Measuring Reactive Oxygen Species in Cellular Environments

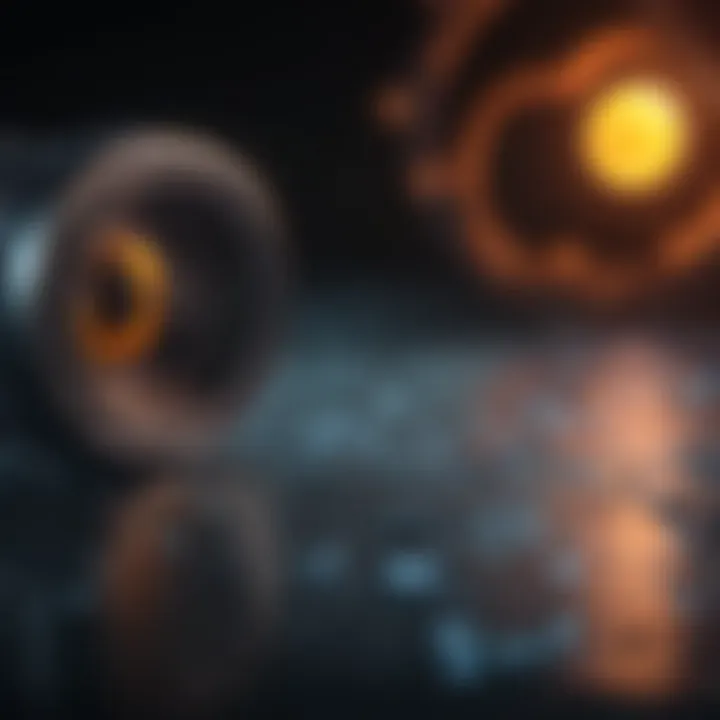
Intro
Reactive oxygen species (ROS) play critical roles in various cellular processes. Understanding how to measure ROS is vital for researchers. This area of study shines light on cellular responses to oxidative stress and helps elucidate its implications for health and disease.
The significance of ROS extends to both normal physiological functions and pathological conditions. For example, ROS are involved in cell signaling and homeostasis. However, an overproduction of these species can lead to oxidative damage, impacting DNA, proteins, and lipids. Such damage is linked to many diseases, including cancer and neurodegenerative disorders. Therefore, precisely measuring ROS levels is essential for understanding their role in these conditions.
Research Overview
Summary of Key Findings
Surveying the existing literature reveals several key findings regarding ROS measurement. Early methods primarily relied on chemiluminescent and spectrophotometric techniques. More recent innovations have introduced advanced methods such as
- Fluorescence microscopy
- Electrochemical sensors
These methods provide enhanced sensitivity and specificity, allowing for more accurate quantifications of ROS in a variety of cell types.
> Advances in measurement techniques have transformed our understanding of reactive oxygen species and their biological roles.
Background and Context
In biological systems, ROS arise from various sources, including mitochondrial respiration and cellular metabolism. They can serve as signaling molecules, yet their accumulation can provoke significant oxidative stress. Strategies for measuring these species have evolved from simple chemical assays to sophisticated imaging techniques.
Research indicates that effective measurement of ROS is multifaceted. It requires consideration of various factors, such as
- The specific ROS targeted
- The cellular environment
- The timing of measurements
The continuous advancements in methodologies provide opportunities for deeper insights into cellular mechanisms. As these technologies evolve, they not only enhance accuracy but also broaden the applications of ROS measurement in clinical and research settings.
Preamble to Reactive Oxygen Species
Reactive oxygen species, commonly known as ROS, emerge as key players in cellular physiology. Understanding ROS is vital for both biological research and medical applications. These species are not merely byproducts of cellular metabolism but rather significant molecules that influence numerous physiological processes. Recognizing their implications provides insight into both healthy cellular function and the pathophysiology of various diseases.
Definition and Types of ROS
Reactive oxygen species include a variety of unstable molecules that contain oxygen. The primary types are singlet oxygen, superoxide anion, hydrogen peroxide, and hydroxyl radicals.
- Singlet Oxygen: This is an excited form of oxygen, which plays a role in photochemical reactions.
- Superoxide Anion: This molecule results from the one-electron reduction of molecular oxygen. It serves as a precursor to other ROS.
- Hydrogen Peroxide: Although less reactive, it acts as a signaling molecule in cells. It can also lead to oxidative stress when present in high concentrations.
- Hydroxyl Radical: This is one of the most reactive species, migrating quickly and causing significant damage to macromolecules like DNA and lipids.
The balance between these various forms is crucial to maintaining cellular health and preventing damage.
Sources of ROS in Biological Systems
The production of ROS occurs from endogenous and exogenous sources. Endogenous sources include:
- Mitochondrial respiration: This process generates superoxide during the electron transport chain function.
- NADPH oxidases: Enzymes in the plasma membrane produce superoxide, especially in immune cells.
- Peroxisomes: These organelles generate hydrogen peroxide during fatty acid metabolism.
Exogenous sources include: - Environmental stressors: UV radiation, pollution, and chemicals contribute to ROS formation.
- Infections: Certain pathogens stimulate ROS production during immune responses.
Roles of ROS in Cellular Functions
While ROS are often associated with cell damage, they also have essential roles in cellular signaling. For example, ROS participate in redox signaling, regulating various pathways including cell proliferation and apoptosis. Additionally, they help in defense mechanisms against pathogens.
"The dual nature of ROS invites both protective and damaging responses, underscoring their importance in cellular dynamics."
In summary, ROS are crucial in maintaining cellular homeostasis. Yet, their dysregulation can lead to oxidative stress, contributing to diseases such as cancer, neurodegenerative disorders, and cardiovascular conditions. Thus, studying ROS goes beyond understanding mere chemical reactions; it encompasses a comprehensive understanding of cellular life itself.
The Importance of Measuring ROS
Understanding the role of reactive oxygen species (ROS) in cellular biology is critical for various fields, particularly in health and disease management. Measuring ROS provides insight into the dynamic biological processes that are influenced by oxidative stress. By quantifying ROS levels, researchers can evaluate their effects on cellular functions, contributing to a greater understanding of physiological and pathological states.
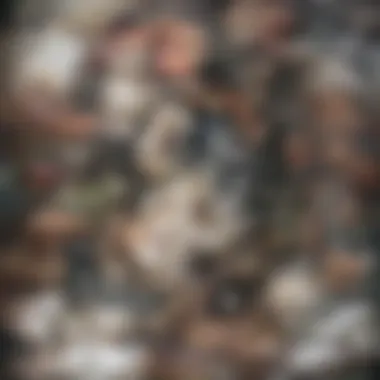
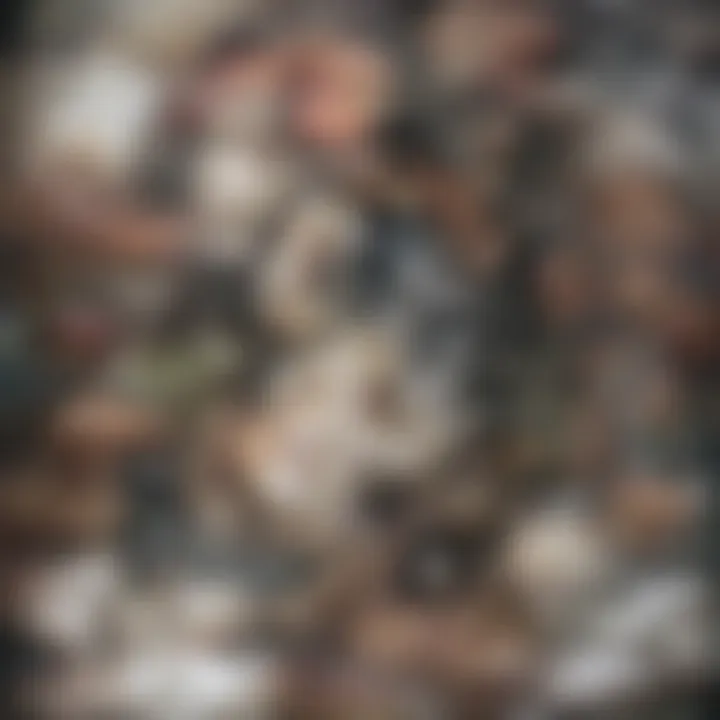
Significance in Health and Disease
The measurement of ROS is pivotal in studying numerous health conditions. Elevated ROS levels often correlate with significant health issues, such as cancer, neurodegenerative disorders, and cardiovascular diseases. For instance, in cancer research, high ROS levels can both initiate and promote tumorigenesis. Investigating these correlations can lead to identifying potential therapeutic targets.
Conversely, in conditions such as neurodegenerative diseases, ROS are believed to contribute to neuronal damage. Monitoring ROS allows scientists to understand disease mechanisms better and to test potential interventions. Clinical applications of ROS measurements provide real-time data that can allow for personalized treatment approaches.
Impact of Oxidative Stress
Oxidative stress occurs when there is an imbalance between ROS production and cellular antioxidant defenses. This imbalance can lead to cellular damage, affecting proteins, lipids, and DNA, which may result in severe health consequences.
Measuring ROS serves as a crucial assessment tool for oxidative stress. By identifying stress markers, researchers can evaluate the efficacy of antioxidants in mitigating damage. For example, substances like Vitamin C and E are known to combat oxidative stress.
Furthermore, oxidative stress markers can offer predictive insights in clinical settings. Understanding how different sources of ROS contribute to oxidative stress may lead to improved disease prevention strategies.
"Examining ROS measurement is vital for comprehending their involvement in health and disease, providing pathways for innovative treatment approaches."
In summary, measuring ROS encompasses several key aspects. It helps define the pathological role of oxidative stress, assess treatment outcomes, and improve clinical approaches to various diseases. This understanding informs the scientific community and enhances strategies for combating diseases influenced by ROS.
Methodologies for ROS Measurement
Measuring reactive oxygen species (ROS) in cellular contexts is crucial for understanding their roles in both health and disease. Various methodologies have emerged to quantify ROS due to their dynamic and transient nature. This section delves into several prominent techniques that not only allow for effective ROS detection but also shed light on their implications in biological processes. The choice of methodology heavily influences the insights gained from experiments. Therefore, selecting the right technique is paramount for achieving accurate results.
Fluorescence-Based Techniques
Fluorescence-based techniques are often the first choice for ROS measurement due to their sensitivity and simplicity. They utilize specific fluorescent probes that react with ROS, producing a measurable signal. Among these methods, there are notable assays like the Dichlorofluorescein Assay and the Dihydroethidium Assay, which are both widely accepted in the field.
Dichlorofluorescein Assay
The Dichlorofluorescein Assay is well-known for its effectiveness in detecting hydrogen peroxide and other ROS. This assay works based on the conversion of an inactive dichlorodihydrofluorescein compound to a fluorescent compound in the presence of ROS. A key characteristic of this method is its ability to provide real-time measurements of ROS levels in live cells. This feature positions the Dichlorofluorescein Assay as a beneficial choice for researchers focusing on dynamic changes in oxidative stress within various biological systems.
However, one must consider the assay's limitations, such as potential interference from cellular components and a tendency to give false positives in some contexts. Despite these disadvantages, its ease of use and effectiveness make it a popular selection for evaluating ROS in experimental setups.
Dihydroethidium Assay
Another significant tool in the toolbox for measuring ROS is the Dihydroethidium Assay. This method is primarily used for the detection of superoxide anions. The key characteristic of this assay lies in its specific reaction with superoxide, leading to the production of a red fluorescent product. This specificity makes it a valuable resource for researchers examining particular oxidative species.
Its unique feature is the ability to selectively indicate the presence of superoxide in live cells, which is advantageous for understanding cellular processes influenced by this specific ROS. However, it is essential to note that the Dihydroethidium Assay can also have drawbacks, such as photobleaching and the potential for interference from other cellular molecules that may affect signal accuracy.
Chemiluminescence Methods
Chemiluminescence methods leverage chemical reactions that emit light to quantify ROS. This allows for highly sensitive detection. These techniques often benefit from low background interference and high specificity. The emitted light correlates with ROS concentration, facilitating direct and quantifiable measurements. However, these methods may require sophisticated equipment and can be complicated by factors like local environment conditions within the test sample, which must be controlled during experiments.
Electrochemical Sensors
Electrochemical sensors represent another innovative approach for ROS measurement. These sensors operate by detecting changes in the chemical properties of ROS through electrochemical reactions. This method allows for real-time monitoring of ROS levels. The advantages include high sensitivity and the ability to be miniaturized for in vivo measurements. Nevertheless, the development and calibration of these sensors can be complex, and they may not be as widely available as other techniques.
Mass Spectrometry Approaches
Mass spectrometry approaches offer another avenue for assessing ROS. This technique is particularly useful for providing detailed information about the types and quantities of ROS present. Mass spectrometry can distinguish between different ROS and their reaction products, enabling a comprehensive understanding of oxidative stress in biological systems. However, this technique is often more expensive and requires specialized skills and equipment. Additionally, it may not always provide real-time data, which is a drawback when studying dynamic cellular events.
In summary, the methodological landscape for measuring ROS is diverse, each offering distinct strengths and weaknesses. The choice of method should align with the specific requirements of the study, emphasizing the careful consideration of the biological context in which ROS are being measured.
Assessment of Measurement Techniques
In the realm of measuring reactive oxygen species (ROS), assessing the techniques used is fundamental. Measurement methods should provide accurate and reliable results to understand the biological implications of ROS fully. Different techniques have specific strengths, yet they also come with limitations. Therefore, a careful evaluation of these methods is essential for researchers. The focus on assessment includes sensitivity, specificity, and the ability for real-time monitoring, which will all be explored further below.
Sensitivity of Measurement Methods
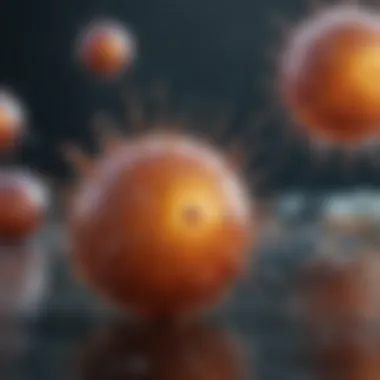
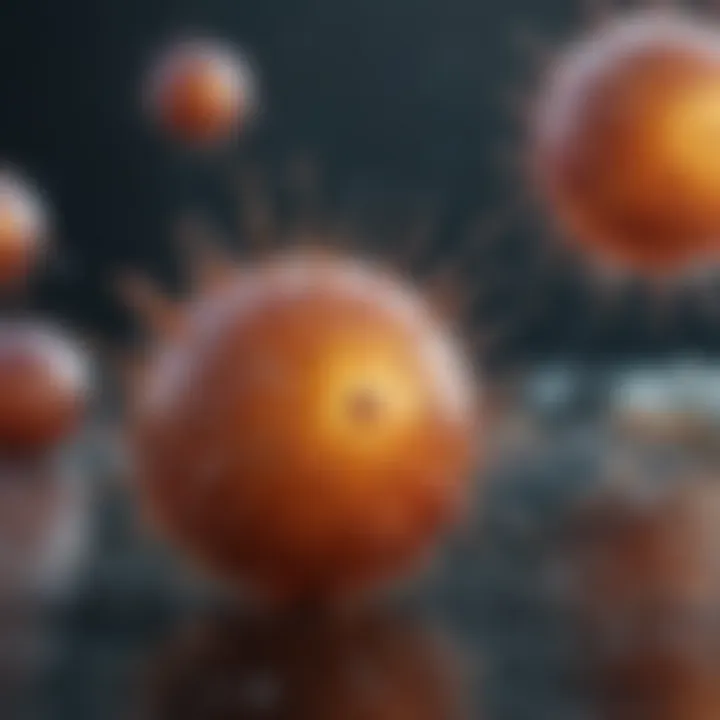
Sensitivity refers to a method's ability to detect low concentrations of ROS. It is crucial for accurately representing biological conditions. If a method is not sensitive enough, it might overlook important fluctuations of ROS that could impact cellular health. Methods like fluorescence-based techniques can be very sensitive, allowing researchers to detect minute changes in ROS levels. However, sensitivity can also lead to the potential issue of noise, as even minor interferences can skew measurements. Balancing sensitivity with the potential for false positives is key in research settings.
Specificity and Selectivity of Assays
Specificity refers to how well a method can identify ROS without being influenced by other reactive species. This quality ensures that the data obtained reflects true biological activity rather than artifacts from other cellular components. Selectivity, on the other hand, guarantees that certain types of ROS are distinguished from others. In practice, techniques such as the Dihydroethidium assay stand out for their selectivity towards superoxide anions. Researchers must consider both specificity and selectivity to obtain precise data for their studies.
Real-Time Monitoring Capabilities
The ability to monitor ROS levels in real-time is an emerging advantage of various modern techniques. Traditional methods often involve endpoint measurements that fail to capture dynamic changes in cellular environments. Real-time monitoring provides insights into the rapid fluctuations in ROS, offering a more detailed view of cellular responses over time. For example, advanced electrochemical sensors can track ROS levels continuously, which helps in understanding processes like oxidative stress during different phases of cell life. This capability brings researchers closer to comprehending how ROS impact health and disease in living systems.
Understanding the assessment of measurement techniques is vital for effectively studying ROS. By focusing on sensitivity, specificity, and real-time monitoring, researchers can gain deeper insights into cellular health and disease mechanisms.
Applications of ROS Measurement
Measuring reactive oxygen species (ROS) holds significant value in numerous scientific and medical domains. These applications extend beyond basic research into vital areas such as disease management and drug development. Understanding how to effectively measure ROS can provide insights into various health conditions and contribute to the development of innovative treatment strategies.
Clinical Implications in Disease Management
Cancer
Cancer presents one of the most critical areas for ROS measurement. Tumor cells often exhibit altered redox states compared to normal cells. This imbalance can lead to increased levels of ROS, which further promotes tumor progression. The ability to measure ROS allows for better understanding of these mechanisms. For instance, treatments aimed at increasing oxidative stress can selectively harm cancer cells while sparing normal cells. This characteristic makes cancer a fascinating subject in the study of ROS.
Key Characteristics of Cancer:
- Abnormal proliferation
- Enhanced metabolic activity
Such traits provide new avenues for therapeutic intervention.
The unique aspect of cancer as a target for ROS measurement is its selective vulnerability to oxidative changes. However, the advantage of targeting ROS in cancer therapy comes with a caveat; excessive oxidative stress can lead to resistance. Thus, precision in ROS measurement is crucial for optimizing treatment regimens.
Neurodegenerative Diseases
Neurodegenerative diseases such as Alzheimer's and Parkinson's also feature prominently in the context of ROS measurement. These conditions are characterized by an accumulation of oxidative damage, ultimately leading to cellular dysfunction. By measuring ROS levels, researchers can track disease progression and response to treatments.
Key Characteristics of Neurodegenerative Diseases:
- Progressive neuronal loss
- Impaired cognitive functions
Understanding ROS in these diseases helps in developing biomarkers for early diagnosis and monitoring disease activity. The unique feature here connects ROS measurement to mitochondrial dysfunction, a hallmark in many neurodegenerative conditions.
In this context, the advantage of measuring ROS lies in its potential to identify therapeutic targets. Treatments could be designed to reduce oxidative stress and subsequently slow disease progression. However, the challenge remains in discerning which ROS are beneficial and which contribute to pathology.
Role in Drug Development
In drug development, measuring ROS is equally vital. As researchers explore new therapeutic compounds, understanding the oxidative properties of these compounds becomes essential. Drugs that can modulate ROS levels are now under investigation for their roles in various diseases, particularly inflammatory conditions.
Benefits include:
- Targeting oxidative stress directly
- Inducing cell death in cancer cells via ROS overproduction
Additionally, using ROS as pharmacological targets can lead to more effective therapeutics. This has become a popular approach in designing new drugs, influencing both efficacy and safety.
Challenges in ROS Measurement
Understanding the challenges in measuring reactive oxygen species (ROS) is essential for advancing biological research and clinical applications. Despite the significance of ROS in cellular functions and disease processes, accurately measuring these species in living cells remains a complex task. Key challenges arise from the limitations of existing techniques, difficulties in quantifying ROS levels, and the variability posed by experimental conditions. Each of these elements contributes to the ongoing effort to achieve reliable, reproducible results in ROS research.
Limitations of Current Techniques
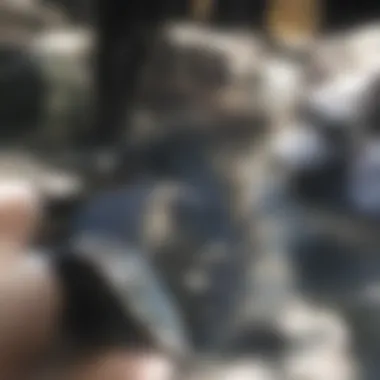
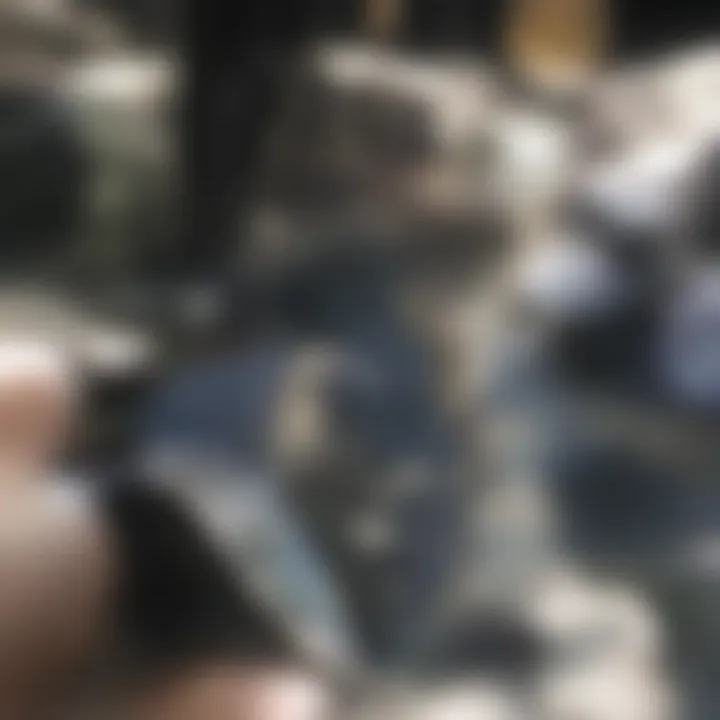
Current techniques used for ROS measurement, such as fluorescence-based assays and chemiluminescence methods, have inherent limitations.
- Sensitivity Issues: Some techniques may not detect low levels of ROS, which can be crucial in understanding subtle cellular changes. This lack of sensitivity can lead to underestimating ROS presence in various situations.
- Interference from Other Cellular Components: Assays sometimes suffer from interference caused by other oxidative species or non-specific reactions, leading to inaccurate readings.
- Short-lived Nature of ROS: ROS are highly reactive and often exist for very short durations. Capturing these fleeting species efficiently is challenging and limits the methods' effectiveness.
These limitations necessitate the development of improved methodologies that can address existing shortcomings in ROS detection.
Difficulty in Quantification
Quantifying ROS accurately poses another significant challenge. ROS exist in various forms and react quickly with cellular components, complicating their measurement.
- Dynamic Nature: As ROS concentrations fluctuate rapidly due to changes in cellular metabolism, obtaining precise readings becomes complex.
- Calibration Issues: Many assays require calibration against known concentrations, which can be difficult due to the varying reactivity of ROS.
- Unit Consistency: Different techniques often employ various units and standards, creating inconsistencies in quantifying ROS levels across studies.
These factors hinder researchers from obtaining a clear understanding of the role of ROS in cellular biology and disease states.
Variability in Experimental Conditions
Experimental conditions can vary considerably in studies involving ROS measurement, leading to another layer of complexity. Factors that contribute to variability include:
- Sample Preparation: Differences in cell handling and treatment protocols can affect ROS levels.
- Environmental Factors: Conditions such as temperature, pH, and oxygen levels can influence ROS production and stability within cells.
- Timing of Measurements: When measurements are taken also affects outcomes, as ROS levels can fluctuate significantly over time.
This variability complicates comparisons between different studies and can lead to inconsistent results, making it harder to draw definitive conclusions about the biological roles of ROS.
"The challenges in ROS measurement reflect the need for continuous innovation in detection methods to better capture this elusive aspect of cellular biology."
In addressing these challenges, researchers can improve the accuracy and reliability of ROS measurements, ultimately advancing our understanding of their implications in health and disease.
Future Directions in ROS Research
Research on reactive oxygen species (ROS) is rapidly advancing, revealing new dimensions of their roles in cellular mechanisms. The future of ROS research focuses on enhancing detection techniques and understanding ROS's complex interactions within biological systems. Addressing these areas presents several benefits. For instance, improving measurement precision can lead to better insights into the pathology of diseases linked to oxidative stress. Moreover, innovative approaches can facilitate the exploration of novel therapeutic interventions aimed at modulating ROS levels in cells.
Innovative Detection Methods
There is a strong impetus to develop innovative detection methods for ROS, which may lead to greater accuracy and real-time monitoring capabilities in cellular environments. Recent advances have introduced new fluorescent probes that are sensitive to specific ROS, allowing researchers to visualize oxidative species in living cells with high specificity. These probes generally possess unique chemical structures designed to react with particular types of ROS, such as hydrogen peroxide or superoxide. For example, the use of genetically encoded biosensors provides a powerful perspective on ROS dynamics inside cells, as these sensors can provide insights in real-time and under physiological conditions.
"The evolution of detection methods will define the trajectory of our understanding of ROS in various biological contexts."
In addition to fluorescence methods, electrochemical sensors are being refined for ROS detection. These sensors offer the advantages of simplicity and high throughput, making them suitable for real-world applications. Combining these novel methods can improve the sensitivity and resolution of ROS measurements significantly.
Integration of Multimodal Approaches
The integration of multimodal approaches is crucial in the future study of ROS. By combining different methodologies, such as electrochemical sensors with mass spectrometry, researchers can obtain a more comprehensive understanding of the oxidative state within cells. Such integration would not only enhance the specificity of measurements but also allow for cross-validation between methods.
For instance, employing mass spectrometry can provide detailed molecular information about ROS and their reactions, while electrochemical detection can quantify the levels of these species in real-time. This synergy between different technologies allows more robust data collection and interpretation, leading to more informed conclusions regarding cellular redox states.
In clinical settings, employing multimodal detection systems could revolutionize the way diseases are monitored and treated. Personalizing therapies based on real-time ROS measurements could immensely improve patient outcomes.
Ending
The conclusion serves as a pivotal element in this article by synthesizing the content covered and underscoring the importance of measuring reactive oxygen species (ROS) in cells. This section summarizes crucial insights gained regarding ROS, their roles in biological systems, and the methodologies employed for their measurement. Given the prominence of oxidative stress in various health conditions, understanding ROS dynamics is essential for researchers and practitioners alike.
Summary of Key Insights
In reviewing the content of this article, several key insights emerge:
- Definition and Types of ROS: Understanding the various forms of ROS, such as superoxide and hydrogen peroxide, allows for better insight into their specific roles in cellular processes.
- Sources and Impact: Recognizing the sources of ROS in biological systems aids in comprehending how oxidative stress arises and its subsequent effects on cell function.
- Measurement Techniques: Multiple methodologies for measuring ROS, including fluorescence-based techniques and electrochemical sensors, are explored, showcasing their individual strengths and limitations.
- Implications for Health: The relationship between ROS and disease, particularly in areas like cancer and neurodegenerative conditions, highlights the need for precise measurement to develop effective interventions.
"Accurate measurement of ROS not only enhances our understanding of cellular mechanisms but also informs clinical strategies in disease management."
This synthesis not only reinforces the importance of ongoing research into ROS but also emphasizes the need for innovation in measuring techniques to address current limitations.
The Future of ROS Measurement
As we look ahead, the future of ROS measurement is promising yet presents challenges. Current methodologies must evolve to increase their sensitivity and specificity. Furthermore, there is a noticeable trend toward integrating multimodal approaches, combining various techniques to provide a comprehensive view of ROS dynamics in cellular contexts. Researchers are likely to explore innovative detection methods that harness advances in technology, enabling real-time monitoring of ROS levels in living cells. This progression is essential for translating laboratory findings into practical health solutions.