Phusion PCR Protocol: Insights and Applications
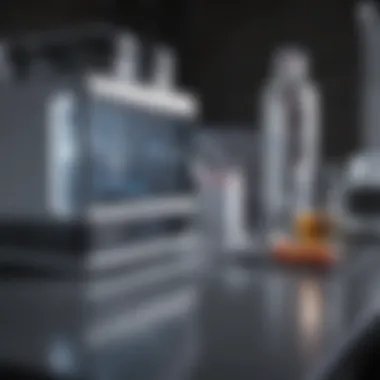
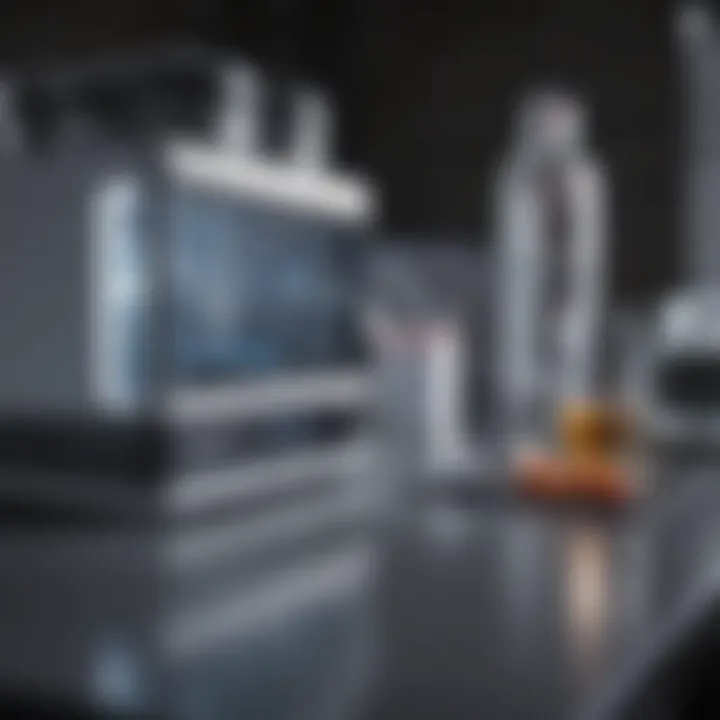
Intro
In the realm of molecular biology, the ability to amplify DNA sequences without introducing errors is paramount. High-fidelity polymerases have revolutionized how scientists approach molecular cloning, gene expression analysis, and genetic engineering. Among these, Phusion polymerase stands out as a premier choice. This article intends to unfold the intricacies of the Phusion PCR protocol and its substantial implications in the lab.
Research Overview
Summary of Key Findings
The Phusion PCR protocol offers several advantages that significantly streamline research processes. Researchers consistently find that it generates higher yield and specificity with fewer mutations compared to traditional Taq polymerase methods. Moreover, the fast thermal cycling of the Phusion polymerase allows for quicker overall experiment times while maintaining accuracy. Through examining various studies, this article lays out these benefits, providing insights into the optimization of PCR experiments.
Background and Context
PCR, or polymerase chain reaction, has long been the backbone of molecular biology. Traditional methods, while valuable, often fall short in precision, especially when accuracy is essential. Previously, labs had to choose between yield and fidelity; Phusion polymerase changes the game entirely by enhancing both qualities. This change isn’t just a minor update but a transformational leap, offering scientists new avenues for exploration.
Methodology
Experimental Design
The impact of the Phusion PCR protocol was assessed through a series of comparative studies against earlier methods. By using specific templates and controlling variables like temperature and cycle counts, the experiments aimed to provide a comprehensive overview of performance metrics. The choice of primers, MgCl2 concentration, and other critical reagents were also standardized.
Data Collection Techniques
Data was meticulously collected via gel electrophoresis, allowing for visualization and quantification of PCR products. High-resolution photography was employed to ensure accurate documentation. Statistics were employed to analyze amplification efficiency, error rates, and product yield, providing a robust set of results that highlights the efficacy of the protocol.
Phusion polymerase has emerged as a robust solution to common issues found in previous PCR methodologies, primarily dealing with accuracy and yield.
In summary, the Phusion PCR protocol is not just another tool in a geneticist's toolbox; it's a pivotal advancement that opens the door to new opportunities and greater accuracy in research. As we dive deeper into statistics, applications, and troubleshooting strategies, this article aims to be a comprehensive resource for both seasoned researchers and newcomers in the field.
Prolusion to Phusion PCR
In the realm of molecular biology, the ability to amplify DNA with precision is paramount. This is where Phusion PCR comes into play. It’s not just another method on the block; it's a standout technique that significantly enhances the fidelity, specificity, and overall outcome of DNA amplification. Understanding this protocol sets the stage for more effective experimental design and exploration of genetic material.
Overview of PCR Techniques
Polymerase Chain Reaction (PCR) techniques have revolutionized genetics since Kary Mullis first developed them in the 1980s. The core idea remains the same: replicate a targeted segment of DNA. Traditional PCR methods, such as Taq polymerase, paved the way for molecular biology breakthroughs but tend to introduce errors during amplification. This is where Phusion PCR shows its true colors.
Phusion polymerase, derived from a Thermus thermophilus strain, offers higher fidelity due to its robust proofreading abilities. For instance, while Taq polymerase might make an error rate of about 1 in 1000 bases, Phusion boasts an error rate closer to 1 in 1 million. That stark difference is critical for downstream applications like cloning and sequencing, where accuracy is non-negotiable.
Moreover, Phusion PCR incorporates exquisite temperature control and precise annealing, optimizing the process beyond what we might come to expect from earlier PCR techniques. Having a clear grasp of these elements will undoubtedly empower students and researchers to design experiments that yield higher quality results.
Significance of High-Fidelity PCR
Why should one care about high-fidelity PCR? Well, the answer lies in the outcomes of critical research and experiments. High-fidelity PCR is vital for producing accurate PCR products, maintaining the integrity of the original DNA sequence. This is paramount in processes like cloning where you want the inserted gene to be exactly as intended.
"Errors in the amplification can lead to misinterpretations in studies, derail experiments, and skew results."
Moreover, high-fidelity methods are often crucial when working with complex templates, such as genomic DNA, where unwanted mutations could lead to drastically different biological implications. In clinical diagnostics, for instance, the precision of amplification can influence the accuracy of test results, leading to proper diagnoses and treatment plans.
Research applications that hinge on accurate DNA sequence amplification benefit immensely from Phusion PCR's meticulous approach. Applications range from basic research, such as gene function studies, to advanced techniques like CRISPR and next-generation sequencing.
Simply put, high-fidelity PCR is more than a procedure; it's a cornerstone of effective molecular biology that enables breakthroughs in genetics and associated fields.
Composition of Phusion PCR Protocol
The composition of the Phusion PCR protocol stands as a pillar in its effectiveness, intricately influencing the outcomes of PCR experiments. Understanding the components not only provides insights into the functionality of the process but also reveals how different variables interplay to either enhance or hinder the amplification of DNA. Each element of the protocol holds significance, and recognizing their roles can catapult the efficiency of experiments to new heights.
Components of the Reaction Mix
In any PCR protocol, the reaction mix is the bread and butter. For Phusion PCR, this mix typically comprises water, DNA template, primers, nucleotides, and the Phusion polymerase itself. Each component contributes uniquely to the process:
- Water: Acts as the solvent, diluting the other components to ensure proper enzymatic activity.
- DNA Template: This is the starting material from which the target DNA will be amplified.
- Primers: Short sequences that flank the target DNA region, crucial for initiating synthesis. The choice of primers is vital, as incorrectly designed primers can lead to failed reactions or non-specific amplification.
- Nucleotides (dNTPs): The building blocks of DNA, dNTPs are the resources that the polymerase will use to construct the new DNA strands.
- Phusion Polymerase: A high-fidelity enzyme that accounts for the accuracy and efficiency of the amplification process.
The careful calibration of these components is fundamental. If any ingredient is amiss — too little primer, a tainted template, or subpar polymerase — the whole endeavor can backfire. Thus, understanding the right proportions and quality of each element is of great importance.
Role of Phusion Polymerase

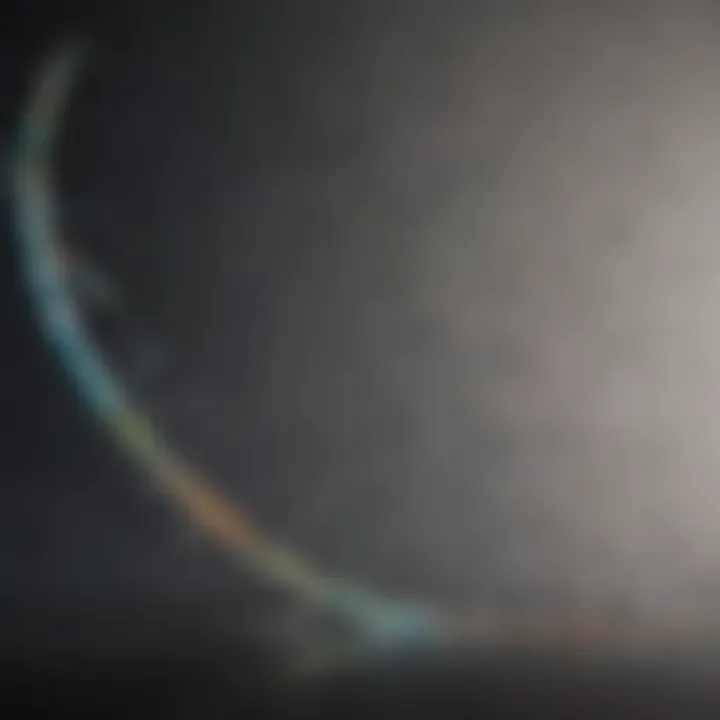
Phusion polymerase is not just a run-of-the-mill enzyme; it’s a sophisticated tool tailored for high-fidelity DNA amplification. The enzyme derives its name from its remarkable ability to produce DNA with high accuracy, which is paramount in applications requiring precise mutations or sequences.
- High-Fidelity Nature: Phusion polymerase boasts a lower error rate than standard Taq polymerase, making it preferable for cloning and sequencing tasks where accuracy is non-negotiable.
- Robust Performance: It can withstand higher temperatures, and it’s designed to handle challenging templates, including those with secondary structures.
- Processivity: With a unique mechanism, it remains attached to the DNA strand longer than ordinary polymerases, which enhances the elongation speed and efficiency.
The enzyme’s robust qualities make it a favored choice in molecular biology laboratories where precision is key.
Buffer System and its Importance
A PCR buffer system is another integral part of the Phusion PCR protocol. It is not merely an afterthought. In essence, buffers maintain the optimal pH and ionic environment necessary for optimal enzyme activity and stability. Here's why the buffer is impactful:
- pH Stability: Enzymatic reactions are highly sensitive to pH. If the buffer fails to keep the pH within the ideal range, even the best polymerase could falter.
- Salt Concentration: Buffers often contain salts, which help stabilize the negative charges on DNA phosphate backbones, enabling effective annealing of primers to the template. The right salt concentration can significantly enhance the selectivity of the amplification process.
- Detergents and Additives: Some buffers are augmented with detergents or additives to improve enzyme handling and reduce non-specific binding.
In summary, the buffer system is a foundational element that supports the entire reaction, ensuring that all other components can function harmoniously. By deeply understanding these aspects, researchers can better troubleshoot potential pitfalls and tailor their reactions for optimized outcomes.
Step-by-Step Process of Phusion PCR
Understanding the step-by-step process of Phusion PCR is essential for achieving accurate and high-fidelity DNA amplification. Each phase plays a pivotal role in ensuring that the reaction runs smoothly, yielding reliable results that can be utilized in various fields such as genetics, molecular biology, and biotechnology. Knowing how to navigate through these intricacies not only enhances the efficacy of protocols but also aids in troubleshooting when things don't go as planned. Let’s break down the key phases that compose this indispensable technique.
Initial Denaturation
The first step in any PCR process, including Phusion PCR, is the initial denaturation, and it sets the stage for everything that follows. In this phase, the temperature is raised to around 98°C, which causes the double-stranded DNA template to separate into two single strands. This moment is critical because it essentially "unlocks" the genetic information contained in the DNA, making it accessible for the subsequent steps.
- Duration: Typically, this step lasts for about 30 seconds. However, the exact time can vary based on the DNA template. For complex templates, extending this duration slightly may sometimes yield better results.
- Temperature Sensitivity: The high temperature is necessary to disrupt hydrogen bonds that hold the DNA strands together. Hence, it’s not advisable to skimp on this phase; without proper denaturation, the efficiency of the overall reaction decreases.
This preliminary step can be likened to the foundation of a house. Just as a solid base supports the structure above, successful denaturation ensures a robust foundation for the remainder of the amplification process.
Annealing Temperature Optimization
Once the DNA strands are denatured, the next step involves cooling the reaction mixture to allow the primers to bind to their complementary sequences on the single-stranded DNA. This step, referred to as annealing, requires careful consideration of temperature settings.
- Importance of Annealing Temperature: The exact temperature for this step generally ranges from 50°C to 65°C, depending on the melting temperature of the primers used. If the temperature is too low, primers may bind non-specifically, which can lead to unwanted products; too high, and they may not bind at all.
- Optimization Strategies: To find the sweet spot for annealing temperature, one can conduct a gradient PCR experiment. This technique involves testing different temperatures in parallel, which provides a clearer picture of where optimal binding occurs.
- Concentration of Primers: The amount of primers can also influence the annealing process. Using primers in excess can increase binding efficiency, but it might also lead to non-specific annealing under certain conditions.
By honing in on the perfect annealing temperature, one can ensure that only the desired DNA sequences are amplified, thereby significantly enhancing the specificity of the results.
Extension Phase and Its Duration
After successful annealing, we move on to the extension phase, where the actual synthesis of the new DNA strand occurs. This step is where Phusion polymerase showcases its prowess.
- Optimal Temperature for Extension: Typically performed at around 72°C, this phase allows Phusion polymerase to add nucleotides to the 3' end of the primers, effectively synthesizing complementary strands to the original template. At this temperature, Phusion polymerase operates at its peak efficiency, which is crucial for high-fidelity amplification.
- Duration: The extension time varies with the length of the target DNA. A general rule of thumb is about 10-30 seconds per kilobase of DNA to be amplified. Adhering to this guideline helps in optimizing the amplification without extending the run time unnecessarily.
Proper execution of the extension phase can be likened to watching a skilled artist at work, where each stroke of the brush (or nucleotide addition) contributes to the final masterpiece of DNA sequence amplification.
Getting the step-by-step process right lays the groundwork for a successful Phusion PCR, ensuring the fidelity and specificity of the amplification.
Comparison with Other PCR Methods
When looking to expand one’s understanding of PCR methodologies, comparing Phusion PCR with other techniques is key. This section serves as a junction point where readers can appreciate not only the features of Phusion PCR but also the landscape of polymerase chain reaction methods. By examining the similarities and differences, we can clearly articulate why researchers might opt for Phusion PCR as their tool of choice.
Advantages of Phusion PCR
Phusion PCR stands proud in the PCR realm. It boasts several benefits that make it particularly attractive for a variety of applications:
- High Fidelity: One of the most significant advantages is its low error rate during DNA synthesis, which is crucial for applications requiring precise genetic alterations. Estimates suggest its error rate is about 50 times lower than that of traditional Taq polymerase, giving researchers confidence in the accuracy of amplified products.
- Robustness: Phusion is designed to work efficiently across a broad range of templates and conditions. Whether you’re working with complex genomic DNA or sparse templates, this polymerase exhibits reliable performance.
- Rapid Amplification: The enzyme operates over a wider temperature range, which allows for shorter extension times without compromising the integrity of the PCR product. This efficiency can be a game-changer in time-sensitive experiments.
In practical terms, these advantages combine to offer high-yield, precise results that enable researchers to push the boundaries of their studies, which can ultimately translate into innovations in fields as diverse as genetics, microbiology, and forensic science.
Limitations and Drawbacks
However, even a star performer like Phusion PCR comes with its own set of limitations that warrant consideration:
- Cost: Phusion polymerase tends to be on the pricier side when stacked against alternatives like Taq polymerase. For labs operating under tight budgets, this can be a factor that complicates its use.
- Optimizing Conditions: It often requires more meticulous optimization than other methods. Finding the right annealing temperatures and extension times can be a nuanced process, and for less experienced practitioners, this could translate into hurdles.
- Complexity of Procedure: The setup may be perceived as more complicated due to its rigorous requirements for reaction conditions. Dual primers and higher concentrations of magnesium can be daunting for those used to the one-size-fits-all approach of simpler PCR techniques.
Thus, while Phusion PCR shines in many scenarios, understanding its drawbacks is vital for making informed decisions in laboratory settings.
Application Scenarios for Each Method
Different PCR methods have niches where they excel, and understanding these can help researchers choose appropriately:
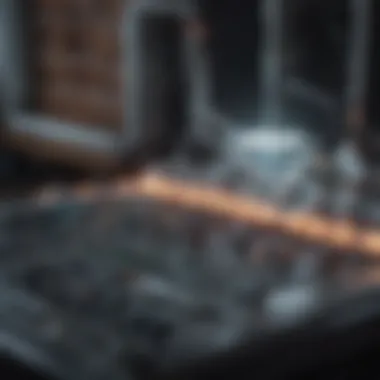
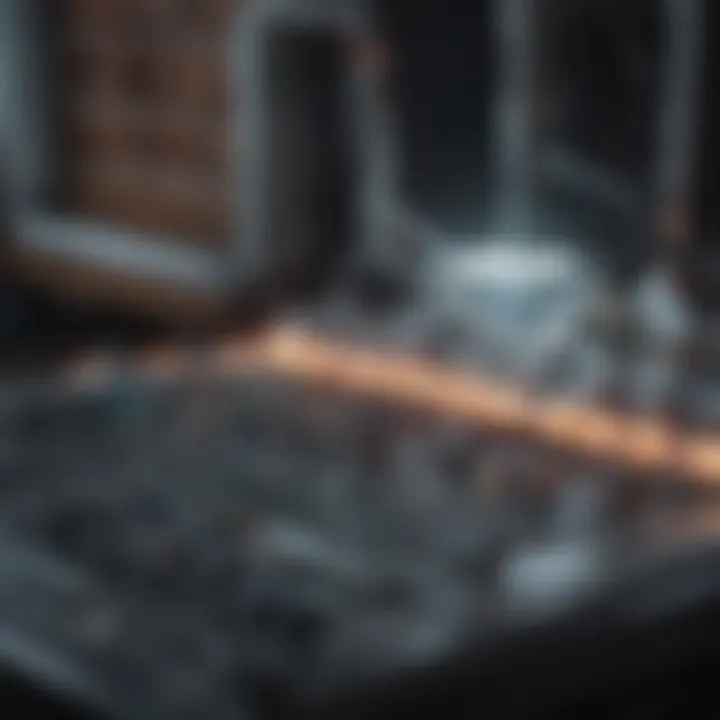
- Phusion PCR: Best suited for cloning and amplifying high-fidelity DNA fragments, particularly when working with a high complexity of templates. This is the go-to method when accuracy is non-negotiable, for instance in creating DNA for mutagenesis or sensitive applications like sequencing.
- Taq PCR: This method is generally more suited for routine applications where speed and convenience matter. It can be effective when working with relatively simple tasks, such as amplifying gene fragments for downstream analysis in cases where precision doesn’t take precedence over time.
- Q5 High-Fidelity DNA Polymerase: Another high-fidelity option, this is often favored in demanding applications similar to Phusion, but has slight differences in enzyme characteristics that may make it preferable based on specific experimental conditions.
In summary, understanding the comparative merits and limitations of these methods not only helps in selecting the right tool for each experiment but also adds a layer of depth to one’s molecular biology toolkit.
Applications of Phusion PCR in Research
The applications of Phusion PCR are vast and varied, playing a crucial role in advancing the fields of molecular biology and genetics. This method has dramatically improved the accuracy and efficiency of DNA amplification, setting a standard for precision and reliability in research. Understanding the applications of Phusion PCR helps researchers better leverage its capabilities to drive innovative discoveries and solutions in their fields.
Genomic DNA Amplification
One notable area where Phusion PCR shines is in genomic DNA amplification. This process is essential for studying the structure, function, and regulation of genes within an organism's DNA. Phusion PCR stands out due to its high fidelity, allowing for the generation of specific DNA fragments without significant errors.
This accuracy is particularly beneficial for downstream applications, such as sequencing and genetic analysis. In essence, researchers can amplify regions of interest with confidence that the resulting sequences reflect the true nature of the genetic material. A few practical considerations when employing Phusion PCR for genomic DNA amplification include:
- Template Quality: Ensure the quality of the genomic DNA is high, as impurities can affect amplification efficiency.
- Primer Design: Well-designed primers enhance specificity and efficiency, so spending time on this step pays off.
- Thermal Cycling Conditions: Optimizing the cycling conditions based on the template and primers is key to success.
Cloning and Plasmid Construction
Phusion PCR is heavily utilized in cloning and plasmid construction due to its ability to amplify long fragments of DNA with high precision. Cloning relies on the insertion of a DNA fragment into a vector to create recombinant DNA molecules that can be replicated within host cells.
With Phusion polymerase, researchers can generate high-quality inserts that can be efficiently ligated into vectors. This application is particularly important for the development of expression systems for proteins of interest, contributing to fields such as therapeutic protein production and enzyme development. Some advantages in employing Phusion PCR for cloning include:
- High Yield of Inserts: Ability to produce a large quantity of the desired DNA sequence.
- Minimal Misincorporation: Reduction in errors enhances the reliability of the clones produced.
- Versatility: Its application extends across various vectors and systems.
Key considerations include ensuring proper restriction sites on the vector and choosing the right selection markers to facilitate screening of successful clones.
Mutagenesis and Functional Studies
Mutagenesis and functional studies are other critical aspects of research greatly aided by Phusion PCR. This technique allows for site-directed mutagenesis, where researchers can introduce specific mutations into a DNA sequence. These mutations can be vital for understanding gene function, protein interaction, or enzyme activity.
One of the key benefits of using Phusion PCR in mutagenesis is the accuracy with which mutations can be incorporated.
- Precision: Mutations are introduced with a high degree of accuracy, minimizing any unwanted changes in the DNA sequence.
- Flexible Conditions: The protocol can be adapted to introduce a range of mutations, whether single nucleotide changes or larger deletions.
- Functional Analysis: Allows for detailed assessments of how these mutations affect the biology of the organism or systems under study.
Ultimately, Phusion PCR enhances experimental designs aimed at unraveling complex biological processes by providing reliable techniques for DNA manipulation.
Phusion PCR has allowed researchers to push the boundaries of genetic manipulation, revealing insights into biological systems that were previously beyond reach.
Troubleshooting Common Issues
In any endeavor, particularly one as nuanced as molecular biology, it's crucial to confront challenges head-on. When using the Phusion PCR protocol, various complications can emerge, often leaving researchers scratching their heads. Recognizing the significance of troubleshooting common issues is vital for both novice and seasoned practitioners. By dissecting these concerns, we not only improve our skills but also enhance our understanding of the reaction dynamics and ultimately boost the reproducibility and reliability of our results.
Low Yield of PCR Products
Experiencing a low yield of PCR products can be frustrating. This issue might arise from a number of different factors, such as improper concentrations of reagents, suboptimal annealing temperatures, or the intrinsic quality of the DNA template itself. Here are a few considerations to help navigate this issue:
- Template Quality: Always use high-quality genomic DNA or cDNA. Degraded samples can lead to poor amplification.
- Reagent Concentrations: Ensure that the concentrations of dNTPs, primers, and Phusion polymerase are optimized for your specific reaction.
- Annealing Temperature: Adjust the annealing temperature appropriately. It’s critical; too high can prevent primer binding, while too low can produce non-specific products.
A systematic approach, modifying one variable at a time, will help identify the underlying cause of low yield. Keeping careful records of your conditions and results is a good practice that pays off when troubleshooting.
Non-Specific Amplification
Non-specific amplification is another common pitfall in PCR reactions. When the desired product isn't the only one taking center stage, it can obscure your results significantly. Potential causes include too low an annealing temperature, poor primer design, or insufficient specificity of the primers. Here’s what to check:
- Primer Design: Ensure that your primers are specific to the target sequence. Consider using tools like Primer3 or NCBI's Primer-BLAST to assess specificity.
- Temperature Optimization: Experiment with different annealing temperatures to find the sweet spot where specific binding occurs without inviting non-specific reactions.
- Polymerase Fidelity: Phusion polymerase is known for its high fidelity. However, using it under suboptimal conditions can still lead to undesired products.
Utilizing a gradient PCR can be particularly beneficial for identifying the right conditions for your primers and templates.
Contamination Concerns
Contamination in PCR can lead to false positives, making it a concern that cannot be overlooked. Contaminants might arise from various sources, be it reagents, samples, or even the environment. Here are some strategies to minimize these risks:
- Use of Dedicated Equipment: Work with separate sets of pipettes and tips for pre- and post-PCR stages. This simple alteration can go a long way.
- Clean Workspace: Maintain a sterile environment. Regularly clean work surfaces and avoid clutter that can harbor unwanted nucleic acids.
- DNa-ases Treatment: Incorporate DNa-ases in your lab setup to eliminate any extraneous DNA that could interfere with your amplification efforts.
A robust control system, including negative controls and replicates, should be standard practice to catch any oddities early in the experimental phase.
By understanding and addressing these common issues, researchers can foster a greater degree of success with the Phusion PCR protocol. Such diligence not only improves the reliability of results but also enhances the overall research experience.
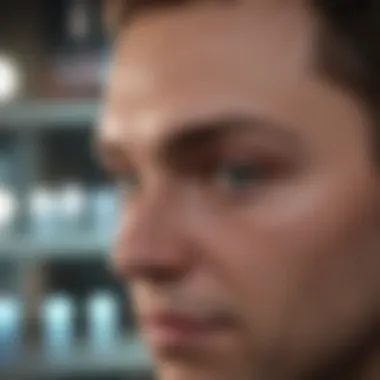
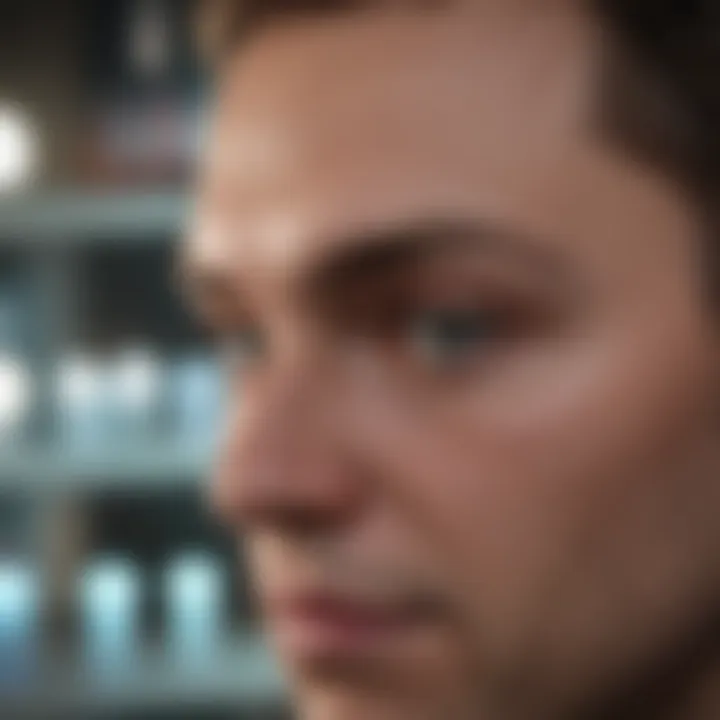
Best Practices for Successful Phusion PCR
The realm of molecular biology is not merely a series of pipette actions; it’s an intricate dance that balances precision with creativity. When utilizing the Phusion PCR protocol, applying best practices can spell the difference between a successful experiment and one mired in frustration. Understanding these key practices enhances both efficiency and result accuracy, making the whole endeavor a lot more rewarding.
Choosing Appropriate Primers
Primers function like the guiding stars in the PCR universe. Choosing the right ones can directly affect the yield and specificity of your target DNA amplification. You want primers that are just right—not too long, not too short. Ideally, they should be around 18 to 25 nucleotides long, providing a decent melting temperature, typically between 55°C and 65°C.
- Specificity: Primers should match the target region closely. A mismatch can lead to non-specific amplification. Using software tools can help you design primers with higher specificity.
- GC Content: Aim for a balanced GC content, around 40% to 60%. This balance facilitates stable binding while avoiding excessive secondary structures.
- No Self-Complementarity: Avoid primers that can form hairpins or dimers. Such formations can hinder the binding efficiency, leading to low yields.
Properly selected primers lay a solid foundation for successful amplification. Even in a well-structured PCR, if the primers are off the mark, you might end up with unexpected results.
Handling and Storing Phusion Polymerase
Phusion Polymerase is a prized asset in your molecular toolkit. To preserve its integrity, correct handling and storage practices are crucial. This enzyme is often temperature-sensitive and can be sensitive to repeated freeze-thaw cycles.
- Storage Conditions: Always keep your Phusion Polymerase at -20°C. If possible, keep it in a dedicated freezer to avoid fluctuations in temperatures. Label your tubes clearly to avoid mix-ups.
- Avoiding Freeze-Thaw Cycles: It's best to aliquot your polymerase into smaller volumes to minimize freeze-thaw cycles. This practice extends the enzyme's shelf-life significantly.
- Working Conditions: When setting up your PCR reactions, ensure that the polymerase is on ice to maintain its stability until you mix it into the reaction. Once the mix is prepared, don't dawdle—start the PCR run promptly.
By treating your enzyme with care, you're setting the stage for optimal activity, paving the way for robust amplification during your PCR runs.
Optimizing PCR Conditions
Every PCR experiment can benefit from a fine-tuning process. Optimizing conditions is analogous to finding the right tempo in a musical piece; too fast or too slow, and something feels off. For Phusion PCR, a few aspects can be adjusted for better results.
- Annealing Temperature: This is a critical parameter. Start high (around the higher end of your primer Tm), and perform a gradient PCR to determine the sweet spot where specificity and yield are maximized.
- Extension Time: With Phusion, you can run up to 30 seconds per kilobase of product. Adjust it depending on the length of your target DNA but accommodate for the enzyme's speed. It's better to start conservatively and adjust as needed.
- Cycle Number: Too many cycles can lead to non-specific products. Generally, 25 to 35 cycles are adequate, but this may vary depending on your starting template.
By meticulously tweaking these conditions, you create an environment conducive to target amplification, which can save countless hours in the lab. These optimizations can lead to cleaner, more reliable results, ensuring that your work makes its mark in the world of research.
"The difference between success and failure in PCR often boils down to the small, meticulous details that can easily be overlooked."
Future Perspectives in PCR Technologies
As the realm of molecular biology continues to evolve, the future perspectives in PCR technologies present exciting opportunities and challenges. Innovations in PCR hold the potential to fundamentally alter how researchers conduct experiments and interpret results. Not only will these advancements enhance the precision and efficiency of DNA amplification, but they will also pave the way for novel applications in genomics, diagnostics, and beyond.
Emerging Trends in High-Fidelity PCR
High-fidelity PCR continues to gain traction as a preferred method for amplification, especially in applications where precision is paramount. This trend is largely driven by the need for more accurate and reproducible DNA sequences. For instance, the introduction of advanced polymerases with improved error rates and processivity has significantly bolstered the reliability of PCR results. Novel high-fidelity enzymes can now amplify long fragments of DNA without compromising the quality of the output. Researchers are finding that these enzymes not only increase yield but also enhance specificity—a key requirement in applications such as cloning and genetic engineering.
Moreover, advancements in the formulation of reaction buffers are optimizing conditions for these high-fidelity enzymes, making it easier to adapt protocols to specific applications. The integration of these aspects creates a landscape where the potential for increased accuracy in sequences translates to more reliable outcomes in downstream applications.
Impact of Automation on PCR Protocols
Automation is reshaping the landscape of PCR—turning what was once a manual, labor-intensive process into a streamlined, reproducible workflow. Automated systems enable high-throughput screening, allowing laboratories to process an increased number of samples with minimal human intervention. This shift not only saves time but also reduces variability introduced by human error. For example, robotic systems can precisely dispense reagents and manage thermal cycling, ensuring consistent results across multiple batches.
The benefits of automation extend beyond efficiency; they also include enhanced safety and reduced risk of contamination. In a fast-paced research environment, the ability to consistently run multiple parallel reactions is invaluable. This potential for scalability means that even smaller labs can compete with larger institutions in genomic studies, thereby democratizing access to advanced PCR technologies.
Integration with Next-Generation Sequencing
As sequencing technologies advance, the integration of PCR with next-generation sequencing (NGS) methodologies has become increasingly prevalent. This synergy allows researchers to not only amplify DNA but also prepare samples for sequencing in a more efficient manner. High-fidelity PCR techniques play a crucial role here, as they ensure that the amplification of DNA fragments meets the quality requirements for sequencing applications.
With the growing demand for high-quality genomic data, the combination of PCR and NGS offers a pathway to improve the accuracy of variant detection and fire up personalized medicine initiatives. The ability to amplify specific regions of interest prior to sequencing is revolutionizing fields like cancer genomics and infectious disease research.
"The future of PCR is bright, with high-fidelity techniques and automation leading the charge toward more reliable results and innovative applications."
In summary, the future perspectives in PCR technologies anchor themselves in the continual pursuit of precision, efficiency, and accessibility. As novel high-fidelity polymerases, automation strategies, and enhanced integrations with NGS emerge, the scope of PCR will undoubtedly expand, fostering breakthroughs in molecular biology research.
Ending
In the realm of molecular biology, the Phusion PCR protocol stands as a benchmark for high-fidelity amplification techniques. The importance of robust methodologies in this field cannot be overstated. This article sheds light on the nuances of the Phusion PCR approach, highlighting the benefits it brings to researchers and practitioners alike.
Summary of Key Points
The Phusion PCR protocol, when meticulously followed, demonstrates several key advantages:
- High Fidelity: Ensures accurate DNA replication with minimal errors, crucial for reliable experimental outcomes.
- Speed: Provides quicker amplification cycles compared to other methods, enhancing lab productivity.
- Versatility: Suitable for a wide range of applications including cloning, sequencing, and mutagenesis.
Moreover, detailed considerations about the components like the polymerase, buffer systems, and primer quality significantly affect the success rates of experiments.
Final Thoughts on Phusion PCR
Phusion PCR serves not only as a formidable tool for DNA amplification but also as a central player in advancing molecular techniques. The protocol's design caters to a variety of research scenarios, showcasing how the right choice of method can lead to groundbreaking discoveries. However, it is imperative for users to remain aware of potential hindrances, such as non-specific amplification or contamination. By addressing these concerns through thorough troubleshooting and best practices, researchers can harness the full potential of Phusion PCR.
As we look toward future developments in PCR technologies, the integration of automation, along with emerging trends in high-fidelity amplification, promises to reshape the molecular biology landscape. Embracing these advancements will undoubtedly elevate the accuracy and efficiency of DNA studies, reinforcing the legacy of the Phusion PCR protocol as a cornerstone of biological research.