each sensor's output."
Through these processes, user applications in numerous domains, including aviation and healthcare, benefit significantly. In aviation, accurate tracking is crucial for aircraft navigation, whereas in healthcare, patient rehabilitation can be enhanced with precise movement data.
Closure
Inertial motion tracking is at a fascinating intersection of technology and application. By harnessing the capabilities of IMUs, gyroscopes, accelerometers, and sophisticated fusion algorithms, we continue to push the envelope across multiple sectors. As technology advances, we can expect further refinements in motion tracking accuracy, usability, and integration across diverse platforms. For those keen to dive deeper, resources like Wikipedia, Britannica, and discussions on Reddit can provide additional insights.
Application Domains
Inertial motion tracking has woven itself into the fabric of many sectors, offering solutions and enhancements that were once the stuff of science fiction. From the realm of healthcare to the world of virtual reality, the spectrum of applications is wide and varied. Understanding these application domains can illuminate their respective challenges and benefits. Here, we will dive into four critical areas where inertial motion tracking is making a significant impact.
Healthcare and Rehabilitation
In recent years, the importance of inertial motion tracking in healthcare has surged. This technology allows medical professionals to monitor patients' physical movements with astonishing precision. For instance, sensors can track a patient’s gait, providing valuable information for rehabilitation programs tailored to individual needs. With these insights, therapists can design exercises that promote recovery and adjust as necessary, based on real-time data.
- Post-Surgery Monitoring: In post-operative care, tracking motion helps evaluate recovery progress. If a patient is not moving as expected, interventions can happen sooner rather than later, enhancing recovery outcomes.
- Fall Risk Assessment: For the elderly population, understanding how someone walks can predict fall risks, leading to preventive measures that can dramatically increase safety at home.
The benefits of integrating inertial motion tracking into healthcare extend beyond mere data collection; they also enhance patient engagement and outcomes. As patients witness tangible progress through real-time feedback, their motivation to adhere to rehabilitation programs often increases.
Robotics and Autonomous Systems
Robotics is another prominent field harnessing inertial motion tracking. With autonomous systems, accurately detecting and responding to motion in real-time is crucial. For example, drones equipped with inertial measurement units (IMUs) can navigate complex terrains, compensate for unexpected movements, and maintain stability during flight. This technology is vital for ensuring both efficiency and safety in robotic operations.
- Self-Driving Cars: Vehicles utilize motion tracking to gauge their position relative to the environment, essential for navigation and collision avoidance.
- Industrial Automation: In manufacturing environments, robots can track their movements precisely, ensuring tasks like assembly or packing are completed in a timely and efficient manner.
These applications illustrate that inertial motion tracking not only improves performance but also enhances the safety of complex systems, which is coveted in both personal and commercial sectors.
Sports and Performance Analysis
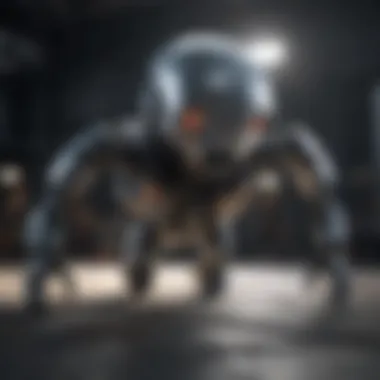
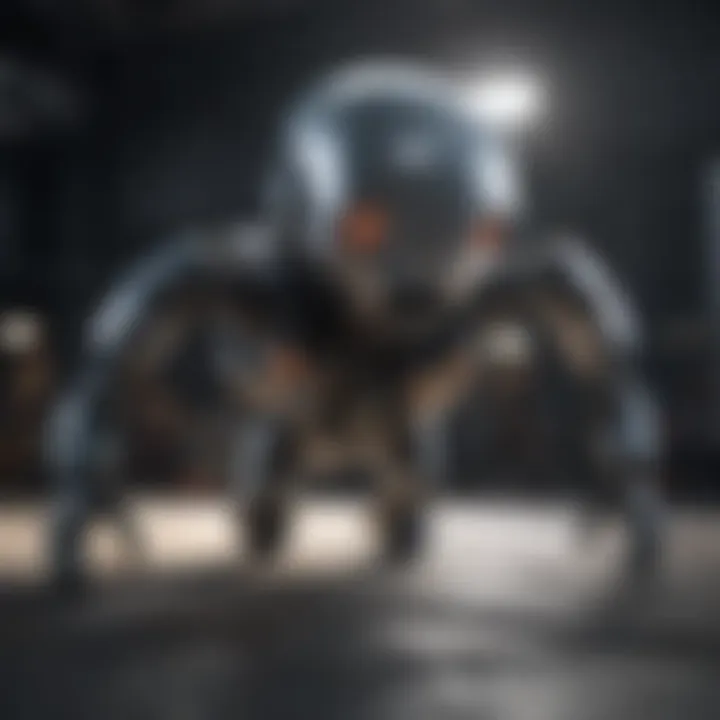
When it comes to sports, the integration of motion tracking is a game changer. Athletes and coaches use these technologies to analyze and enhance performance significantly. By employing sensors during practice sessions, athletes can receive valuable feedback that highlights areas for improvement.
Here are some specific uses:
- Biomechanical Analysis: Coaches can study an athlete’s body mechanics during crucial movements to refine techniques, potentially leading to enhanced performance and fewer injuries.
- Player Tracking: In team sports, individual motion data help coaches develop strategies that leverage each player's strengths, maximizing the team's overall performance.
Sports scientists are continually pushing the envelope to ensure that athletes are not just performing but also performing smartly, and motion tracking stands as a pillar in achieving that.
Virtual and Augmented Reality Applications
The marriage of inertial motion tracking with virtual and augmented reality creates immersive experiences that are blurring the lines between the physical and digital worlds. Users can interact within these environments in real-time, heightening engagement and realism.
Consider video games or simulators. Here, motion sensors track head and hand movements, allowing players to navigate environments intuitively. The implications extend beyond entertainment:
- Training Simulations: Medical students might conduct virtual surgeries with real-time feedback, honing their skills without risk to patients.
- Architectural Visualization: Architects can walk through their designs virtually, understanding spatial relationships and making immediate adjustments before construction begins.
The impact of inertial motion tracking in these fields showcases its ability to revolutionize how we perceive and interact with technology, merging reality with the virtual realm in unprecedented ways.
"Inertial motion tracking is more than just data collection; it's about enhancing human interaction with technology."
In summary, the application domains for inertial motion tracking are vast and varied, each presenting unique advantages that continue to evolve as technology progresses. Innovations in this field are not only improving traditional practices but are also paving the way for entirely new ways of engagement, understanding, and efficiency.
Challenges in Inertial Motion Tracking
Inertial motion tracking has transformed numerous industries by providing real-time data about movement and orientation. However, like anything that has potential, it’s not without its hurdles. Addressing these challenges is crucial for enhancing the reliability and accuracy of motion tracking systems. This section will delve into significant challenges: sensor drift and error accumulation, environmental interference, and data processing limitations.
Sensor Drift and Error Accumulation
Sensor drift is akin to a tiny pebble in the shoe of an otherwise well-crafted hiking boot. It may not seem like much at first, but over time, it can lead to significant discomfort or even halt progress entirely. In the realm of inertial motion tracking, sensor drift refers to the gradual deviation of sensor readings from the true value over time.
- Origins of Sensor Drift: This issue often arises from imperfections in the sensors used, particularly in accelerometers and gyroscopes. Environmental factors such as temperature fluctuations can exacerbate the problem, leading to additional drift which is difficult to correct.
- Impact: Without regular calibration, the accumulation of these errors can result in misleading data. Imagine a car’s GPS that constantly underestimates your speed due to sensor drift; it could put you at risk or lead to inefficient route choices.
- Mitigation Techniques: Solutions generally involve algorithms designed to compensate for drift, such as Kalman filters. Another tactic is to periodically recalibrate the sensors, though this may not always be feasible in real-time applications. The balance between maintaining accuracy and minimizing interruptions is always a critical concern.
Environmental Interference
Inertial motion tracking systems are sensitive, much like a well-tuned orchestra. They rely on precise synergy to function optimally, but various factors can create dissonance. Environmental interference poses a significant challenge by introducing noise and inaccuracies into the sensor readings.
- Electromagnetic Interference: Common sources include mobile phones and various electronic devices. Just as a buzzing fly can distract from a riveting performance, these signals can confuse the sensors, leading to erratic outputs. This interference can distort data, making it crucial to identify environments that might cause such problems.
- Physical Obstructions: Another aspect to consider is the spatial arrangement and physical conditions. Factors such as vibrations from nearby machinery, or even extreme temperatures, can impact the performance of inertial sensors.
- Solutions: Shielding sensors and integrating other types of sensors can provide a more comprehensive picture. Combining inertial data with outputs from GPS or visual tracking can significantly enhance the robustness against such environmental noise.
Data Processing Limitations
Data processing limitations often serve as the bottleneck in the workflow of inertial motion tracking systems. While the sensors gather critical data, translating that into a usable format can be a daunting task. Think of it like preparing for a big presentation: gathering information is only part of the battle.
- Real-time Processing Needs: In many applications, especially those in dynamic environments, there’s a pressing requirement for real-time analysis. However, processing high-volume data quickly enough to maintain accuracy can push current systems to their limits. Slow processing results in a lag that can render the motion tracking ineffective.
- Algorithmic Constraints: Existing algorithms that analyze the data might not adapt well to all circumstances. Some might perform brilliantly in simulations yet fail in real-world scenarios due to unexpected complexities. This can lead to poor user experience in applications like gaming or augmented reality.
- Future Directions: Continuous improvements in computational efficiency and the advent of more sophisticated algorithms could pave the way for better data handling. As machine learning continues to evolve, its integration into data analysis processes may prove pivotal for overcoming existing limitations.
"In addressing these challenges, the robustness and reliability of inertial motion tracking systems can improve significantly, ushering in more advanced applications and user experiences throughout diverse fields."
Advancements and Future Directions
Inertial motion tracking is at a pivotal point, with technological innovations paving the way for future enhancements. This section will explore significant advances that promise to reshape how motion tracking operates across different sectors. By focusing on elements such as the integration of machine learning, miniaturization of sensor technologies, and improved user interfaces, we can begin to understand their profound impacts on the industry and the potential benefits they bring.
Integration of Machine Learning
Machine learning is revolutionizing how data from inertial motion tracking is analyzed and interpreted. Traditionally, understanding motion data relied heavily on algorithms that may not always adapt well to real-world complexities. However, by integrating machine learning, systems can now learn from vast amounts of data, identifying patterns and anomalies that humans might miss.
For instance, in healthcare applications, machine learning can accurately differentiate between normal and abnormal movement patterns in patients. This can lead to personalized rehabilitation programs that adapt to the patient’s specific needs, ultimately improving recovery times and outcomes. Moreover, in robotics, these advanced algorithms enable robots to predict their next movements based on previous data, leading to smoother and more efficient navigation.
Miniaturization of Sensor Technologies
The trend of miniaturizing sensor technologies, such as gyroscopes and accelerometers, is not just about making devices smaller; it's about making them smarter and more versatile. As sensors become smaller, they can be integrated into a wider array of devices without compromising performance.
For example, consider smartwatches and wearable fitness trackers. The reduced size of sensors allows them to be more discreet and comfortable while still providing accurate data for motion tracking. This miniaturization leads to a greater adoption of these technologies in everyday life and supports a shift towards more ubiquitous forms of tracking, which can benefit sectors like sports performance analysis and personal health monitoring.
Enhanced User Interfaces
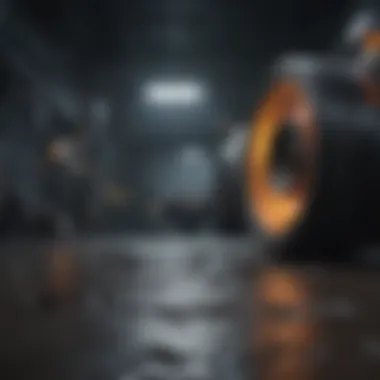
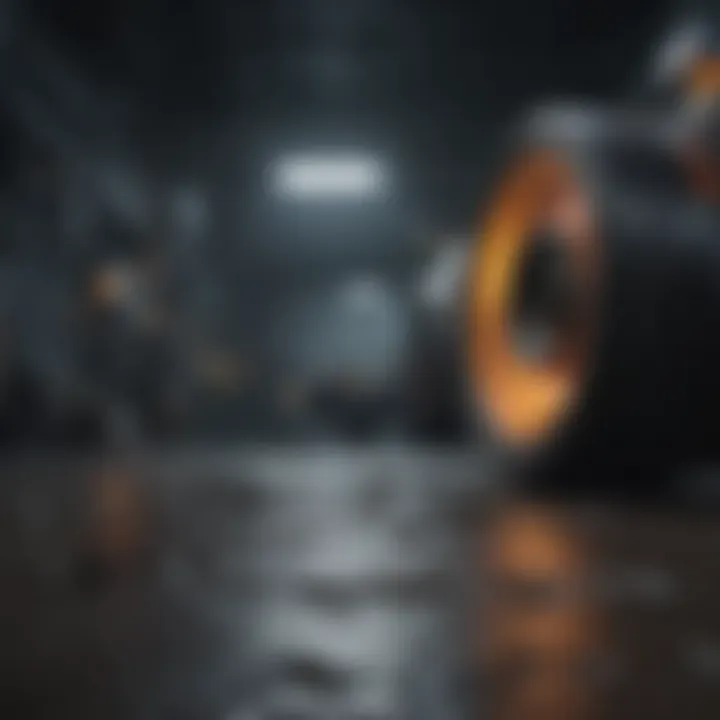
A crucial aspect of pushing inertial motion tracking into the mainstream lies in developing enhanced user interfaces. It goes beyond just making applications look good; it’s about creating intuitive systems that allow users to interact seamlessly with motion data.
The introduction of touch screens, voice control, and augmented reality applications has changed the way users engage with motion tracking technologies. Simplified interfaces make it easier for professionals and amateurs alike to access and understand complex data without needing extensive technical backgrounds.
"The future of motion tracking technologies hinges on how accessible and user-friendly we can make them."
In summary, these advancements not only enhance the effectiveness of inertial motion tracking but also expand its applications across various fields. As machine learning continues to evolve, sensors shrink, and interfaces improve, the potential for innovation appears limitless, setting the stage for exciting developments in the near future.
Ethical Considerations
The intersection of technology and ethics tends to be quite a fertile ground, especially in fields like inertial motion tracking. As these technologies proliferate, the discussion around ethical considerations becomes not just important, but essential. Understanding the ramifications of employing such technologies is vital for ensuring that innovation does not come at the cost of personal freedoms or integrity.
One of the primary ethical concerns is the potential infringement on personal privacy. With more devices equipped to track motion, data collection has significantly escalated. That data could be sensitive, revealing patterns of everyday behaviors, or even indicating health conditions. This raises the question—who has access to this information, and what are they doing with it?
"Ethics in technology is not just a side note; it’s the bedrock upon which trust is built."
As technology evolves, regulations struggle to keep pace. In a society driven by data, individuals may inadvertently find themselves in a situation where their movements are monitored without their explicit consent. This highlights the necessity for robust guidelines around privacy. Grainy privacy policies that few read leave room for interpretations that serve corporate interests more than those of the individual.
Privacy Issues in Motion Tracking
When we delve into privacy issues surrounding motion tracking technologies, several specifics come to light. For one, user consent is crucial. It’s one thing to have technology that tracks how you move but quite another to have that data sold or misused. Clear norms regarding informed consent are necessary. Users should be fully aware of what data is being collected, the purpose behind it, and, most importantly, who will have access to it.
Key considerations include:
- Transparency: Companies ought to be crystal clear about their data collection methods.
- User Control: Individuals should have the power to manage their own data, including opting out whenever possible.
- Anonymization: It’s not just about collecting data; it’s also about using methods that anonymize user data to protect identities.
Data Security Implications
Data security presents another layer of ethical considerations. As collection methods improve, so do the methods for potential unauthorized access to that data. An unsecured motion tracking system becomes an open door for hackers, exposing sensitive information. If motion tracking data ends up in the wrong hands, the repercussions can be severe, ranging from identity theft to more invasive forms of harassment.
For organizations involved in motion tracking, setting up stringent security measures is non-negotiable. Protecting user data involves more than just ground-level encryption; it calls for a multi-layered approach that includes:
- Regular audits to assess security vulnerabilities.
- Employee training on best practices for maintaining data integrity.
- Two-factor authentication for accounts that handle sensitive data.
In light of these ethical considerations, it’s clear that while inertial motion tracking offers remarkable benefits, it carries responsibilities that cannot be overlooked. As society continues to embrace these technologies, a proactive stance toward ethical practices is imperative to ensure a balanced and fair integration into our daily lives.
Case Studies
Examining case studies in inertial motion tracking provides invaluable insights into how the theories and technologies discussed earlier manifest in real-world scenarios. Case studies showcase specific implementations that clarify the practical benefits, challenges, and the broader impact of inertial motion tracking across various fields. By diving into concrete examples, we can grasp the diverse applications and the lessons learned from both successes and setbacks. This not only aids in understanding the current landscape but also highlights future directions for research and technology development in this domain.
Successful Implementations in Healthcare
In healthcare, inertial motion tracking is revolutionizing patient monitoring and rehabilitation practices. One notable example is its application in post-surgical recovery, where hospitals have begun using wearable inertial measurement units on patients. These devices track movements, helping clinicians analyze recovery patterns and identify any potential complications early on.
Consider a study conducted in a rehabilitation center that utilized IMUs to monitor the gait of stroke patients. By collecting data on their walking patterns, therapists could tailor individualized rehabilitation programs. The insights gained allowed for more adaptive therapy sessions, ultimately resulting in improved mobility scores among participants. This success story highlights how motion tracking can enhance recovery outcomes, emphasizing the need for more widespread adoption in the healthcare sector.
Another significant case involves the use of motion tracking technology in physical therapy. A system developed for assessing balance and stability in elderly patients has been receiving attention. The device integrates accelerometers and gyroscopes to provide real-time feedback. This feedback allows therapists to adjust interventions dynamically, increasing the effectiveness of treatments and empowering patients to engage actively in their own recovery.
Innovations in Robotic Motion Tracking
The field of robotics has also seen a surge in innovative applications of inertial motion tracking. One prominent example can be found in autonomous drones, which utilize advanced sensor fusion algorithms to create precise navigation systems. A specific case is the use of inertial measurement units combined with global positioning systems (GPS) in delivery drones. The constant updates from these sensors help maintain stability and navigational accuracy, addressing the challenges posed by abrupt maneuvers and environmental disturbances.
Moreover, researchers at a leading robotics lab have experimented with tracking technology for robotic arms used in manufacturing. By embedding gyroscopes and accelerometers within the robot's framework, they achieved significant improvements in motion accuracy. This advancement has led to more efficient automation processes, as the robotic arms can adapt their actions based on real-time data regarding position and velocity. The findings indicate that such systems not only enhance the precision of tasks but also reduce wear and tear on machinery, prolonging the lifespan of equipment.
These case studies underscore the transformative potential of inertial motion tracking. Both in healthcare and robotics, the applications demonstrate the significant advantages that arise from tracking motion accurately. The benefits are not merely technical; they touch upon the essence of improved human experiences and efficiency.
Summary and The End
The exploration of inertial motion tracking within this article serves as a compass, guiding readers through its myriad principles and real-world applications. This section stands as a pivotal reflection on the significance of understanding inertial motion tracking, encapsulating the vital points discussed throughout the article while highlighting its benefits and considerations.
Inertial motion tracking is not just a technical concept but a powerful tool that bridges various fields like healthcare, robotics, and sports. The capabilities of this technology offer unique insights and improvements across these sectors. For instance, in healthcare, the ability to continuously monitor a patient’s motion allows for personalized rehabilitation programs tailored to individual recovery needs. Likewise, in the sphere of robotics, enhancing motion precision can lead to more efficient operations and innovations in automation.
Additionally, the challenges surrounding inertial motion tracking cannot be overlooked. Factors like sensor drift and environmental interferences pose significant hurdles that require ongoing research and adaptive solutions. This discussion is crucial, as it encourages stakeholders to remain cognizant of these issues while fostering innovation within the field.
Understanding inertial motion tracking not only unveils its operational mechanics but also highlights its transformative potential across various domains.
Recap of Key Points
- Defining Inertial Motion Tracking: Inertial motion tracking provides methods to understand and monitor physical motion using technologies such as gyroscopes and accelerometers.
- Fundamental Principles: It’s deeply rooted in Newtonian mechanics, where inertia plays a crucial role in determining motion behaviors.
- Key Technologies: With components like Inertial Measurement Units (IMUs) and respective sensors, the precision of tracking is vastly improved through fusion algorithms that interpret data effectively.
- Application Domains: Various industries utilize this technology, notably in healthcare for treatment monitoring, in robotics for improved functionality, and in sports for performance analysis.
- Challenges: The field grapples with sensor drift, environmental influences, and limits in data processing, all of which must be addressed to ensure accuracy and reliability.
- Advancements: Future prospects include machine learning integration, sensor miniaturization, and improved user interfaces, all contributing to an enhanced tracking experience.
- Ethical Considerations: Discussions around privacy and data security are essential to mitigate risks associated with widespread motion tracking technology.
In summary, this article underscores the importance of inertial motion tracking, paving the way for deeper understanding and future innovation. It not only highlights current applications but sets the stage for addressing ongoing challenges, allowing for further exploration and adoption of this transformative technology.