Comprehensive Insights into Ellipsometer Thickness
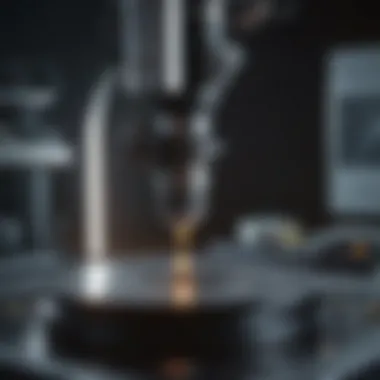
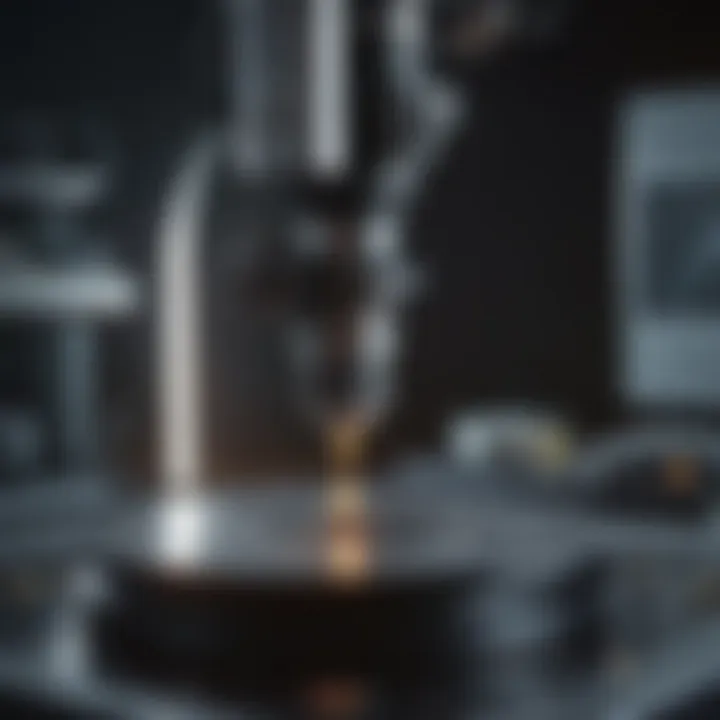
Intro
In the world of material science and optics, understanding the thickness of thin films can feel like trying to find a needle in a haystack. Yet, ellipsometry shines a light on this challenge, providing precision that is hard to beat. This article aims to demystify the concept of ellipsometer thickness, focusing on its relevance in various scientific fields. A deep dive into its principles, measurement techniques, and technological advancements lay the groundwork for understanding how ellipsometry transforms our approach to material characterization.
Research Overview
Summary of Key Findings
Throughout this examination, we uncover several critical insights:
- Operating Principles: Ellipsometers function by analyzing the change in polarization state of light reflected from a surface, granting a unique perspective on thin film thickness.
- Wide-ranging Applications: From semiconductor manufacturing to biological research, the applications of ellipsometry span many disciplines.
- Measurement Challenges: Various factors, such as surface roughness and optical inhomogeneities, can complicate thickness measurements, necessitating advanced solutions.
Background and Context
To appreciate ellipsometry's role, one must understand the context in which it was developed. Originating in the 19th century, ellipsometry has evolved significantly, driven by advances in technology and materials science. Early implementations were rudimentary, but as optical components and lasers became more refined, ellipsometric methods adapted. Now, this technique enjoys a reputable standing in academic and industrial settings alike. Its accuracy and adaptability enable it to meet the rigorous demands of scientific inquiry.
Methodology
Experimental Design
When conducting ellipsometric measurements, the setup should be meticulously planned. The configuration generally includes:
- A monochromatic light source, often a laser, which illuminates the sample.
- A polarizer that prepares the light’s initial polarization state.
- A device to detect the reflected light and analyze its polarization change.
The results can then be correlated to the film’s thickness using mathematical models and curves that interpret the data from the experiment.
Data Collection Techniques
The collection of accurate data is paramount. Different techniques may be employed depending on the setup:
- Parameter Extraction: Utilizing software tools to extract the necessary parameters from the detected polarization changes.
- Calibration: Regular calibrating procedures are essential to ensure the equipment's accuracy, influencing the reliability of thickness measurements.
- Repetition: Performing multiple measurements helps account for variations and enhances the robustness of the results.
"In ellipsometry, the nuances of light's interaction with materials reveal a universe of information about thickness and surface properties."
The above encapsulates the essence of what makes ellipsometry pertinent in understanding thin films. As we progress through the article, you’ll discover deeper complexities and further nuances behind thickness measurement techniques, their applications, and the innovations reshaping how we think of this crucial parameter.
Preamble to Ellipsometry
Ellipsometry serves as a gateway into understanding not just the properties of thin films, but the interactions of light with materials at a microscopic scale. This technique doesn’t merely scratch the surface; it dives into the very essence of how light behaves when it meets various substrates, offering unparalleled insights in fields ranging from material sciences to bioengineering. In a world where precision is paramount, grasping the fundamentals of ellipsometry becomes not just beneficial, but essential for researchers and professionals alike.
What makes ellipsometry particularly intriguing is its dependence on polarized light. Unlike traditional measurement methods, which might rely on direct thickness readings or simple reflectance, ellipsometry analyzes changes in light's polarization upon reflection from a surface. This subtle shift can indicate variations in film thickness, composition, and even surface roughness. Understanding this method is a crucial building block for anyone looking to delve deeper into material characterization.
Ellipsometry embodies a marriage of sophisticated optics and practical application, revealing properties that are often invisible to the naked eye.
In addition to its fascinating operational principles, the historical evolution of ellipsometry offers context to its significance within contemporary research. Tracing back to the initial experiments that led to its adoption, one can appreciate how far the technique has come. The blend of innovation and application that embodied its development underscores its relevance today and provides a rich backdrop against which current advancements can be measured.
In summary, the importance of ellipsometry in scientific exploration cannot be understated. Its ability to provide in-depth information about thin films and surface interactions positions it as a cornerstone tool for researchers across various disciplines. As we unravel the subsequent sections, we will delve further into its definition, historical background, and the physics governing this essential technique.
The Physics Behind Ellipsometry
In the field of optical measurement, understanding the physics behind ellipsometry emerges as a cornerstone for accurately interpreting thin film properties. This section elucidates the intricate principles that govern the operation of ellipsometers. By grasping these fundamentals, one can appreciate how variations in light behavior become pivotal in determining film thickness and optical constants.
Understanding Optical Properties
Optical properties are at the heart of ellipsometry. The relationship between light and matter dictates how films reflect and transmit light. When light strikes a surface, it can be either reflected or transmitted. The degree to which this occurs is influenced by the material's refractive index, absorption coefficient, and thickness.
For thin films, especially those less than 1 micron thick, these properties interplay in complex ways. Polarization, for instance, plays a crucial role. Unpolarized light can be represented as a combination of two perpendicular wave components. When this light interacts with a thin film, the two components undergo different phases of reflection. By analyzing the resultant polarized light, one can extract vital information about the film’s physical characteristics.
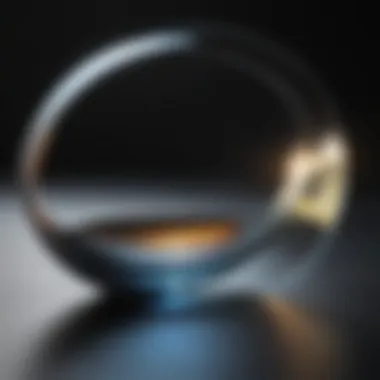
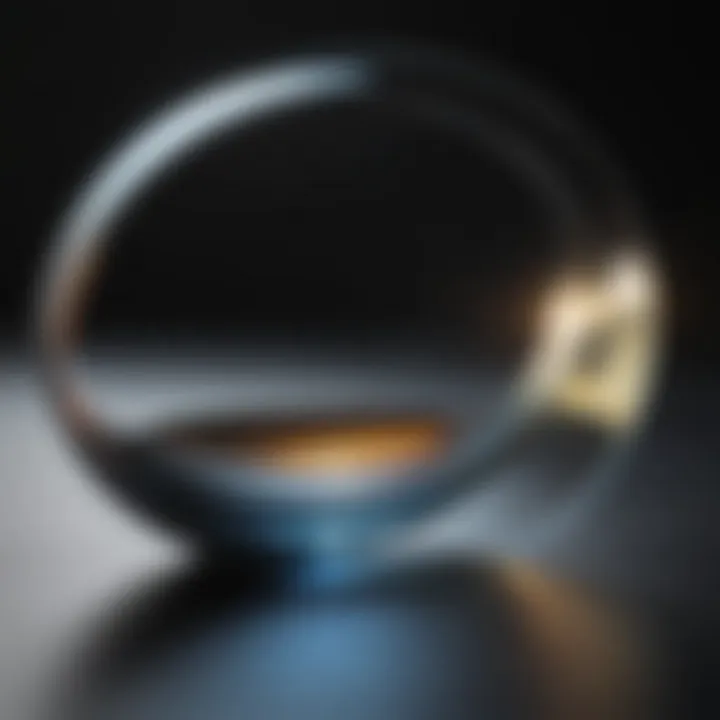
An insightful aspect to consider here is that the ellipsometric parameters—psi (Ψ) and delta (∆)—represent the amplitude ratio and phase difference of the reflected light. These measurements allow scientists to establish a clearer picture of the thin film's structure, layer composition, and even the material's uniformity.
Fundamentals of Light Interaction
Light interaction with materials isn't just a matter of hitting a surface and bouncing back. It entails a series of phenomena, including reflection, refraction, diffraction, and interference. Each of these plays its own part in the broader ellipsometric analysis.
- Reflection occurs when light encounters a boundary between air and a film. The extent of this reflection is dictated by the film's optical constants and the angle of incidence.
- Refraction deals with the bending of light as it passes from one medium to another. This bending is essential for understanding how light behaves within the film. If the refractive index of the thin film is lower than that of the substrate, light is partially refracted into the substrate. A clear grasp of Snell's law helps in determining this behavior.
- Diffraction affects how light disperses after striking a surface. For thin films, the spacing of the layers can lead to diffractive effects, which further complicates measurements. Understanding these patterns is crucial in troubleshooting measurement errors.
- Interference becomes significant as multiple layers are involved. The coherent superposition of light waves can lead to constructive or destructive interference, thereby providing insights into layer thickness and uniformity.
To summarize, a solid understanding of these interactions lays the groundwork for effective ellipsometric techniques. By disentangling these optical behaviors, one equips themselves with the knowledge necessary to apply ellipsometric measurements accurately and with confidence.
"Understanding the physics behind ellipsometry is not just about knowing the facts—it’s about connecting those facts to the real-world applications that shape our scientific landscape."
In essence, the analysis of optical properties and light interaction forms the bedrock of ellipsometric measurements. As we progress in this detailed narrative, we will delve deeper into how these principles translate into practical measurement techniques, thereby enhancing our understanding of this vital technology.
Operational Principles of Ellipsometers
The operational principles of ellipsometers are the backbone of this sophisticated optical measurement technique. Understanding these principles is vital, not just for grasping how ellipsometers work, but also for comprehending their value in various scientific applications. This section dives into three key areas: modulation of light waves, measurement techniques, and data analysis methods that unveil the effectiveness and validity of ellipsometry in thickness measurement and material characterization.
Modulation of Light Waves
At the heart of ellipsometry lies the modulation of light waves. This involves tweaking light's direction and polarization before it interacts with the sample. By using specific light sources, typically lasers, the characteristics of this light—like wavelength and intensity—become crucial. In particular, the polarization states, such as linear or circular, influence how light behaves when it strikes a surface.
To break it down:
- Light Source Selection: The choice of laser wavelength can target certain transitions in materials, improving the measurement's precision.
- Polarization Control: Progressive modulation might involve changing not only the amplitude but also the phase of the light. A common practice is the use of polarizers and phase shifters to establish different polarization states.
- Reflectance: When polarized light reflects off a thin film, it undergoes changes in amplitude and phase that are monitored, yielding information about the film properties.
Overall, manipulating light in such precise ways enhances the ellipsometer's ability to discern minute thickness variations, making it a powerful tool in many scientific arenas.
Measurement Techniques
Measurement techniques in ellipsometry are systematic approaches that combine optics with sophisticated data interpretation. Two commonly employed methods are:
- Spectroscopic Ellipsometry: This method involves measuring how the polarization state of light changes across different wavelengths. By collecting data over a broad spectrum, researchers can infer properties of materials over a range of thicknesses and compositions.
- Time-Resolved Ellipsometry: This versatile technique observes changes over time, providing insights into dynamic processes, such as film growth or phase transitions. This is particularly useful for studying materials that change state rapidly.
With these techniques in play, ellipsometry stands out in accuracy and adaptability. The ability to precisely measure thin films, even when layered or coated, positions it as a preferred method in both research labs and industrial settings.
Data Analysis Methods
Once the measurement data is collected, the next important step is analyzing it effectively. Data analysis methods in ellipsometry are diverse, employing mathematical models to interpret the complex interactions between light and material surfaces. Techniques include:
- Modeling and Simulation: Mathematical models simulate the ellipsometric data, enabling comparison between observed and theoretical results. These models often consider factors like refractive index dispersion or film thickness.
- Nonlinear Curve Fitting: This is a statistical method where the collected data is fit to theoretical curves, allowing the extraction of key parameters like thickness and optical constants.
- Principal Component Analysis (PCA): PCA can be used to reduce data complexity, helping to isolate relevant features from experimental noise. This technique finds its place in high-dimensional datasets typical in modern ellipsometry.
As a final thought, investing time to understand these core operational aspects can pay dividends in the forms of enhanced experimental designs and better data interpretations.
Ellipsometer Thickness Measurement
Understanding ellipsometer thickness measurement is pivotal when it comes to the realm of thin films. This topic not only brings insights into better characterizations of various materials but also unveils the fundamental principles that govern how measurement occurs. Thin films play a vital role in various applications, including electronics, optics, and coatings. Therefore, having precise thickness measurements can significantly influence performance and effectiveness.
To fully grasp this significance, one must consider that the accuracy of measurements ensures the reliability of data we depend on in research and industrial settings. Accurate thickness measurements are crucial for applications such as layer depositions in semiconductor devices and optical coatings where precision can dictate the success or failure of a product.
Moreover, the measurement of thin film thickness allows scientists and engineers to engage with finer details. For instance, they can monitor changes over time, understand the growth processes, and validate theoretical models. More than simply providing numerical values, effective ellipsometry can lead to the optimization of processes and products, making it an invaluable tool in the hands of those who harness its potential.
Measuring Thin Film Thickness
When it comes to measuring thin film thickness, ellipsometry stands out as a powerful technique. The method hinges on analyzing the change in polarization of light as it reflects off a thin film. This light interaction effectively encodes information about the optical properties of the material, enabling the calculation of film thickness down to nanometer levels.
In practical terms, using ellipsometry for measuring thin film thickness involves the following steps:
- Light Source: A coherent light source, usually a laser, is directed onto the surface of the film.
- Polarization Control: The light beam is polarized before entering the sample. The two main types of polarization used in ellipsometry are linear and circular polarization.
- Reflection Measurement: When the polarized light hits the film, it reflects back, and a detector measures the change in polarization state. By interpreting these changes, the thickness can be deduced.
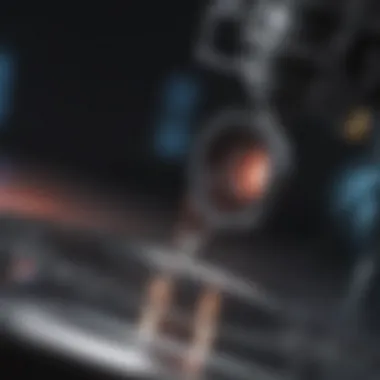
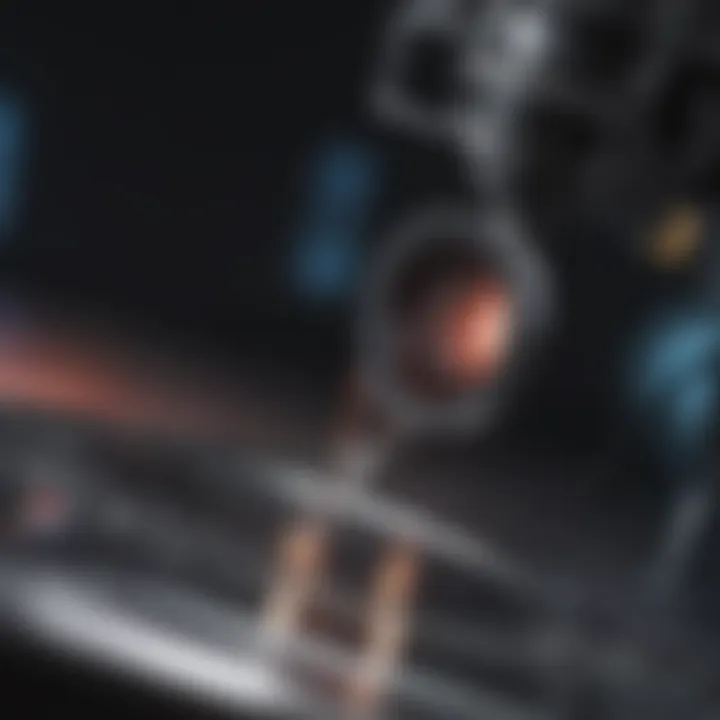
One of the primary benefits of this method is its non-destructive nature, allowing films to be analyzed without any physical contact. This measure of precision and minimal interference greatly advantages industries where even the slightest alteration can lead to significant performance issues.
Role of Polarization
Polarization plays a fundamental role in ellipsometric measurements. It acts as the key that unlocks the secrets held within the thin films. When light undergoes reflection, its state of polarization changes. Understanding these alterations is essential for extracting accurate information about thickness and optical properties.
The two primary types of polarization relevant in this context are:
- Linear Polarization: Here, the light oscillates in one direction. Analyzing light that is linearly polarized enables researchers to gain insights into the anisotropic properties of materials.
- Circular Polarization: This involves light that rotates as it propagates. It can be beneficial when examining complex multi-layer structures and helps in identifying subtle variations in material properties.
Understanding how to manipulate these polarization states allows researchers to enhance their measurements, improve accuracy, and broaden the applicability in various fields. Overall, the role of polarization in ellipsometry can't be overstated; it is the cornerstone upon which effective measurements rest.
Applications of Ellipsometry in Science
Ellipsometry serves as a powerful optical measurement technique, significantly enhancing our understanding in a range of scientific fields. Its ability to provide precise information about film thickness and optical properties makes it indispensable in material characterization. The applications of ellipsometry extend beyond simple measurement; they encompass intricate analyses that pave the way for groundbreaking discoveries and innovations. Some key elements of its application include versatility, sensitivity, and the ability to gather data non-destructively. In this section, we'll delve into specific applications of ellipsometry, covering three principal sectors where its influence is most significantly felt.
Use in Material Science
In material science, ellipsometry stands out as a critical tool for studying properties of thin films. It facilitates the advanced characterization of various materials, enabling researchers to assess everything from the fundamental properties of crystalline structures to the surface conditions of polymers. The technique leverages the fundamental physics of light interaction with materials, allowing for discernible differences in coatings, substrates, and more to be meticulously analyzed.
"Ellipsometry can distinguish between subtle layers of material, which plays a crucial role in the development of multi-layer coatings used in optics and photonics."
Typically, ellipsometry is employed to determine refractive indices and dielectric constants, which are pivotal for understanding material behavior. Accurate thickness measurements help refine processes such as thin-film deposition and engineering of surface properties, thus making it a cornerstone in material development. Researchers can modify parameters like composition and environmental conditions and monitor changes in real-time.
Application in Semiconductor Industry
The semiconductor industry greatly relies on ellipsometry, particularly during the fabrication of electronic devices. Here, precision is paramount. Any defects or inconsistencies can lead to substantial losses in yield or functionality. Ellipsometers are utilized to monitor thin film growth in processes such as chemical vapor deposition and molecular beam epitaxy.
With the push towards smaller, more efficient circuits, ellipsometry enables better control over layer uniformity and composition. Its non-invasive nature ensures that the underlying substrate remains unharmed during measurements, allowing for multiple evaluations over the same sample without degrading material quality. In addition, integrated ellipsometric feedback systems assist in real-time adjustments during manufacturing, greatly enhancing the production workflow.
Role in Biological Studies
The field of biology has embraced ellipsometry as well, particularly in the study of biomaterials and cellular structures. By measuring layer thicknesses at the nanometer scale, researchers can investigate how cells interact with their environment. This application is crucial in fields such as tissue engineering and regenerative medicine, where understanding cell behavior is essential for designing effective therapies.
Ellipsometry provides insights into molecular layer formation and cell adhesion without causing damage, making it invaluable for assessing biocompatibility of materials used in implants and prosthetics. It aids in tracking the effects of drugs on cellular membranes, leading to better understanding of drug delivery mechanisms.
In summary, ellipsometry's broad applicability across material science, semiconductors, and biology showcases its vital role in advancing scientific knowledge. As technology evolves, we can only expect this tool to become even more integral in diverse applications.
Challenges in Ellipsometric Measurements
Measuring thin film thickness is no easy feat. Ellipsometry, while powerful, is not without its challenges. Understanding these challenges is crucial for both the accuracy and the reliability of ellipsometric measurements. Issues can arise from systematic errors and the need for precise instrument calibration, both of which can significantly affect outcomes. Addressing these challenges ultimately enhances measurement fidelity, leading to better science and technology applications.
Systematic Errors
Systematic errors in ellipsometric measurements stem from consistent, repeatable inaccuracies that affect all observations under similar conditions. These errors can be caused by various factors, and recognizing them is essential to correcting the data collected. A few notable sources of systematic errors include:
- Optical Misalignment: Alignment of the optics must be spot on. Any misalignment can lead to skewed results.
- Inconsistent Light Source: Variability in the light source can also introduce errors. This includes changes in intensity or wavelength that might go undetected.
- Environmental Factors: Changes in temperature or atmospheric pressure can alter the properties of the samples being measured, leading to inconsistencies in data collection.
"A tiny misalignment in the optical path can lead down a rabbit hole of incorrect measurements."
To mitigate systematic errors, it is advisable to carry out regular checks and calibrations of the equipment. Establishing a baseline measurement with known standards can also help to identify potential deviations in data collection. Proper documentation of the environmental conditions during measurement helps ensure that external factors are taken into account.
Instrument Calibration Issues
Calibration of the ellipsometer itself is a step often described as 'boring' but is, in fact, critically important. An incorrectly calibrated instrument can yield measurements that misrepresent the true properties of the material being analyzed. Several issues may arise regarding calibration:
- Drift Over Time: Instruments can experience drift, where calibration changes slowly over time as components wear or as environmental conditions change.
- Calibration Standards: Using the wrong calibration standards can mislead the results. It is crucial to use standards whose properties are well-defined and known.
- Regular Maintenance: Failing to maintain the instrument can result in deviations, making regular maintenance a must.
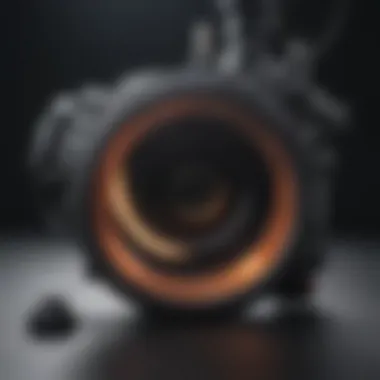
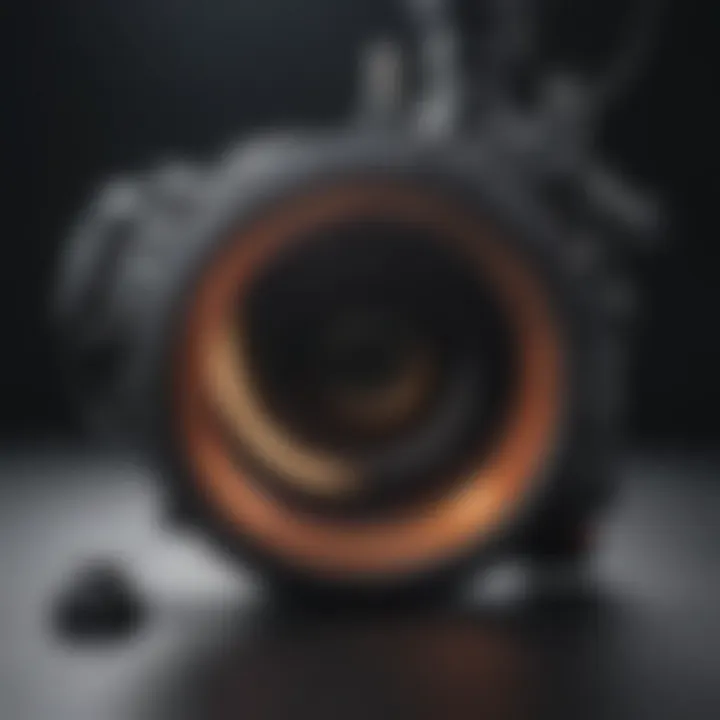
To ensure optimal performance, it is often recommended to conduct calibration checks frequently, especially if the instrument is in constant use. This includes running checks with standard samples before and after significant measurement sessions.
Advancements in Ellipsometry Technology
In understanding ellipsometer thickness, it is vital to examine how advancements in the technology have enhanced measurement capabilities. These developments not only improve the accuracy of thickness measurements but also expand the applicability of ellipsometers in various fields. As the demand for precision in measuring thin films grows, technologies that foster these improvements are becoming increasingly significant. The integration of advanced components, better algorithms, and cutting-edge methodologies allows for an evolved approach in ellipsometry, making it indispensable in both research and industrial contexts.
Enhancements in Measurement Precision
The precision of measurements in ellipsometry plays a crucial role in applications ranging from semiconductor manufacturing to material characterization. With the advent of new optical components, such as high-quality lasers and advanced detectors, measurement precision has reached new heights. These enhancements include:
- Improved Light Sources: Modern lasers provide stable and coherent light, which mitigates noise and enhances the signal quality in measurements.
- High-Resolution Detectors: Contemporary detectors offer a better dynamic range and response time, allowing for capturing subtle changes in polarization states with great accuracy.
- Advanced Modulation Techniques: Techniques such as phase modulation and spectroscopic ellipsometry enable more sophisticated interpretations of light-material interactions, resulting in higher precision in thickness assessments.
Each of these factors contributes to a more reliable measurement, essentially minimizing the errors that can occur in thin film studies.
Integration with Computational Techniques
The integration of computational techniques in ellipsometry marks a significant stride toward optimizing the processes involved in thickness measurement. Computational models and simulation tools aid in data interpretation and analysis, facilitating clearer insights into the materials being studied. This integration encompasses:
- Machine Learning Algorithms: Using machine learning to analyze ellipsometric data helps in identifying patterns that might not be apparent through traditional methods. These algorithms can predict surface properties and behavior based on extensive databases of material characteristics.
- Numerical Modeling: Techniques like finite element modeling allow for accurate simulations of light interaction with complex materials, better predicting outcomes and validating experimental results.
- Automated Data Processing: Automation in data analysis streamlines the workflow, reducing manual input errors and significantly expediting the process of obtaining detailed readings from complex measurements.
By embracing these computational advancements, researchers are not just enhancing ellipsometric measurements; they are revolutionizing the way we approach material science across various disciplines.
The fusion of state-of-the-art technology with robust computational methods emphasizes a pivotal moment for ellipsometry, situating it firmly at the forefront of advanced material studies and applications. As this field continues to evolve, the potential uses for ellipsometry within diverse sectors only seem to broaden, promising intriguing avenues for exploration and innovation.
Future Directions in Ellipsometry Research
The field of ellipsometry is evolving at a rapid pace, driven by technological advancements and the increasing demand for precise measurements in various applications. Looking ahead, the future of ellipsometry research is brighter than ever, characterized by innovative techniques and promising applications. Understanding these future directions not only enhances the existing body of knowledge but also lays the groundwork for improved methodologies and broader applicability of ellipsometers.
Emerging Techniques
The landscape of ellipsometry is being transformed with the advent of emerging techniques that promise to enhance measurement capabilities. One such technique gaining traction is spectroscopic ellipsometry, which involves measuring the complete spectrum of reflected light rather than just its polarization state. This method provides richer data and increases the sensitivity of thin film characterization, making it invaluable in research and manufacturing settings.
Another area of interest is the development of in situ ellipsometry. This approach enables real-time monitoring of thin films during fabrication processes. By integrating ellipsometry with manufacturing systems, researchers can gain instantaneous insights into film growth dynamics and other material properties, thereby optimizing production and ensuring quality control.
"Innovation in measurement techniques is essential to keep pace with the evolving materials science landscape."
Moreover, machine learning algorithms are being incorporated into ellipsometric data analysis. This fusion of AI with traditional measurement methods can facilitate a more nuanced understanding of complex data sets, dramatically reducing analysis time and increasing accuracy. As industries push for faster and more reliable results, such advancements will be crucial.
Potential Applications in Novel Materials
As we embark on the journey of exploring future possibilities, the application of ellipsometry in the realm of novel materials stands out as particularly exciting. The rise of 2D materials, such as graphene and transition metal dichalcogenides, presents a fertile ground for high-precision measurement techniques. Ellipsometry offers a non-destructive method to probe these materials, revealing their optical properties and thickness, which are essential for integrating them into practical applications.
Another promising domain is the exploration of quantum dot technology. These nanomaterials have unique optical properties that can be exploited in various applications, including displays, sensing, and solar cells. By leveraging advanced ellipsometric techniques, researchers can investigate the complex interactions between light and matter at the nanoscale, paving the way for innovative uses in optoelectronics and photonics.
Furthermore, organic photovoltaics and thin-film solar cells are increasingly gaining attention due to their potential for a more sustainable energy future. Ellipsometry can play a pivotal role in optimizing the design and fabrication of these photovoltaic devices, enhancing their efficiency and stability.
The integration of ellipsometric techniques with novel materials not only spurs advancements in fundamental research but also contributes to the development of groundbreaking applications across multiple sectors. The fusion of cutting-edge technology with the meticulous measurement capabilities of ellipsometry positions researchers to make significant strides in material science, energy, and beyond.
Closure
The topic of ellipsometer thickness holds a pivotal role in understanding optical measurement techniques and their applications in various scientific fields. From material science to semiconductor technology and beyond, accurately measuring thin film thickness is crucial, and this is where ellipsometry shines. This closing section aims to encapsulate the key findings discussed throughout the article, highlighting the significance and the complexities involved in achieving precise measurements using ellipsometers.
Summarizing Key Findings
In summation, the exploration of ellipsometer thickness uncovers a wealth of knowledge:
- Importance of Thin Film Measurement: The ability to measure thin films with great accuracy allows researchers and industry professionals to optimize processes and develop advanced materials. The thin film's properties can significantly affect the overall performance of devices in electronics, optics, and material science.
- Operational Principles: Understanding the underlying principles of how ellipsometers operate is essential. The interaction of light with materials, the role of polarization, and measurement techniques utilized are all components that contribute to accurate thickness assessments. Each of these elements works in concert to provide reliable data that can influence the direction of research and development.
- Current Challenges: The article sheds light on various challenges faced in ellipsometric measurements, such as systematic errors and instrument calibration issues. Recognizing these pitfalls is the first step towards refining techniques and improving measurement reliability.
- Technological Advancements: Recent advancements in ellipsometry technology have opened new avenues for achieving higher precision and integrating these tools with computational methods. This progress underscores how essential continual development is in the pursuit of accuracy that meets modern demands.
"Understanding the intricacies of ellipsometric techniques can make a world of difference in scientific research and development."
Final Thoughts on Ellipsometer Thickness
To wrap up this comprehensive overview, it's clear that grasping the nuances of ellipsometer thickness is not just an academic exercise, but a foundational element in numerous fields. For students and professionals alike, engaging with this topic offers a deeper appreciation for how optical properties are connected to material characteristics. As research propels forward into new materials and applications, the role of ellipsometry will only rise in importance.
As we venture into an era where precision and innovation are paramount, understanding and leveraging the capabilities of ellipsometers can furnish professionals with competitive advantages, ultimately pushing the boundaries of what's possible in science and technology.