Mastering Reverse Primer Design in Molecular Biology
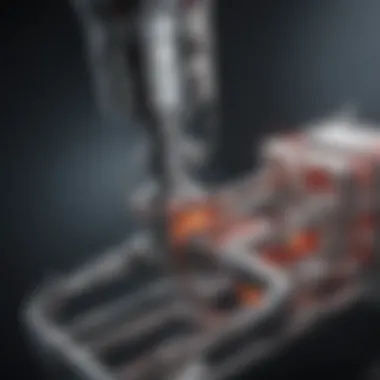
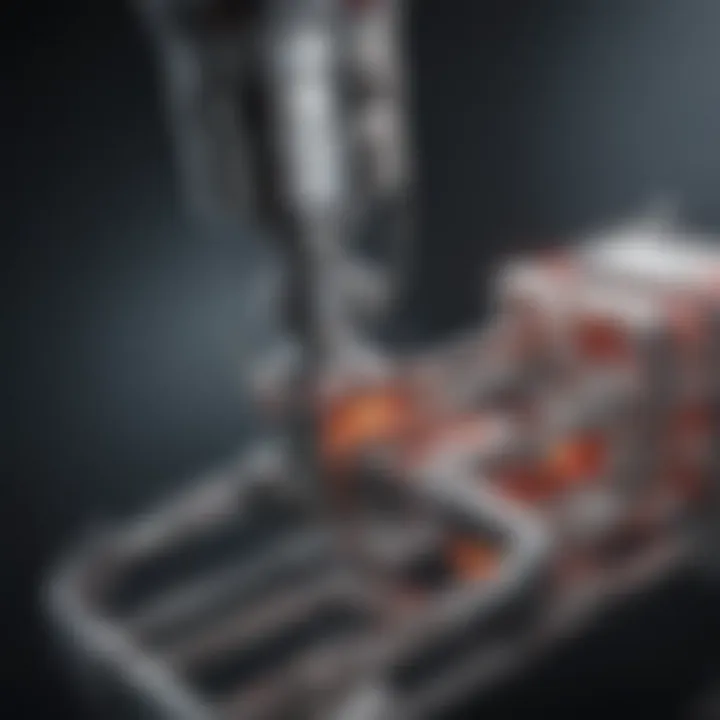
Research Overview
Summary of Key Findings
Reverse primer design is an essential aspect of PCR that plays a critical role in the amplification of DNA. This section will discuss how the design of reverse primers influences the efficiency and specificity of the PCR process. Important findings include the significance of primer length, melting temperature, and GC content. These factors must be optimized to achieve successful amplification.
Background and Context
To better understand reverse primer design, one must appreciate the context of polymerase chain reaction itself. PCR is a technique used to replicate specific DNA sequences, making it invaluable in research, clinical diagnostics, and biotechnology. The selection of primers—forward and reverse—is fundamental to success. Reverse primers, in particular, are designed complementary to the target sequence to ensure accurate and efficient binding during the annealing phase of PCR.
Methodology
Experimental Design
In studies involving reverse primer design, a systematic approach is utilized. Typically, researchers start by identifying the target DNA sequence to amplify. This requires aligning the selection of reverse primers with the desired gene or region of interest. Computational tools often assist in this process, allowing for simulations of binding efficiency and specificity under various conditions.
Data Collection Techniques
Data collection can involve various methods depending on the research goals. Common techniques include:
- Sequencing the amplified products to confirm specificity.
- Quantitative evaluation of PCR products via gel electrophoresis.
- Comparative analysis of primer pairs to determine which combination yields the highest yield and fidelity.
Researchers often emphasize validation through real-time PCR to accurately assess the effectiveness of designed reverse primers. This ensures that the conclusions drawn from the experiments are robust and reproducible.
Reverse primers significantly affect the yield and accuracy of PCR, making careful design essential in molecular experiments.
Prologue to Reverse Primer Design
In the context of molecular biology, reverse primer design holds substantial significance. Understanding this topic is crucial for executing precise experiments involving the Polymerase Chain Reaction (PCR). Primers are short sequences of nucleotides that initiate DNA synthesis during PCR. Properly designed reverse primers enhance the specificity and efficiency of DNA amplification. Given the rapid advancements in genetic research, a steadfast grasp of reverse primer design not only aids in experimental design but also facilitates reproducibility in results.
Definition and Importance
The term "reverse primer" refers to the primer that binds to the complementary strand of DNA in a PCR reaction. This is in contrast to the forward primer, which binds to the target sequence. Essentially, reverse primers are vital for amplifying target regions of DNA, allowing for detailed study and manipulation of genetic material. Without correctly designed reverse primers, PCR could yield non-specific results, leading to a host of challenges during the analysis stage.
The importance of reverse primer design extends beyond basic amplification. It influences the accuracy of genetic studies and diagnostics. As genetic engineering and research become more prevalent, precision in primer design is paramount. A well-conceived reverse primer benefits many areas, including but not limited to gene cloning, sequencing, and pathogen detection.
Role in PCR
PCR relies heavily on primers to dictate the specificity of the amplification process. Reverse primers serve to bind to the complementary DNA strand, aiding the polymerase enzyme in synthesizing the target DNA sequence. The role of reverse primers is twofold: they assist in defining the starting point for copying the DNA and ensure the selection of the correct strand for amplification.
During PCR, the temperature cycles create conditions that favor the annealing of these primers to their respective sequences. An appropriate reverse primer not only ensures high yield but also minimizes the occurrence of undesired products. Thus, choosing an optimal reverse primer is not merely a procedural step; it represents a critical component that defines the entire experimental outcome.
Effective primer design is central to successful PCR applications. Accurate amplification leads to reliable interpretations in various biotechnological applications.
In essence, understanding reverse primer design is foundational for anyone engaging in molecular biology's vast landscape. It establishes a pathway to rigorous experimentation, fostering advancements in the field.
Fundamental Principles of Primer Design
Understanding the fundamental principles of primer design is essential for any molecular biology researcher. This section will discuss key elements such as primer length, melting temperature, and GC content, providing insights into their importance and implications for successful PCR experiments.
Primer Length Considerations
The length of a reverse primer is crucial for its binding efficiency and specificity. Generally, primers range from 18 to 24 nucleotides. Shorter primers may be more prone to non-specific bindings, while longer primers tend to provide more specificity. Each primer should be long enough to ensure a low chance of mismatches with the template DNA but not too long to introduce complexities in the synthesis or annealing processes.
For example, a primer of 20 nucleotides can provide enough unique sequence to target a specific region of interest. However, if the sequence is highly repetitive in the genome, longer primers may enhance specificity. When designing primers, it is also important to consider the potential for secondary structures, which can impact the primer's efficiency. Striking the right balance in length will help achieve the best amplification results.
Melting Temperature (Tm) Calculations
Melting temperature, or Tm, is a significant factor in primer design that affects the annealing process during PCR. Tm refers to the temperature at which half of the DNA strands are in double-helix form and half are in single-strand form. Calculating Tm allows researchers to optimize the PCR conditions by setting appropriate annealing temperatures.
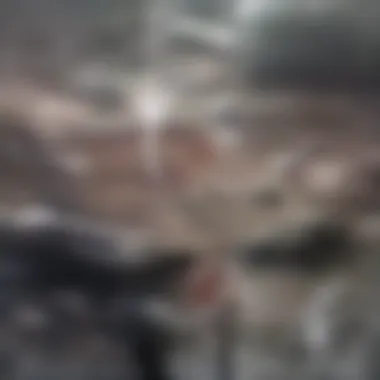
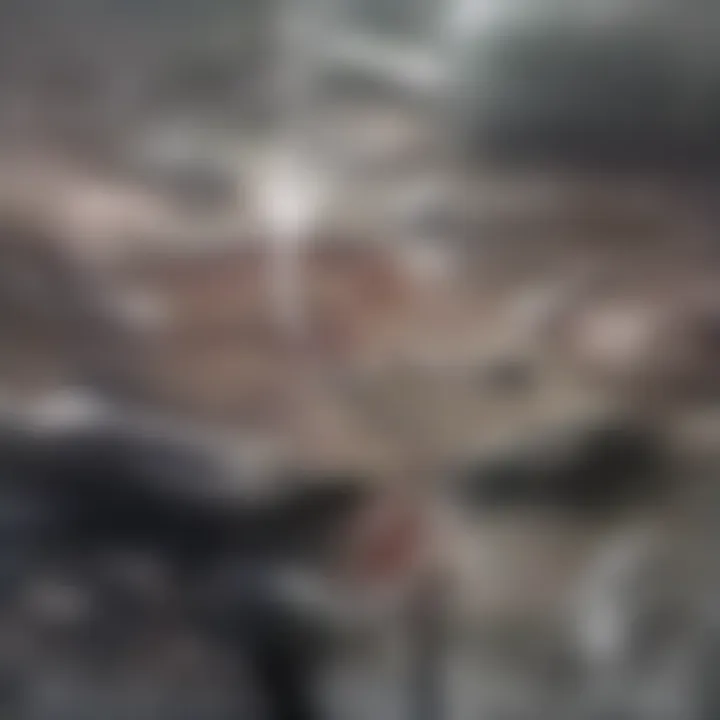
A typical rule of thumb is to aim for a Tm of around 55 to 65 degrees Celsius for primers. Calculating Tm can be done using various formulas, including the widely used formula based on the GC content and the length of the primer:
where A, T, G, and C represent the number of adenine, thymine, guanine, and cytosine nucleotides in the primer. Understanding Tm allows researchers to select appropriate cycling conditions, which can enhance the overall yield and fidelity.
GC Content Variation
The GC content of a primer refers to the proportion of guanine and cytosine bases within it. This content is vital because the GC pairs form three hydrogen bonds, while AT pairs only form two. Thus, primers with higher GC content typically exhibit higher stability and a higher melting temperature.
A recommended range for GC content is 40% to 60%. This variation ensures that the primer binds efficiently to its target without being overly stable, which can hinder amplification. It is essential to account for GC content during the design phase as it influences both the Tm and the occurrence of secondary structures, which can complicate PCR efficiency.
By applying these fundamental principles—considerations of primer length, calculations of melting temperature, and adjustments for GC content—researchers can improve their chances of successful target amplification. Each factor is interconnected and contributes to the overall outcome of PCR experiments.
"A well-designed primer not only enhances specificity but also efficiency during amplification."
Mastering these foundational elements will empower researchers to tackle complex molecular biology challenges effectively.
The Structure of Reverse Primers
The structure of reverse primers holds significant importance in the realm of molecular biology, specifically in techniques like PCR, where accurate amplification is critical. A well-structured reverse primer helps ensure specificity, efficiency, and the overall success of an experiment. By understanding the intricate details of reverse primer structure, researchers can design better primers that yield high-quality results. This section delves into the fundamental components, including nucleotide composition, specificity, binding sites, and potential structural issues like dimerization.
Nucleotide Composition
The nucleotide composition of a reverse primer is vital to its functionality. Typically, primers consist of a blend of adenine (A), cytosine (C), guanine (G), and thymine (T), with the choice of these nucleotides affecting several factors.
A balanced composition fosters effective binding to the target DNA. This requires a consideration of GC content, which influences the melting temperature and stability of the primer. Generally, a GC content of 40-60% is recommended, as it provides optimal binding and reduces the risk of non-specific amplification.
Additionally, the last few nucleotides at the 3’ end of the primer are crucial, as they must be complementary to the template. This allows for efficient extension during PCR.
In summary, nucleotide composition plays a determinative role in primer effectiveness, guiding researchers to create designs that meet their experimental needs.
Specificity and Binding Sites
Specificity is essential in primer design. A reverse primer must anneal selectively to its intended target sequence while avoiding interactions with unintended sequences. This is largely governed by the primer’s length and sequence.
Most reverse primers range from 18 to 25 nucleotides in length, providing a balance between specificity and usability. Primers that are too short may lead to non-specific bindings, while excessively long primers can exhibit reduced efficiency and increased formation of secondary structures.
When choosing sequences, researchers should ensure that the primer does not match any regions in other genomic sequences. One useful approach is to use bioinformatics tools to analyze sequences before experimental design.
Ultimately, specificity and appropriate binding sites ensure that the amplification process targets the desired DNA fragment efficiently, minimizing the likelihood of cross-reactivity with other sequences.
Secondary Structures and Dimerization
Secondary structures can complicate PCR by hindering primer performance. Common issues like hairpins, self-dimers, and cross-dimers can reduce the efficiency of the reaction. These structures arise from intra- or intermolecular base pairing, which should be avoided.
To minimize the formation of secondary structures, it is essential to evaluate the primer’s sequence carefully. Tools are available to predict potential structures based on nucleotide arrangements.
A good practice is to ensure a low probability of dimerization for reverse primers. This can be achieved by selecting sequences with low complementarities. Furthermore, monitoring the stability of the primer under varying conditions can provide insights into potential issues. When designing primers, researchers must always account for these structural considerations, as they directly influence the success of PCR amplification.
"Understanding the structure of reverse primers is not just a technicality; it is a fundamental component of successful molecular experiments."
Factors Influencing Primer Performance
The performance of reverse primers can greatly determine the success of a PCR experiment. Several factors impact their effectiveness, and understanding these elements is crucial for optimizing experimental protocols. Key considerations include the quality of the DNA template, the choice of polymerase, and the optimization of reaction conditions.
Template Quality
The quality of the template DNA is a fundamental factor in primer performance. High-quality template ensures that the primers can bind effectively, leading to optimal amplification. If the template is degraded or poorly purified, it can contain inhibitors that interfere with the binding and extension processes of the PCR.
To improve template quality, it is important to consider the source of DNA, extraction methods, and storage conditions. For example, DNA obtained from fresh tissues generally yields better results than that from older or stored samples. The integrity of the nucleic acids should also be assessed, ideally using gel electrophoresis. Proper quantification of the DNA template is important; too much or too little can disrupt amplification efficiency.
"Quality templates lead to reliable PCR results. Degraded templates result in poor specificity and yield."
Polymerase Choice
The selection of polymerase also plays a significant role in PCR performance. Different polymerases possess unique properties that can either enhance or limit the effectiveness of reverse primers. Some polymerases are designed for high-fidelity amplification, while others may be optimized for speed or yield.
For instance, Taq polymerase is widely used for its robustness in amplifying DNA, but it lacks proofreading ability. In contrast, enzymes like Phusion or Q5 offer high fidelity and efficient amplification for difficult templates. It's important to match the polymerase to the experimental needs. Researchers should also consider the enzyme's reaction conditions, such as optimal temperature and buffer composition, to maximize performance.
Optimization of Reaction Conditions
Optimizing reaction conditions is another critical aspect of ensuring primer performance. Factors such as concentration of primers, template, and magnesium ions can all affect the outcome of a PCR reaction.
- Primer Concentration: Using too much primer can lead to non-specific amplification, while too little may result in weak or no signals. Finding the right balance is crucial for achieving desired results.
- Magnesium Ions: Magnesium is a cofactor for DNA polymerases and influences enzyme activity and stability. Its concentration should be carefully adjusted to enhance specificity and yield.
- Annealing Temperature: This is where the primer binds to the template. If the temperature is too low, non-specific bindings may occur; too high, and the primers may not bind at all.
- Cycle Number: The number of amplification cycles can also impact results. Too many cycles can lead to the amplification of non-specific products.
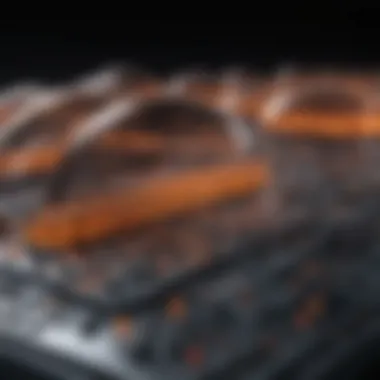
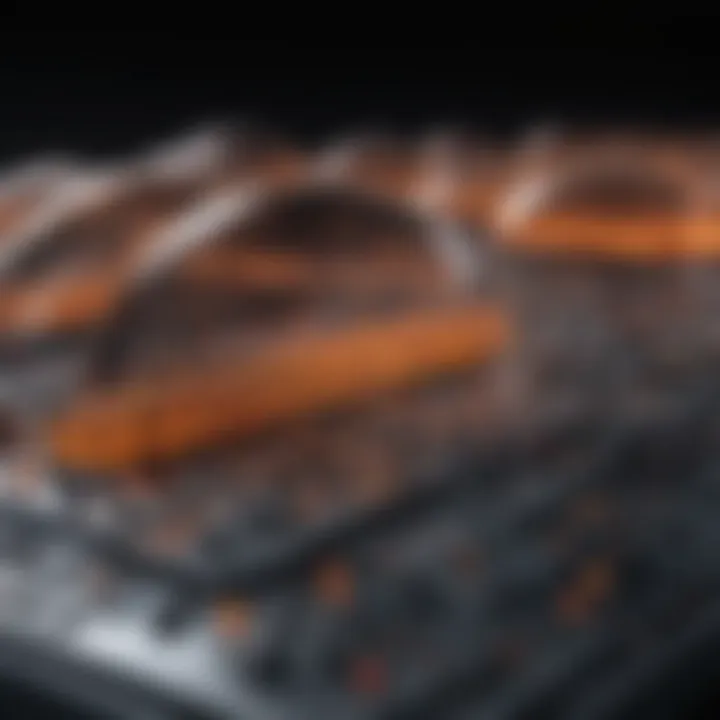
By meticulously adjusting these parameters, researchers can significantly enhance the performance of their reverse primers, leading to more reliable and reproducible results in PCR assays. Engaging in such optimizations is not merely a best practice but a necessity for achieving meaningful scientific outcomes.
Bioinformatics Tools for Primer Design
Bioinformatics tools have significantly enhanced the precision and effectiveness of reverse primer design in molecular biology. These software applications streamline the primer design process, allowing researchers to predict the behavior, specificity, and efficiency of primers before actual experiments. By employing these tools, scientists can avoid common pitfalls, reduce costs, and expedite their research processes. Furthermore, bioinformatics approaches open doors to advanced analyses, thus expanding possibilities in genomic studies. Understanding various available software options and in-silico techniques is essential for optimizing primer design and subsequent experiments.
Overview of Available Software
A variety of software tools exist for primer design, each offering unique features and functionalities. Some notable examples include:
- Primer3: This is one of the most popular tools used for designing PCR primers. Primer3 allows users to specify particular criteria, such as primer length, GC content, and melting temperature, enabling tailored design suitable for specific applications.
- Beacon Designer: Designed mainly for real-time PCR experiments, this software focuses on designing primers along with fluorescent probes. It helps in optimizing the efficiency of quantitative PCR controls.
- OligoCalc: This online tool provides a straightforward interface for calculating various properties of oligonucleotides. It is particularly useful for quick checks on primer properties, such as Tm and concentration.
- Integrated DNA Technologies (IDT): IDT offers a web-based primer design tool that incorporates advanced features such as customizations specific for high-throughput applications.
These tools provide essential support for primer design, helping researchers select optimal primers for their experiments effectively.
In-Silico Analysis Techniques
In-silico analysis techniques play a crucial role in primer design. These techniques aid in evaluating the designed primers for possible mispairing, secondary structures, and off-target amplification. Here are some common approaches:
- Homology Searches: This technique involves comparing the designed primer sequences against existing databases or genomic sequences to identify potential binding sites. Tools such as BLAST (Basic Local Alignment Search Tool) are frequently used for this purpose.
- Secondary Structure Prediction: Computing the likelihood of secondary structures forming within the primer or target region can prevent issues during amplification. Software such as Mfold assists researchers in predicting these structures to ensure successful PCR reactions.
- Binding Affinity Calculations: Assessing the binding energy of the primer-template interactions contributes to understanding specificity. Calculating the Gibbs free energy change can help in predicting if the primer will bind correctly to the target.
These in-silico analysis techniques provide a comprehensive pre-experimental assessment, thus increasing the chance of success in achieving desired amplification outcomes.
By integrating bioinformatics tools into reverse primer design, researchers can ensure a more strategic approach, minimizing trial and error while enhancing the quality of experimental results.
Experimental Validation of Primers
Experimental validation is a pivotal phase in the primer design process. It seeks to confirm that the designed reverse primers function effectively in a real-world setting, beyond the theoretical calculations and predictions available in computational tools. The vitality of this process cannot be underestimated, as successful PCR results depend heavily on the primers' capacity to bind specifically to the target DNA sequence. Proper validation ensures reproducibility and reliability in experimental outcomes, which is essential in the field of molecular biology.
The process involves several steps to assess primer efficiency and specificity. It can provide critical insights into how well a primer can amplify the target sequence. If validation is done correctly, it adds a layer of confidence that the primers will yield consistent results across different reactions.
Testing Primer Efficiency
To evaluate the efficiency of reverse primers, a common method is to perform a standard curve analysis. This involves amplifying a known quantity of template DNA and measuring the resulting fluorescence values at each cycle. The efficiency can be calculated from the slope of the standard curve. An ideal efficiency falls in the range of 90% to 110%, indicating optimal performance.
The evaluation process is not limited to quantitative PCR, though it is perhaps the most common. For qualitative assessments, gel electrophoresis can be employed to visualize the amplified products. Specific bands can confirm that both the forward and reverse primers function as intended, amplifying the target without generating nonspecific products.
In addition to standard curves, the use of controls is essential during testing. Including a positive control with a well-characterized template ensures that any observed amplification is indeed due to the primer activity. Negative controls, on the other hand, help identify contamination or primer-dimer formations, which can compromise experimental integrity.
Troubleshooting Common Issues
Despite diligent design and careful testing, various issues can arise during the validation of reverse primers. Recognizing these challenges is critical for ensuring accurate experimental outcomes.
Common Issues Include:
- Non-specific amplification: This occurs when primers bind to off-target regions. It can lead to multiple bands on a gel, indicating the presence of undesired products. To troubleshoot, consider adjusting the annealing temperature or redesigning the primers to increase specificity.
- Primer-dimer formation: This happens when primers hybridize with each other instead of the target DNA. This can significantly inhibit the efficiency of the PCR. Reducing primer concentrations or using tools to predict dimer formation during the design phase may help mitigate this issue.
- Inconsistent results: Variability in results may arise from issues with template quality or reaction conditions. Ensuring the template is free from contaminants and using consistent cycling parameters can aid in yielding reproducible data.
Through careful and systematic testing and troubleshooting, the validation of reverse primers establishes a solid foundation for consistent and accurate DNA amplification. This ultimately supports significant advancements in research and applications in molecular biology.
Case Studies in Reverse Primer Design
The investigation of reverse primer design in real-world applications adds substantial depth to our understanding of its role in molecular biology. By analyzing specific case studies, we can discern what approaches lead to successful outcomes and where missteps may occur. The clear benefits include practical insights into both the execution and pitfalls of primer design. Researchers can extract useful lessons that refine their own techniques, leading towards more reliable results.
Successful Applications
Successful applications of reverse primers often underpin significant breakthroughs in molecular biology. One notable example is the development of reverse primers for detecting RNA viruses. Primers designed for reverse transcription PCR have proven essential in diagnosing infections like HIV and influenza. These primers target specific regions of viral RNA, facilitating its amplification. This application highlights the crucial balance between specificity and efficiency during the design phase should not be overlooked.
In another interesting case, reverse primers have been employed in genetic engineering. Researchers desiring to insert or modify genes use reverse primers to amplify specific DNA segments. The incorporation of this PCR step has allowed for precise editing through techniques like CRISPR. This demonstrates that not only is reverse primer design vital in diagnostics, but it also plays a role in modern biotechnological applications.
Lessons from Failures
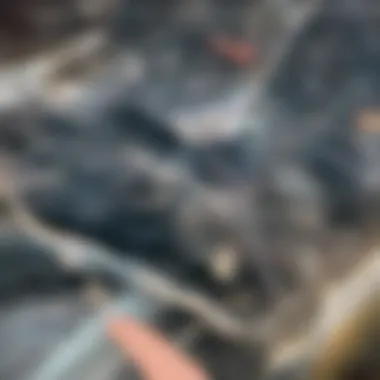
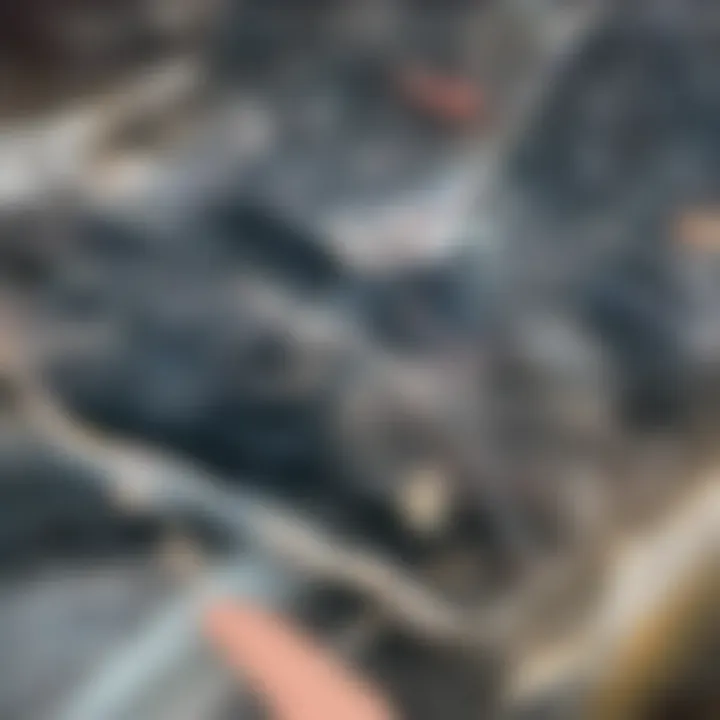
Despite myriad successes, there are numerous instances where poor reverse primer design has led to profound failures. A common issue arises with non-specific binding, where primers attach to unintended sequences. Such incidents lead to false positives in experimental results. For instance, a study aimed at amplifying a specific gene found that poorly designed reverse primers amplified a non-target region, complicating analysis and resulting in unreliable data.
Additionally, various researchers have faced challenges due to secondary structures. In some cases, the primers formed dimers resulting in greatly reduced amplification efficiency. This issue emphasizes the need for thorough testing of the designed primers before heading into actual experiments. Primers should be validated in-silico and through initial PCR trials, ensuring they function as intended.
Understanding successes and failures in reverse primer design is essential. It aids in the refinement of approaches, allowing for improved experimental outcomes.
By examining both successful applications and learned lessons from failures, we can foster a deeper understanding of the significance of reverse primer design in molecular biology. Each case study offers unique insights, contributing to the ongoing evolution of primer design strategies.
Ethical and Practical Considerations
In the field of molecular biology, ethical and practical considerations are paramount. They ensure that research is conducted responsibly, fostering trust in scientific advancements. Understanding these elements is essential for both new entrants and seasoned researchers in reverse primer design. The implications of primer design extend beyond the laboratory; they influence public perception and regulatory frameworks.
Regulatory Guidelines
Regulatory guidelines provide a framework for responsible research practices. These guidelines help ensure that primer design aligns with both national and international standards. Researchers must stay abreast of the regulations established by organizations such as the National Institutes of Health (NIH) and the World Health Organization (WHO). These regulations cover various aspects, including the ethical treatment of samples, data accuracy, and transparency in reporting results.
Adhering to these regulations can prevent misconduct and enhances the reliability of results. Furthermore, researchers must seek the necessary approvals before commencing experimental work. This approach not only protects human subjects involved in studies but also ensures that the research outcomes contribute positively to the scientific community.
Impact on Research Integrity
Research integrity is a cornerstone of scientific inquiry. Primer design impacts the reliability of results and ultimately affects the credibility of scientific findings. If primers are designed without consideration for reproducibility, there exists a risk of generating false or misleading data.
Research misconduct can arise from various sources, such as selective reporting or failure to disclose potential conflicts of interest. Therefore, researchers should strive for transparency in their methods and results. By openly sharing their primer design protocols and outcomes, researchers contribute to a culture of integrity and accountability in science.
"Scientific integrity is not a luxury; it is a necessity for the advancement of knowledge."
Maintaining high standards in reverse primer design not only serves to advance science but also protects public trust in research. This trust is crucial for continued funding and support of scientific endeavors, making it more important than ever to align ethical considerations with practical practices.
Future Trends in Primer Design
The landscape of molecular biology is continually evolving, with reverse primer design at the forefront of innovation. Understanding future trends in this field is essential for researchers aiming to enhance the precision and efficiency of PCR applications. As technology progresses, there are critical elements to consider.
Primarily, advancements in analytical techniques are reshaping how primers are designed and evaluated. These techniques allow for better prediction of primer behavior, enhancing the efficiency of DNA amplification. Additionally, the integration with next-generation sequencing (NGS) techniques is revolutionizing primer application, enabling more nuanced and comprehensive analyses.
Advancements in Analytical Techniques
Modern analytical methods are crucial for refining reverse primer design. High-throughput approaches and computational modeling now provide unprecedented insights. For instance, machine learning algorithms can predict the performance of primers based on large datasets, improving the accuracy of design choices.
These advancements not only facilitate faster primer testing but also enhance the predictability of outcomes. Tools like Primer3 or OligoCalc are becoming standard, allowing users to input various parameters and quickly receive optimized primer designs. Furthermore, the increasing accuracy of in-silico simulations means researchers can confidently select primers that are likely to perform well in experiments.
Some key points regarding these advancements include:
- Greater efficiency in identifying optimal primer sequences.
- Minimized trial-and-error approaches leading to reduced costs and time.
- Enhanced specificity by utilizing sophisticated algorithms which account for secondary structures.
"In-silico predictions are transforming the traditional methods of primer design by reducing the need for extensive empirical testing."
Integration with Next-Generation Sequencing
The integration of reverse primers with next-generation sequencing technologies is a significant trend impacting how genomic studies are conducted. NGS allows researchers to sequence multiple fragments of DNA simultaneously, providing a comprehensive view of genetic information. Here, reverse primers play an essential role in targeting specific regions for amplification before sequencing.
This synergy facilitates:
- Increased throughput, allowing for more extensive genetic analyses.
- Enhanced sensitivity in detecting low-abundance sequences that were previously difficult to analyze effectively.
- Ability to combine multiple primers in multiplex assays, broadening the scope of studies that can be simultaneously performed.
The direct implications of this integration include better insights into genetic diversity, improved disease diagnostics, and the ability to perform detailed studies on heterogeneous samples.
End
Summary of Key Points
- Definition and Importance: Reverse primers are crucial for specific DNA amplification in PCR.
- Role in PCR: They ensure accurate targeting of complementary DNA strands, enhancing amplification efficiency.
- Primer Length and Tm: Appropriate primer length and melting temperature (Tm) are vital for optimal binding conditions and amplification efficiency.
- Specificity of Binding Sites: Ensuring specificity minimizes non-specific amplification and increases assay reliability.
- Factors Influencing Performance: Template quality, polymerase selection, and reaction conditions are critical for successful experiments.
- Bioinformatics Tools: Software aids in the design process, improving efficiency and accuracy.
- Experimental Validation: Testing for efficiency and troubleshooting enhance the overall quality of the research.
- Ethical Considerations: Awareness of regulatory guidelines and maintaining research integrity are crucial aspects of primer design.
The Ongoing Importance of Primer Design
The significance of primer design does not stop at basic understanding; it evolves with advancements in technology and methodologies. As PCR techniques continue to improve, sophisticated requirements emerge. The introduction of next-generation sequencing implies that primer design strategies must adapt accordingly to meet the complex needs of modern genomic research. Moreover, continuous exploration of bioinformatics tools enhances the potential for innovative designs, further emphasizing the critical need for ongoing education and adaptation in this area.
Overall, primer design remains a cornerstone of molecular biology. A strong grasp of these concepts ensures robust research outputs that can influence findings across various scientific domains. For both novices and seasoned researchers, continuous learning in this arena stands as a testament to the evolving nature of biological sciences and the importance of precision in research.